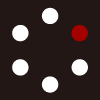
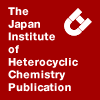
HETEROCYCLES
An International Journal for Reviews and Communications in Heterocyclic ChemistryWeb Edition ISSN: 1881-0942
Published online by The Japan Institute of Heterocyclic Chemistry
e-Journal
Full Text HTML
Received, 15th February, 2014, Accepted, 27th February, 2014, Published online, 4th March, 2014.
DOI: 10.3987/COM-14-S(K)7
■ Cobalt-Catalyzed C5-Selective C-H Functionalization of 4-Me-Quinolines with Styrenes: An Approach to 5,6-Dihydro-4H-benzo[de]quinolines
Shohei Yamamoto, Shigeki Matsunaga,* and Motomu Kanai*
Graduate School of Pharmaceutical Sciences, The University of Tokyo, 7-3-1 Hongo, Bunkyo-ku, Tokyo 113-0033, Japan
Abstract
Cobalt-catalyzed C5-selective C-H functionalization of 4-Me-quinolines is described. A postulated cobalt-hydride catalyst, generated from 2 mol % of CoI2, promoted the reaction of 4-Me-quinolines with styrenes, giving 5,6-dihydro-4H-benzo[de]quinolines (34-55% yield) in one-pot. A plausible reaction mechanism is also described.Nitrogen-containing heteroarenes are important structural cores found in many biologically active natural products, drug candidates, and clinically applied drugs.1 In medicinal chemistry research, modifications of heteroarenes are often necessary to optimize the properties of drug candidates. For example, in lead optimization studies of the pentacyclic anti-cancer camptothecin, a quinoline core was intensively modified (Figure 1).2 Hexacyclic camptothecin analogs bearing the 5,6-dihydro-4H-benzo[de]quinoline core had high topoisomerase I inhibitory activity,2a,2b and exatecan (DX8951f) mesylate is now clinically used as a water-soluble camptothecin analog.2c Previous methods for synthesizing the 5,6-dihydro-4H-benzo[de]quinoline core, however, often required multi-step processes including quinoline construction.2,3 Thus, studies are needed for the development of a concise straightforward approach starting from readily available quinolines.
Transition metal-catalyzed regioselective direct C-H bond functionalization of heteroarenes has attracted attention because it provides rapid access to various functionalized heteroarenes.4 In many reports, a Lewis basic sp2 nitrogen atom in the heteroarenes is used as a directing group to achieve C2-selective C-H bond functionalization of pyridines and quinolines.5 In contrast, methods for selectively functionalizing other positions are rare. Recently, several groups reported either C3-,6 C4-,7 or C8-selective8 catalytic C-H functionalization of pyridines and quinolines. As a part of our ongoing studies of first-row transition metal-catalyzed C-H functionalization,9-12 we also succeeded in C4-selective alkylation of pyridines10a and quinolines.10b Our approach, utilizing a postulated cobalt-hydride species generated from cobalt amide, is summarized in Scheme 1. Mechanistic studies suggested that the reaction proceeds via hydrometalation/C4-selective nucleophilic addition/re-aromatization,13,14 giving C4-alkylated pyridines and quinolines with no stoichiometric oxidant. Here we describe further application of our cobalt catalysis for C5-selective C-H functionalization of 4-Me-quinolines with styrenes, giving 5,6-dihydro-4H-benzo[de]quinolines in one-pot.
