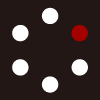
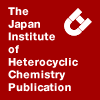
HETEROCYCLES
An International Journal for Reviews and Communications in Heterocyclic ChemistryWeb Edition ISSN: 1881-0942
Published online by The Japan Institute of Heterocyclic Chemistry
e-Journal
Full Text HTML
Received, 20th May, 2014, Accepted, 2nd June, 2014, Published online, 4th June, 2014.
DOI: 10.3987/COM-14-S(K)31
■ Three-Way Output Molecular Response System Based on Tetrakis(3,4-dialkoxyphenyl)-3,4-dihydro[5]helicenes: Perturbation of Properties by Long Alkyl Chains
Yusuke Ishigaki, Satoshige Yoshida, Hidetoshi Kawai, Ryo Katoono, Kenshu Fujiwara, Takanori Fukushima, and Takanori Suzuki*
Division of Chemistry, Graduate School of Science, Hokkaido University, Kita 10 Nishi 8, Kita-ku, Sapporo, Hokkaido 060-0810, Japan
Abstract
Starting from (S)-dimethyl 1,1'-binaphthyl-2,2'-dicarboxylate, optically pure (P)-3,3,4,4-tetrakis(3,4-dialkoxyphenyl)-3,4-dihydro[5]helicenes (P)-1 were prepared via bis(diarylhydroxymethyl)binaphthyl and/or tetraaryldinaphthodihydroxepin as key intermediates. Upon electrochemical oxidation to the corresponding (S)-1,1'-binaphthyl-2,2'-diylbis(diarylcarbenium)s (S)-22+, three kinds of spectral changes (UV-Vis, circular dichroism, fluorescence) were successfully observed. Compared to the methoxy compound, the octyloxy and hexadecyloxy derivatives exhibit a redshifted emission maxima in the dihydro[5]helicenes. The long alkyl chains induce step-by-step two-electron reduction of the binaphthyldiyl(dicarbenium)s whereas the dication with methoxy groups exhibits one-wave two-electron process.Recently much attention has been focused on the molecular response systems, in which the physical/spectral properties of the molecule can be reversibly modified by the external stimuli. The electrochemical input is transduced into UV-Vis spectral output by the electrochromic compounds,1 for which the organic redox systems are promising candidates. Other spectra, such as circular dichroism (CD) or fluorescence (FL), would be modified in addition to UV-Vis under eligible molecular design. Such transducers can serve as the less-explored multi-output response systems,2,3 which are attracting in pursuing the error-free "molecular logic gate", since the logical output can be verified by multiple independent signals.
We previously studied a series of electron-donating 3,3,4,4-tetrakis(4-alkoxyphenyl)-3,4-dihydro[5]helicenes 3, which were interconvertible with 1,1'-binaphthyl-2,2'-diylbis[bis(4-alkoxyphenyl)-carbenium]] dyes 42+.2 Beside vivid change in color, their 2e-redox processes were accompanied by C-C bond formation/cleavage ("dynamic redox" behavior)4 as in the case of 9,9,10,10-tetraaryl-9,10-dihydrophenanthrenes 5 and biphenyl-2,2'-diylbis(diarylcarbenium)s 62+ (Scheme 1). The "dyrex" behavior endows the pairs with electrochemical bistability, which is one of the advantageous properties for their use in developing advanced functionalized materials such as unimolecular memories.4
The largest difference between the pairs of 3/42+ and 5/62+ is configurational stability in helicity/axial chirality in 3/42+ since dibenzo annulation on 5/62+ suppresses the ring-flip/rotation around the biaryl axis, respectively. In fact, (P)/(M)-3 were proven to be interconvertible with (R)/(S)-42+ without loss of enantiopurity.2 Furthermore, dibenzo analogue 3 is fluorescent due to the rigid 3,4-dihydro[5]helicene skeleton whereas 9,10-dihydrophenanthrenes 5 are non-fluorescent. In this way, the optically active pairs of 3/42+ serve as three-way output electrochromic systems (Scheme 2).
