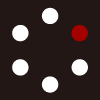
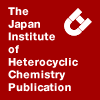
HETEROCYCLES
An International Journal for Reviews and Communications in Heterocyclic ChemistryWeb Edition ISSN: 1881-0942
Published online by The Japan Institute of Heterocyclic Chemistry
e-Journal
Full Text HTML
Received, 13th January, 2014, Accepted, 24th January, 2014, Published online, 30th January, 2014.
DOI: 10.3987/COM-14-12941
■ Enantioselective Intramolecular Aza-Spiroannulation onto Benzofurans Using Chiral Rhodium Catalysis
Takuro Shibuta, Shigeki Sato, Masatoshi Shibuya, Naoki Kanoh, Tohru Taniguchi, Kenji Monde, and Yoshiharu Iwabuchi*
Graduate School of Pharmaceutical Sciences, Tohoku University, 6-3 Aobayama, Sendai 980-8578, Japan
Abstract
The development of efficient and enantioselective intramolecular aza-spiroannulation onto benzofurans using chiral rhodium catalysis is described. The optimized reaction conditions [Rh2(S-TCPTAD)4 (3 mol %), PhIO (1.6 equiv), MeOH (10 equiv) in PhCF3, 0 °C] brought about oxidative aza-spiroannulation of 3-(carbamoylmethyl)benzofuran (3) resulting in (2R,3S)-2-methoxy-2H-spiro- [benzofuran-3,4'-oxazolidin]-2'-one (15a) in 69% yield with 86% ee, the absolute structure of which was determined by a combination of X-ray crystallography and vibrational circular dichroism (VCD) spectroscopy. The reaction is applicable to the asymmetric construction of various 2,3-dihydrobenzofuran derivatives bearing a nitrogen-containing tetrasubstituted carbon stereocenter at C3 (up to 92% ee).2-Oxindoles and 2,3-dihydrobenzofurans, furnished with a nitrogen-containing tetrasubstituted chiral center at the C3 positions,1,2 are structural motifs found in a variety of potential medicines, pharmaceutically relevant and/or structurally intriguing natural products (Figure 1),3-8 thus attracting considerable attention from the synthetic community.
Despite its structural similarity to 3-amino-2-oxindole, for which several synthesis strategies have been developed,3-5,9 few reliable methods capable of constructing 3-amino-2,3-dihydrobenzofurans in an enantiocontrolled manner have been reported to date.6b,6c,7,10 In the light of successful applications of Rh(II)-catalyzed intramolecular nitrene transfer11-13 onto indole substrates (Scheme 1),14-16 an analogous aza-spiroannulation onto benzofuran substrates was envisaged, due to the recent discovery of (–)-fumimycin (1)6a as a novel class of antibiotic agents based on its inhibition of peptide deformylase activity.17 Notwithstanding the rather discouraging precedents of aza-spiroannulation onto benzofuran
(Scheme 1, a),15a,18 the modular nature of the chiral rhodium-15c,16 or iron17-nitrene complex encouraged us to identify useful conditions for installing the intended nitrogen-containing tetrasubstituted carbon at C3 with high enantioselectivity (Scheme 1, b and c). Herein, we describe the first diastereo- and enantiocontrolled aza-spiroannulation onto benzofurans using chiral rhodium catalysis.
The investigation commenced with the inspection of the reactivity and enantioselectivity of simple benzofuran 3 under typical reaction conditions, using the Rh2(S-TCPTTL)4(cat.)19/PhI(OAc)2/MgO system.12 As anticipated, the desired spirocycle 920 was only obtained in 39% yield as a diastereomeric mixture (dr = 1.2:1), which is in line with the result of Padwa et al15b (Scheme 2).