As a part of the mechanistic studies of C4-selective alkylation of quinolines, we investigated the reaction with 4-Me-quinoline, expecting that alkylation would proceed at the C2-position. Unexpectedly, however, the reaction of 4-Me-quinoline with styrene under the optimized conditions for C4-alkylkation of quinolines [2 mol % of Co(OAc)2, 0.4 equiv of BuLi, 1.4 equiv of pyridine, Scheme 2]10b gave C5-functionalized 5,6-dihydro-4H-benzo[de]quinoline 4aa in 36% yield (determined by 1H NMR with an internal standard), while C2-alkylated product 3 was not obtained. Products 5aa with a 5,6,7,8-tetrahydro-4H-cycloocta[de]quinoline core, derived from 4-Me-quinoline and two equivalents of styrene, were also obtained in 4% yield as inseparable regio- and diastereo-mixtures. Because there are no reports of transition metal-catalyzed C5-selective C-H functionalization of quinolines,15 we further optimized the reaction conditions to improve the yield of C5-functionalized products (Table 1). Among the reaction parameters,16 metal sources significantly affected the yield of the desired C5-functionalized products, and Co(OAc)2 produced a much better yield than the other metal acetates (entry 1 vs entries 2-6). The cobalt salt counter ion was also important (entries 7-10), and CoI2 was the best, giving 4aa in 61% NMR yield together with 22% of 5aa (entry 10). Finally, the reaction at 70 °C with CoI2 was selected as the best conditions, giving 62% of 4aa (55% isolated yield) and 26% of 5aa (entry 11). Although any explanation is too speculative at the moment, we assume that the active species of the present reaction with excess hydride sources (0.4 equiv) over cobalt salts (2 mol %) would be a cobalate species, which could explain why the cobalt salt counter ion impacted the reactivity.
The preliminary substrate scope of the reaction under the optimized conditions is shown in Table 2. The reaction of 4-Me-quinoline 1a with various styrene derivatives 2a-2e proceeded to give the desired products 4aa-4ae in 35-55% yield (entries 1-5). The scope of quinolines, however, was narrow, and subtle changes in the substituent on the quinoline ring prevented the desired reaction. 4,7-Me2-quinoline 1b gave 4ba in 34% yield (entry 6), while a substituent at the C6-position significantly retarded the desired C5-functionalization (entries 7-8). Further trials to improve the reactivity and broaden the scope of quinolines by modifying the cobalt catalyst are ongoing.
The hypothetical catalytic cycle of the reaction is shown in Figure 2. Based on previous reports on the reactivity of 4-Me-pyridines,17 we speculate that a relatively acidic benzylic position would be deprotonated with a basic cobalt-hydride species (or excess lithium amide generated from pyridine and BuLi) to give a Co-amide intermediate I. Addition of I to styrene 2 would afford a key organo-cobalt intermediate II. Because the C5-position of the quinoline unit is electrophilic, intramolecular nucleophilic addition of the organo-cobalt species18 onto the quinoline ring would proceed to give III by analogy with our previous reports on C4-alkylation of pyridines10a and quinolines.10b We assume that the C5-selective addition to the quinoline ring was difficult with C6-substituted substrates due to steric hindrance (Table 2, entries 7-8). Re-aromatization of the quinoline ring via cobalt-hydride elimination would afford 4 and regenerate the cobalt-hydride species. Studies to gain insight into the reaction mechanism are ongoing in our group.
In summary, we developed a cobalt-catalyzed C5-selective C-H functionalization of 4-Me-quinolines. A postulated cobalt-hydride catalyst, generated from 2 mol % of CoI2, promoted the reaction of 4-Me-quinolines with styrenes, giving 5,6-dihydro-4H-benzo[de]quinolines in 34-55% yield. Although the scope of 4-Me-quinolines is narrow, the present method provides a new straightforward approach to construct a useful 5,6-dihydro-4H-benzo[de]quinoline core.
ACKNOWLEDGEMENTS
This work was supported in part by ACT-C from JST, Grant-in-aid for Scientific Research on Innovative Areas “Molecular Activation Directed toward Straightforward Synthesis” from MEXT.