In the course of our further studies for pursuing the way to modify the redox/spectroscopic properties of multi-output response systems, we got interested in examining the effects of long alkyl chains on the electron donating aryl groups in the redox pairs. The intermolecular interaction of long alkyl chains has been known as "molecular fastener effects",5 which cause more packed arrangement of molecules in crystals. In polar solvents, the hydrophobic effects often induce close proximity of long alkyl chains. Thus, the molecular response systems with long alkyl chains would have a chance to exhibit different redox/spectroscopic properties compared to those having no long chains.
Here we designed the title 3,4-dihydro[5]helicenes (1a-c) with four 3,4-dialkoxyphenyl groups as the new entry into the multi-output electrochromic systems, which would exhibit three kinds of spectral changes of UV-Vis, CD, and FL upon 2e-oxidation to the corresponding binaphthylic dications 2a-c2+. Although no apparent chain-length dependency was observed in the properties of 3a-c/4a-c2+ with only one alkoxy group on each aryl group,2 we found here that eight octyloxy substituents in 1b/2b2+ and eight hexadecyloxy substituents in 1c/2c2+ could modify their properties, so that significant changes were observed from those of methoxy-substituted redox pair 1a/2a2+, as shown below (Scheme 3).
Following the previous synthetic strategy, new 3,4-dihydro[5]helicene derivatives 1a-c were planned to prepare according to Scheme 4. The final step is reductive cyclization of dicationic dyes 2a-c2+, which in turn would be generated from 5,7-dihydro-5,5,7,7-tetrakis(3,4-dialkoxyphenyl)dinaphtho[c,e]oxepins 8a-c or 2,2'-bis[bis(3,4-dialkoxyphenyl)hydroxymethyl]binaphthyls 7a-c, under acidic dehydrating conditions. They would be obtained from dimethyl 1,1'-binaphthyl-2,2'-dicarboxylate 92,6 by four-time addition of 3,4-dialkoxyphenyllithiums generated from the corresponding bromides 10a-c.
To a THF-hexane solution of bromide 10b (6.5 eq) with two octyl chains was added BuLi in hexane (6 eq) at –78 °C under Ar. After 1 h, optically pure (R)-9 was added at –78 °C. Then, the mixture was stirred at room temperature for 15 h. An aqueous work-up (extraction with CH2Cl2) afforded diol (R)-7b in 94% yield. Another run with a slightly different work-up (extraction with AcOEt) afforded oxepin (M)-8b in 92% yield, showing that the diol 7 can easily undergo intramolecular cyclization to seven-membered cyclic ether 8.
Trimethylsilyl perchlorate (TMSClO4) in 1,1,1,3,3,3-hexafluoro-2-propanol (HFIP) is the reagent of choice (Ichikawa's method)7 for transforming 7/8 into dication 22+ since the weaker acidic conditions could not convert oxepin 8 to 22+ whereas the stronger acid such as HBF4 caused dealkylation of the alkoxy substituents on aryl groups. Thus, upon treatment of a HFIP solution of diol (R)-7b with TMSClO4/toluene (4 eq) at 25 °C for 21 h, deep purple color characteristic to the dicationic dye was developed. After evaporation of solvent, the residue containing (R)-2b2+(ClO4)2 was directly reduced by Zn dust (4 eq) in THF at 25 °C for 5 h to give (M)-1b as colorless oil in 87% yield after SiO2-chromatography. Under the very similarly conditions, oxepin (M)-8b was converted to (M)-1b in 84% yield, showing that oxepin 8 would be involved as the intermediate during the conversion of diol 7 to dication 22+.
By starting with the antipode of chiral ester [(S)-9] and bromide 10a-c, diol intermediates (S)-7a-c were obtained in respective yields of 64, 71, and 73% yield, where lithiation of 10c with hexadecyloxy substituents smoothly proceeded in a different composition of solvent (ether-hexane, 3 : 1) at room temperature.8 The Ichikawa's method7 (TMSClO4/HFIP) again worked satisfactorily on 7a,c irrespective of the chain length. Thus, diols (S)-7a-c were converted to (P)-1a-c via oxepins (P)-8a-c and dication salts (S)-2a-c2+(ClO4)2 in respective yields of 76, 86, and 76% yield after Zn reduction.9 No signs of racemization were detected during the chemical transformation.