The enantioselectivity was also disappointingly low (20% ee). Furthermore, the undesired compound 1020 was produced in 30% yield. On the basis of these results, it was speculated that this particular rhodium-catalyzed reaction of the benzofuran 3 proceeded in the following manner (Scheme 2), namely: (i) the initial generation of the rhodium nitrenoid 11 is followed by an intramolecular aziridination to yield aziridine 12; (ii) 12 can equilibrate with betaine 13, and this equilibrated species can be isomerized to 14 under given conditions; (iii) AcOH, generated in situ, attacks 12 at C2 from the convex face to yield the α–acetoxy anomer of 9; (iv) the nucleophilic attack of AcOH with betaine 13 proceeds in a less diastereoselective manner to produce a diastereomeric mixture of 9, while the nucleophilic attack of betaine 14 by AcOH yields the undesired by-product 10. The desired spirocyclic compounds were predicted to form efficiently and in a stereospecific manner when aziridine intermediate 12 is quickly attacked by stronger nucleophiles prior to its isomerization to betaine 13. The choice of alcohol as a nucleophile seemed to be suitable for this purpose.21,22 As expected, the treatment of carbamate 8 with Rh2(S-TCPTTL)4 (3 mol %), 1.6 equiv of PhIO, 50 equiv of MeOH, and PhCF3 as the solvent afforded the spirocycle 15a in 71% yield as a single diastereomer (Table 1, entry 1). However, the enantioselectivity of 15a was still low, that is, 36% ee. As the desired spirocycle was successfully obtained, the focus shifted to the improvement in enantioselectivity (Table 1). When the amount of MeOH was decreased to 10 equiv, a slightly higher level of ee was observed (entry 2). The use of 3 mol % Rh2(S-TCPTAD)423 as a chiral catalyst provided 15a in 68% ee (entry 3). Screening of nucleophiles was then undertaken to examine their influence on enantioselectivity using EtOH, allyl alcohol,
and AcOH (entries 4–6). As a result, spirocycles 15b and 15c were respectively obtained as a single diastereomer though they exhibited a lower ee than 15a (entries 4 and 5 vs entry 3). When 10 equiv of AcOH was added, the result got worse: spirocycle 9 resulted as a diastereomeric mixture with quite a low enantioselectivity along with undesired compound 10 (entry 6). Ultimately, it was elucidated that lowering the reaction temperature is critical for good enantioselectivity. When this aza-spirocyclization reaction using MeOH was carried out at 0 °C, spirocycle (–)-15a was obtained in good yield with good enantioselectivity characterized by 69% yield and 86% ee (entry 7).
The absolute stereostructure of the product (–)-15a was determined by a combination of X-ray crystallography and vibrational circular dichroism (VCD) spectroscopy.24,25 The relative configuration of 15a was established as shown in Table 1 by X-ray crystallographic study (Figure 2, a). To determine the absolute configuration of (–)-15a, calculations for conformational analysis were carried out using arbitrarily selected (2R,3S)-15a (Figure 2, b). Theoretical IR and VCD calculations were then performed for each conformer using density functional theory (DFT) with the B3LYP/6-311+G(d,p) level of theory, and the final calculated IR and VCD spectra were obtained based on the Boltzmann population average of each spectrum. The population-weighted theoretical VCD spectra were shown to be in good agreement
with the experimental VCD spectra (Figure 2, c). As the result, the absolute configuration of (–)-15a was elucidated to be (2R,3S). The determined absolute stereostructure of (–)-15a indicates that spirocycle 15a was generated through an SN2-type ring opening of the aziridine intermediate 12.
The scope of the asymmetric aza-spiroannulation conditions optimized for the benzofuran 8 was investigated by applying them to a diverse range of benzofurans (Figure 3). The introduction of oxygen functional groups and a phenyl group at C5 was tolerated and the corresponding spirocycles were obtained in good yield with reasonable enantioselectivity (73−80% ee) (16a–16d).26 The halogen-substituted benzofurans were found to be suitable substrates in this particular aza-spirocyclization reaction. The spirocyclic compounds 16e–16h showed good level of ee (84–92% ee). Although bromo-substituted 16h was obtained in low yield, unreacted benzofuran substrate was recovered without severe decomposition. Spirocycle 16i, in which an allyl group was introduced at C4 directed for fumimycin (1) synthesis, was obtained in good yield but with moderate enantioselectivity, implying that modification of the aromatic moiety should be conducted after construction of the C3 stereocenter.
In summary, an efficient asymmetric aza-spiroannulation onto benzofurans was developed based on chiral rhodium catalysis. This procedure provides a reliable platform for the construction of enantio-enriched 3-amino-2,3-dihydrobenzofurans in particular non-substituted and mono-substituted ones. A concise synthesis of the core structure of fumimycin has also been achieved. Efforts toward total synthesis of (–)-fumimycin based on this methodology are now ongoing.27
ACKNOWLEDGEMENTS
This work was partly supported by a Grant-in-Aid for Scientific Research on Innovative Areas “Advanced Molecular Transformations by Organocatalysis” from the Ministry of Education, Culture, Sports, Science and Technology, Japan.