References
1. Reviews: (a) J. A. Joule and K. Mills, in Heterocyclic Chemistry, 4th ed., Blackwell Publishing, Oxford, 2000, pp. 63-120; (b) G. D. Henry, Tetrahedron, 2004, 60, 6043; CrossRef (c) M. Schlosser and F. Mongin, Chem. Soc. Rev., 2007, 36, 1161; CrossRef (d) M. D. Hill, Chem. Eur. J., 2010, 16, 12052; CrossRef (e) J. A. Bull, J. J. Mousseau, G. Pelletier, and A. B. Charette, Chem. Rev., 2012, 112, 2642. CrossRef
2. (a) M. Sugimori, A. Ejima, S. Ohsuki, K. Uoto, I. Mitsui, K. Matsumoto, Y. Kawato, M. Yasuoka, K. Sato, H. Tagawa, and H. Terasawa, J. Med. Chem., 1994, 37, 3033; CrossRef (b) M. Sugimori, A. Ejima, S. Ohsuki, K. Uoto, I. Mitsui, Y. Kawato, Y. Hirota, K. Sato, and H. Terasawa, J. Med. Chem., 1998, 41, 2308; CrossRef (c) I. Mitsui, E. Kumazawa, Y. Hirota, M. Aonuma, M. Sugimori, S. Ohsuki, K. Uoto, A. Ejima, H. Terasawa, and K. Sato, Jpn. J. Cancer Res., 1995, 86, 776. CrossRef
3. For selected other methods for synthesizing the 5,6-dihydro-4H-benzo[de]quinoline core, see: (a) F. C. Uhle, C. G. Vernick, and G. L. Schmir, J. Am. Chem. Soc., 1955, 77, 3334; CrossRef (b) E. Sobarzo-Sánchez, B. K. Cassels, and L. Castedo, Synlett, 2003, 1647; CrossRef (c) J.-U. Peters, T. Capuano, S. Weber, S. Kritter, and M. Sägesser, Tetrahedron Lett., 2008, 49, 4029; CrossRef (d) A. A. Khalaf, A. M. El-Khawaga, I. M. Awad, and H. A. K. Abd El-Aal, ARKIVOC, 2010, (x), 338; CrossRef (e) Y. Lian, J. R. Hummel, R. G. Bergman, and J. A. Ellman, J. Am. Chem. Soc., 2013, 135, 12548. CrossRef
4. Review on C-H functionalization of pyridines and quinolines, Y. Nakao, Synthesis, 2011, 3209. CrossRef
5. For selected examples of C2-selective catalytic direct alkylation of pyridines and quinolines without prior activation, see: (a) R. F. Jordan and D. F. Taylor, J. Am. Chem. Soc., 1989, 111, 778; CrossRef (b) J. C. Lewis, R. G. Bergman, and J. A. Ellman, J. Am. Chem. Soc., 2007, 129, 5332; CrossRef (c) L. D. Tran and O. Daugulis, Org. Lett., 2010, 12, 4277; CrossRef (d) B.-T. Guan and Z. Hou, J. Am. Chem. Soc., 2011, 133, 18086 and references therein. CrossRef
6. C3-Selective catalytic C-C bond formation of pyridines, see: (a) M. Ye, G.-L. Gao, A. J. F. Edmunds, P. A. Worthington, J. A. Morris, and J.-Q. Yu, J. Am. Chem. Soc., 2011, 133, 19090; CrossRef (b) M. Ye, G.-L. Gao, and J.-Q. Yu, J. Am. Chem. Soc., 2011, 133, 6964; CrossRef (c) B.-J. Li and Z.-J. Shi, Chem. Sci., 2011, 2, 488. CrossRef
7. C4-Selective catalytic C-C bond formation of pyridines and quinolines, see: (a) Y. Nakao, Y. Yamada, N. Kashihara, and T. Hiyama, J. Am. Chem. Soc., 2010, 132, 13666; CrossRef (b) C.-C. Tsai, W.-C. Shih, C.-H. Fang, C.-Y. Li, T.-G. Ong, and G. P. A. Yap, J. Am. Chem. Soc., 2010, 132, 11887; CrossRef For C4-Selective catalytic silaboration/rearomatization of pyridines, see also: (c) K. Oshima, T. Ohmura, and M. Suginome, J. Am. Chem. Soc., 2011, 133, 7324. CrossRef
8. C8-Selective catalytic arylation of quinolines, J. Kwak, M. Kim, and S. Chang, J. Am. Chem. Soc., 2011, 133, 3780. CrossRef
9. Review on the first-row transition metal-catalyzed C-H bond activation/C-C bond formation, (a) A. A. Kulkarni and O. Daugulis, Synthesis, 2009, 4087; CrossRef (b) N. Yoshikai, Synlett, 2011, 1047. CrossRef