Newly prepared 3,4-dihydro[5]helicenes are colorless crystals [(P)-1a,c] or colorless oil [(P)-1b] with strong Cotton effects in the UV-region [λext/nm (Δε) in CH2Cl2: 268 (+148) for (P)-1a; 269 (+165) for (P)-1b; 269 (+164) for (P)-1c]. Furthermore, they all emit violet fluorescence (vide infra) characteristic to the 3,4-dihydro[5]helicene skeleton.10 Thus, upon electrochemical oxidation of (P)-1a, continuous changes in three kinds of spectra (UV-Vis, CD, and FL) were observed, which corresponds to clean conversion to (S)-2a2+ (Scheme 2).11 Reversal of the spectral changes were observed by switching the polarity of the electrodes. Similar three-way-output electrochromic behavior was observed when electrolyses were conducted on (P)-1b,c. When the enantiomer was used [(M)-1b], UV-Vis and FL responses were identical and the mirror image of CD spectral change was observed (Figure 1). This is another successful example for stereospecific transformation of optically pure biaryls into helicene derivatives.12
Presence of several isosbestic points in the CD spectral change indicates negligible steady-state concentration of the open-shell intermediates (Scheme 5), and only two species (e.g. 1 and 22+) were predominantly present during the electrolyses as in the cases of electrochemical interconversion of "dyrex" pairs of 3/42+ and 5/62+.2,4 Thus, during the oxidation process, 1●+ generated upon one-electron oxidation of 1 easily undergoes C-C bond fission to give 2●+, which is more easily oxidized to 22+ than 1. In the reduction process of 22+, reduction potential of 22+ to 2●+ and that for 2●+ to 22● are nearly the same, so that 2●+ is short-lived.
The significant difference in the spectral changes of "dyrex" pairs 1a-c/2a-c2+ was found in the FL spectra. Although the intensity of FL monotonically decreased upon electrolyses in all cases, the emission maxima of the starting 3,4-dihydro[5]helicenes 1a-c is redshifted with the increase in length of the alkyl chains [λem/nm in CH2Cl2 (λex 346 nm): 415 for 1a; 421 for 1b; 428 for 1c]. The spectrum of hexadecyl derivative also has a shoulder at 448 nm (Figure 2a). Such a chain-length dependency of fluorescence spectrum was never observed in 3,4-dihydro[5]helicenes (3a-c) with four 4-alkoxypheneyl groups, thus the increase in the number of alkoxy groups made the effects more prominently to be observed in this study. At this moment, the reason for the redshift is unclear but one of the possibilities is the excimer-type association.
Another characteristic modulation of properties by the long alkyl chains was observed in the cyclic voltammetric analyses (Figure 3). Methoxy derivative 1a underwent 2e-oxidation at +1.26 V vs SCE in CH2Cl2, which was the irreversible process due to C-C bond cleavage. The return peak appeared in the far cathodic region (Ered +0.21 V), which corresponds to the 2e-reduction process of bond-dissociated dication 2a2+. Such a large shift of redox peaks is characteristic to the "dyrex" pairs including 3/42+ and 5/62+.4 In contrast, in the return cycle of the voltammogram of hexadecyl derivative 1c (Eox +1.26 V), two reduction peaks were observed, each of which corresponds to the reduction of 2c2+ to 2c●+ (Ered +0.17 V) and that of 2●+ to 22● (Ered –0.01 V), respectively.
Separation of two reduction peaks was observed upon electrochemical reduction of the dications having two electronically different cationic chromophores13 whereas it seldom happened for the dication with two units of the same chromophores as in tetrakis(4-alkoxyphenyl)-type dications 4a-c2+. In the case of octyloxy derivative 1b/2b2+, a single return peak of 2b2+ was observed with the scan rate of 0.1 V s-1 as in the methoxy derivative 2a2+, whereas the two separated peaks similar to 2c2+ were observed by increasing the scan rate up to 1 V s-1 as in the hexadecyl derivative 2c2+. Thus, the long alkyl chains retard the disproportionation of 2b,c●+ into 2b,c2+ and 2b,c2● during the 2e-reduction processes.
In conclusion, we found here that eight octyloxy substituents in 1b/2b2+ and eight hexadecyloxy substituents in 1c/2c2+ modify their properties. Thus, FL spectra of 1b,c is redshifted than that of methoxy-substituted derivative 1a, and the discrete two-step reduction occurred in 2b,c2+ whereas 2a2+ underwent one-wave 2e-reduction. Both differences were observed in CH2Cl2 solutions of medium polarity, suggesting that long alkyl chains actually make significant effects but neither by means of hydrophobic effects working in polar media nor by "molecular fastener effects" working in crystalline state. We are currently continuing the studies to clarify the origin of the effects and to elaborate the observed novel long-alkyl chain effects to be used as a new modifying method for molecular response systems.
ACKNOWLEDGEMENTS
This work was supported by Grant-in-Aid for Scientific Research on Innovative Areas: "Organic Synthesis Based on Reaction Integration" (No. 2105) from MEXT, Japan. T.S. thanks JSPS Grant-in-Aid for Challenging Exploratory Research on "Maximum Function on Minimum Skeleton (MFMS)" (No. 25620050). Y.I. thanks JSPS research fellowship for young scientists (22-648). This work was also supported by the Cooperative Research Program of “Network Joint Research Center for Materials and Devices”.
References
1. P. M. S. Monk, R. J. Mortimer, and D. R. Rosseinsky, 'Electrochromism and Electrochromic Devices', Cambridge Univ. Press, Cambridge, 2007. CrossRef
2. T. Suzuki, Y. Ishigaki, T. Iwai, H. Kawai, K. Fujiwara, H. Ikeda, Y. Kano, and K. Mizuno, Chem. Eur. J., 2009, 15, 9434. CrossRef
3. H. Higuchi, K. Ichioka, H. Kawai, K. Fujiwara, M. Ohkita, T. Tsuji, and T. Suzuki, Tetrahedron Lett., 2004, 45, 3027; CrossRef T. Suzuki, S. Tanaka, H. Kawai, and K. Fujiwara, Chem. Asian J., 2007, 2, 171; CrossRef T. Suzuki, K. Ohta, T. Nehira, H. Higuchi, E. Ohta, H. Kawai, and K. Fujiwara,, Tetrahedron Lett., 2008, 49, 772; CrossRef T. Suzuki, K. Wada, Y. Ishigaki, Y. Yoshimoto, E. Ohta, H. Kawai, and K. Fujiwara, Chem. Commun., 2010, 46, 4100; CrossRef T. Suzuki, Y. Kuroda, K. Wada, Y. Sakano, R. Katoono, K. Fujiwara, F. Kakiuchi, and T. Fukushima, Chem. Lett., 2014, 43, 887.. CrossRef
4. T. Suzuki, E. Ohta, H. Kawai, K. Fujiwara, and T. Fukushima, Synlett, 2007, 851; CrossRef T. Suzuki, H. Higuchi, T. Tsuji, J. Nishida, Y. Yamashita, and T. Miyashi, Chemistry of Nanomolecular Systems. Chapter 1: Dynamic Redox Systems, Springer, Heidelberg, 2003, pp. 3–24.