10. (a) T. Andou, Y. Saga, H. Komai, S. Matsunaga, and M. Kanai, Angew. Chem. Int. Ed., 2013, 52, 3213; CrossRef (b) S. Yamamoto, Y. Saga, T. Andou, S. Matsunaga, and M. Kanai, Adv. Synth. Catal., 2014, 356, 401. CrossRef
11. For other cobalt-catalyzed C-H functionalization from our group, see: (a) T. Yoshino, H. Ikemoto, S. Matsunaga, and M. Kanai, Angew. Chem. Int. Ed., 2013, 52, 2207; CrossRef (b) T. Yoshino, H. Ikemoto, S. Matsunaga, and M. Kanai, Chem. Eur. J., 2013, 19, 9142; CrossRef (c) B. Sun, T. Yoshino, S. Matsunaga, and M. Kanai, Adv. Synth. Catal., 2014, 356, 1491. CrossRef
12. Low-valent cobalt catalysts have been intensively investigated for C-H functionalization reactions. For leading examples, see: (a) K. Gao, P.-S. Lee, T. Fujita, and N. Yoshikai, J. Am. Chem. Soc., 2010, 132, 12249; CrossRef (b) K. Gao and N. Yoshikai, J. Am. Chem. Soc., 2011, 133, 400; CrossRef (c) Q. Chen, L. Ilies, and E. Nakamura, J. Am. Chem. Soc., 2011, 133, 428; CrossRef (d) B. Li, Z.-H. Wu, Y.-F. Gu, C.-L. Sun, B.-Q. Wang, and Z.-J. Shi, Angew. Chem. Int. Ed., 2011, 50, 1109; CrossRef (e) Z. Ding and N. Yoshikai, Angew. Chem. Int. Ed., 2012, 51, 4698; CrossRef (f) W. Song and L. Ackermann, Angew. Chem. Int. Ed., 2012, 51, 8251. CrossRef
13. C2-Selective catalytic arylation of pyridines and quinolines via nucleophilic addition/rearomatization strategy, see: (a) M. Tobisu, I. Hyodo, and N. Chatani, J. Am. Chem. Soc., 2009, 131, 12070; CrossRef A related work on acridine arylation and alkylation: (b) I. Hyodo, M. Tobisu, and N. Chatani, Chem. Commun., 2012, 48, 308. CrossRef
14. C4-selective nucleophilic addition of organometallic reagents to pyridines and quinolines followed by treatment with stoichiometric amount of oxidants: Q. Chen, X. Mollat du Jourdin, and P. Knochel, J. Am. Chem. Soc., 2013, 135, 4958. CrossRef
15. Non-selective direct C5-perfluoroalkylation of quinolines with perfluoroalkyl radicals, see: (a) W.-Y. Huang, J.-T. Liu, and J. Li, J. Fluorine Chem., 1995, 71, 51; CrossRef (b) X.-T. Huang, Z.-Y. Long, and Q.-Y. Chen, J. Fluorine Chem., 2001, 111, 107. CrossRef
16. Other reaction parameters, such as solvent, ligand, additive, concentration, the amount of styrene, and others, did not improve the yield of desired adduct 4aa.
17. Benzylic C-H functionalization of 4-Me-pyridines, (a) M. Rueping and N. Tolstoluzhsky, Org. Lett., 2011, 13, 1095; CrossRef (b) H. Komai, T. Yoshino, S. Matsunaga, and M, Kanai, Synthesis, 2012, 44, 2185; CrossRef For selected related works on benzylic C-H functionalization with 2-Me-azaarenes under metal-catalysis, see: (c) B. Qian, S. Guo, J. Shao, Q. Zhu, L. Yang, C. Xia, and H. Huang, J. Am. Chem. Soc., 2010, 132, 3650; CrossRef (d) B. Qian, S. Guo, C. Xia, and H. Huang, Adv. Synth. Catal., 2010, 352, 3195; CrossRef (e) H. Komai, T. Yoshino, S. Matsunaga, and M. Kanai, Org. Lett., 2011, 13, 1706. CrossRef
18. Other possibility that a radical generated by homolytic cleavage of the cobalt-carbon bond in II reacts with the quinoline ring cannot be excluded at the present stage.