5. H. Inokuchi, G. Saito, P. Wu, K. Seki, T. B. Tang. T. Mori, K. Imaeda, T. Enoki, Y. Higuchi, K. Inaka, and N. Yasuoka, Chem. Lett., 1986, 1263; CrossRef S. Shinamura, I. Osaka, E. Miyazaki, and K. Takimiya, Heterocycles, 2011, 83, 1187; CrossRef S. Ukai, S. Igarashi, M. Nakajima, K. Marumoto, H. Ito, S. Kuroda, K. Nishimura, Y. Enomoto, and G. Saito, Colloids Surf. A: Physicochem. Eng. Aspects, 2006, 284-285, 589. CrossRef
6. T. Ohta, M. Ito, K. Inagqaki, and H. Takaya, Tetrahedron Lett., 1993, 34, 1615; CrossRef H. Hocke and Y. Uozumi, Tetrahedron, 2003, 59, 619. CrossRef
7. T. Saitoh, S. Yoshida, and J. Ichikawa, Org. Lett., 2004, 6, 4563; CrossRef T. Saitoh, S. Yoshida, and J. Ichikawa, J. Org. Chem., 2006, 72, 6414. CrossRef
8. Typical procedure: To a solution of 1-bromo-3,4-dihexadecyloxybenzene 10c (1.98 g, 3.10 mmol) in 30 mL of dry Et2O and 10 mL of dry hexane was added BuLi (1.65 M solution in hexane, 1.9 mL, 3.10 mmol) dropwise over 5 min at 23 °C under Ar, and the mixture was stirred for 1 h. To the suspension was added ester (S)-9 (183 mg, 495 μmol) at 23 °C, and stirred for 18 h. After diluted with water, the whole mixture was extracted with hexane. The combined organic layers were washed with water and brine, and dried over MgSO4. After filtration, solvent was concentrated under reduced pressure. The residue was purified by column chromatography on silica gel (hexane/CH2Cl2 = 1.5) to give diol (S)-7c (916 mg) as a pale yellow solid in 73% yield. Data of (S)-7c: mp 43 °C; 1H NMR (300 MHz, CDCl3): δ/ppm 7.74 (d, J = 8.8 Hz, 2H), 7.66 (d, J = 7.9 Hz, 2H), 7.33-7.26 (m, 4H), 7.12 (dd, J = 7.9, 7.9 Hz, 2H), 6.89 (s, 2H), 6.75 (s, 2H), 6.52-6.40 (m, 8H), 6.13 (d, J = 8.8 Hz, 2H), 4.03 (s, 2H), 3.96 (t, J = 6.6 Hz, 4H), 3.87 (t, J = 6.6 Hz, 4H), 3.76 (t, J = 6.6 Hz, 4H), 3.65-3.54 (m, 2H), 3.45-3.40 (m, 2H), 1.82-0.82 (m, 248H); IR (KBr): ν/cm-1 3541, 3405, 2919, 2849, 1603, 1509, 1468, 1417, 1379, 1260, 1233, 1135, 1065, 1028, 811, 787, 744, 721; LR-MS (FD): m/z (%) 2544 (35), 2543 (70), 2542 (94), 2541 (bp), 2540 (M+, 52); [α]D24 +21.1 (c 1.05, CHCl3).
Diols (S)-7a,b were obtainedby the similar procedure except using CH2Cl2 as an extracting solvent in the work-up process. In situ generation of Ar-Li from 10a,b was conducted in THF-hexane. The spectral data are as follows: (S)-7a: mp 130-131 °C; 1H NMR (300 MHz, CDCl3): δ/ppm 7.79 (d, J = 8.8 Hz, 2H), 7.71 (d, J = 8.1 Hz, 2H), 7.33 (d, J = 8.8 Hz, 2H), 7.19 (dd, J = 8.8, 7.1 Hz, 2H), 6.98 (s, 2H), 6.77 (m, 4H), 6.58-6.44 (m, 8H), 6.18 (d, J = 8.4 Hz, 2H), 3.98 (s, 2H), 3.87 (s, 6H), 3.79 (s, 6H), 3.66 (s, 6H), 3.44 (s, 6H); IR (KBr): ν/cm-1 3416, 2997, 2933, 2833, 1603, 1590, 1510, 1464, 1410, 1258, 1237, 1137, 1028, 869, 808, 787, 764; LR-MS (FD): m/z (%) 860 (21), 859 (59), 858 (M+, bp), 840 (28); [α]D23 -156 (c 0.44, CHCl3). (S)-7b: 1H NMR (300 MHz, CDCl3): δ/ppm 7.74 (d, J = 8.8 Hz, 2H), 7.66 (d, J = 7.9 Hz, 2H), 7.31 (d, J = 8.8 Hz, 2H), 7.12 (dd, J = 8.8, 7.9 Hz, 2H), 6.89 (s, 2H), 6.75 (s, 4H), 6.52-6.40 (m, 8H), 6.13 (d, J = 8.8 Hz, 2H), 4.03 (s, 2H), 3.96 (t, J = 6.6 Hz, 4H), 3.87 (t, J = 6.6 Hz, 4H), 3.77 (t, J = 6.6 Hz, 4H), 3.57 (t, J = 9.2 Hz, 2H), 3.44 (t, J = 9.2 Hz, 2H), 1.82-0.82 (m, 120H); IR (neat): ν/cm-1 3541, 3388, 3053, 2923, 2855, 1603, 1511, 1503, 1469, 1416, 1379, 1257, 1135, 1028, 867, 810, 786, 743, 723; LR-MS (FD): m/z (%) 1646 (27), 1645 (63), 1644 (bp), 1643 (M+, 84); [α]D23 -47.4 (c 0.38, CHCl3).
9. To a solution of diol (S)-7c (916 mg, 360 μmol) in 6 mL of HFIP was added a solution of TMSClO4 in toluene (790 mM, 1.95 mL, 1.54 mmol) at 23 °C under Ar, which was prepared from TMSCl (1.0 mL, 7.92 mmol) and AgClO4 (1.65 mg, 7.96 mmol) in dry toluene (10 mL). The mixture was stirred for 21 h, and the solvent was evaporated. To the residue was added Zn powder (980 mg, 14.99 mmol) and dry THF (30 mL). The mixture was stirred at 23 °C for 5 h and diluted with water. The whole mixture was extracted with hexane. The combined organic layers were washed with water and brine, and dried over anhydrous MgSO4. After filtration, solvent was concentrated under reduced pressure. The residue was purified by column chromatography on silica gel (hexane/toluene = 4/3) to give (P)-1c (100 mg) as a colorless solid in 76% yield. Data of (P)-1c: mp 46 °C; 1HNMR (300 MHz, CDCl3, 50 °C): δ/ppm 7.75 (d, J = 8.4 Hz, 2H), 7.63 (d, J = 8.8 Hz, 2H), 7.42 (d, J = 8.8 Hz, 2H), 7.35 (dd, J = 8.4, 7.1 Hz, 2H), 7.25-7.23 (m, 2H), 7.08 (dd, 8.8, 7.1 Hz, 2H), 6.94 (s, 2H), 6.78 (d, J =8.4 Hz, 2H), 6.56 (d, J = 8.4 Hz, 2H), 6.29 (s, 2H), 6.22 (d, J = 8.8 Hz, 4H), 3.95 (t, J = 6.6 Hz, 4H), 3.68 (t, J = 6.6 Hz, 4H), 3.60-3.42 (br, 4H), 2.83-2.65 (m, 4H), 1.82-0.82 (m, 248H); IR (KBr): ν/cm-1 2919, 2850, 1602, 1511, 1504, 1489, 1468, 1411, 1379, 1257, 1235, 1144, 1017, 785, 766, 721; LR-MS (FD): m/z (%) 2511 (18), 2510 (34), 2509 (63), 2508 (92), 2507 (bp), 2506 (M+, 67), 2505 (28), 2504 (15); [α]D24 +105 (c 0.72, CHCl3); UV-Vis (CH2Cl2): λmax/nm (ε) 362 (9410), 337 (11200).
3,4-Dihydro[5]helicenes (P)-1a,b were obtained by the similar procedure except using CH2Cl2 as an extracting solvent in the work-up process. The spectral data are as follows: (P)-1a: mp 145 °C; 1H NMR (300 MHz, CDCl3, 50 °C): δ/ppm 7.78 (d, J = 8.4 Hz, 2H), 7.68 (d, J = 8.8 Hz, 2H), 7.44 (d, J = 8.8 Hz, 2H), 7.39 (dd, J = 8.4 Hz, 1.3 Hz, 2H), 7.28-7.22 (m, 2H), 7.12 (ddd, 8.8, 7.1, 1.3 Hz, 2H), 6.98 (s, 2H), 6.89 (dd, J = 8.4 Hz, 1.3 Hz, 2H), 6.64 (d, J = 8.4 Hz, 2H), 6.44 (d, J = 8.8 Hz, 2H), 6.27 (d, J = 8.8 Hz, 2H), 6.18 (s, 2H), 3.86 (s, 6H), 3.62 (s, 6H), 3.43 (s, 6H), 2.84 (s, 6H) ; IR (KBr): ν/cm-1 3057, 2996, 2951, 2932, 2905, 2832, 2832, 1603, 1582, 1514, 1464, 1443, 1406, 1315, 1258, 1238, 1181, 1148, 1129, 1028, 809, 787, 759; LR-MS (FD): m/z (%) 826 (19), 825 (60), 824 (M+, bp); [α]D24 +224 (c 0.15, CHCl3); UV/Vis (CH2Cl2): λmax/nm (ε) 369 (6350), 346 (8200). (P)-1b: 1H NMR (300 MHz, CDCl3, 50 °C): δ/ppm 7.75 (d, J = 8.4 Hz, 2H), 7.64 (d, J = 8.8 Hz, 2H), 7.43 (d, J = 8.8 Hz, 2H), 7.35 (dd, J = 8.4, 7.1, 2H), 7.25-7.23 (m, 2H), 7.09 (dd, 8.8, 7.1 Hz, 2H), 6.94 (s, 2H), 6.79 (d, J = 8.4 Hz, 2H), 6.57 (d, J = 8.8 Hz, 2H), 6.29 (s, 2H), 6.23-6.21 (m, 4H), 3.95 (t, J = 6.6 Hz, 4H), 3.69 (t, J = 6.6 Hz, 4H), 3.60-3.42 (br, 4H), 2.82-2.65 (m, 4H), 1.82-0.82 (m, 120H); IR (neat): ν/cm-1 3054, 2926, 2855, 2746, 1602, 1514, 1503, 1469, 1454, 1409, 1379, 1255, 1145, 1027, 904, 865, 798, 768, 745, 723; LR-MS (FD): m/z (%) 1613 (11), 1612 (29), 1611 (64), 1610 (bp), 1609 (M+, 85); [α]D24 +159 (c 0.69, CHCl3); UV/Vis (CH2Cl2): λmax/nm (ε) 362 (8240), 346 (9490).
10. E. Ohta, T. Nehira, H. Kawai, K. Fujiwara, and T. Suzuki, Tetrahedron Lett., 2008, 49, 777; CrossRef P. H. Schippers and H. P. J. M. Dekkers, Tetrahedron, 1982, 38, 2089. CrossRef
11. Upon treatment of 2a with 2eq of (4-BrC6H4)3N●+SbCl6–, dication salt 2a2+(SbCl6–)2 was obtained as a black powder in 93% yield, which regenerated 2a in quantitative yield upon treatment with Zn in THF.
12. H. J. Bestmann and W. Both, Angew. Chem., Int. Ed. Engl., 1972, 11, 296; CrossRef K. Tanaka, H. Suzuki, and H. Osuga, J. Org. Chem., 1997, 62, 4465; CrossRef J. Nishida, T. Suzuki, M. Ohkita, and T. Tsuji, Angew. Chem. Int. Ed., 2001, 40, 3251; CrossRef H. Higuchi, E. Ohta, H. Kawai, K. Fujiwara, T. Tsuji, and T. Suzuki, J. Org. Chem., 2003, 68, 6605; CrossRef E. Ohta, H. Higuchi, H. Kawai, K. Fujiwara, and T. Suzuki, Org. Biomol. Chem., 2005, 3, 3024. CrossRef
13. Y. Ishigaki, T. Suzuki, J. Nishida, A. Nagaki, N. Takabayashi, H. Kawai, K. Fujiwara, and J. Yoshida, Materials, 2011, 4, 1906; CrossRef T. Suzuki, J. Nishida, and T. Tsuji, Chem. Commun., 1998, 2193. CrossRef
14. The cation radicals 2●+ would adopt the conformation in which the diarylmethylium and diarylmethyl groups are stacked in parallel with a partial face-to-face overlap. Such a geometry is favorable for charge-transfer (CT) interaction between the chromophores. The CT interaction stabilizing the cation radical state might be enhanced when the media surrounding those chromophores is less polar. One of the possibilities to account for the stepwise reduction of 2b,c2+ is that the multiple long alkyl chains in 2b,c●+ modify the local polarity around the chromophores to gain higher stability of 2b,c●+ than in 2a●+.