References
1. K. Shen, X. Liu, L. Lin, and X. Feng, Chem. Sci., 2012, 3, 327. CrossRef
2. T. D. Sheppard, J. Chem. Res., 2011, 7, 377. CrossRef
3. For selected synthesis of AG-041R, see (a) T. Emura, T. Esaki, K. Tachibana, and M. Shimizu, J. Org. Chem., 2006, 71, 8559; CrossRef ; CrossRef (c) S. Mouri, Z. Chen, S. Matsunaga, and M. Shibasaki, Heterocycles, 2012, 84, 879; CrossRef (d) N. Hara, S. Nakamura, M. Sano, R. Tamura, Y. Funahashi, and N. Shibata, Chem. Eur. J., 2012, 18, 9276. CrossRef
4. M. Rottmann, C. McNamara, B. K. S. Yeung, M. C. S. Lee, B. Zou, B. Russell, P. Seitz, D. M. Plouffe, N. V. Dharia, J. Tan, S. B. Cohen, K. R. Spencer, G. E. González-Páez, S. B. Lakshminarayana, A. Goh, R. Suwanarusk, T. Jegla, E. K. Schmitt, H.-P. Beck, R. Brun, F. Nosten, L. Renia, V. Dartois, T. H. Keller, D. A. Fidock, E. A. Winzeler, and T. T. Diagana, Science, 2010, 329, 1175. CrossRef
5. H. Takayama, I. Mori, M. Kitajima, N. Aimi, and N. H. Lajis, Org. Lett., 2004, 6, 2945. CrossRef
6. For isolation and biological activity of (–)-fumimycin, see (a) Y.-J. Kwon, M.-J. Sohn, C.-J. Zheng, and W.-G. Kim, Org. Lett., 2007, 9, 2449; CrossRef For the total synthesis; (b) P. J. Gross and S. Bräse, Chem. Eur. J., 2010, 16, 12660; CrossRef (c) P. J. Gross, F. Furche, M. Nieger, and S. Bräse, Chem. Commun., 2010, 46, 9215. CrossRef
7. For isolation of (–)-phalarine, see (a) N. Anderton, P. A. Cockrum, S. M. Colegate, J. A. Edgar, K. Flower, D. Gardner, and R. I. Willing, Phytochemistry, 1999, 51, 153; CrossRef For the total synthesis, see (b) C. Li, C. Chan, A. C. Heimann, and S. J. Danishefsky, Angew. Chem. Int. Ed., 2007, 46, 1448; CrossRef (c) J. D. Trzupek, D. Lee, B. M. Crowley, V. M. Marathias, and S. J. Danishefsky, J. Am. Chem. Soc., 2010, 132, 8506; CrossRef (d) H. Ding and D. Y.-K. Chen, Angew. Chem. Int. Ed., 2011, 50, 676. CrossRef
8. (a) T. Gözler, B. Gözler, I. Weiss, A. J. Freyer, and M. Shamma, J. Am. Chem. Soc., 1984, 106, 6101; CrossRef (b) G. Kadan, T. Gözler, and M. Shamma, J. Nat. Prod., 1990, 53, 531. CrossRef
9. For selected references, see: (a) S. Kato, M. Kanai, and S. Matsunaga, Chem. Asian. J., 2013, 8, 1768; CrossRef (b) F. Shi, Z.-L. Tao, S.-W. Luo, S.-J. Tu, and L.-Z. Gong, Chem. Eur. J., 2012, 18, 6885; CrossRef (c) H. Lv, B. Tiwari, J. Mo, C. Xing, and Y. R. Chi, Org. Lett., 2012, 14, 5412; CrossRef (d) J. J. Badillo, A. Silva-García, B. H. Shupe, J. C. Fettinger, and A. K. Franz, Tetrahedron Lett., 2011, 52, 5550; CrossRef (e) W.-B. Chen, Z.-J. Wu, J. Hu, L.-F. Cun, X.-M. Zhang, and W.-C. Yuan, Org. Lett., 2011, 13, 2472; CrossRef (f) S. Duce, F. Pesciaioli, L. Gramigna, L. Bernardi, A. Mazzanti, A. Ricci, G. Bartolo, and B. Bencivenni, Adv. Synth. Catal., 2011, 353, 860; CrossRef (g) S. Mouri, Z. Chen, H. Mitsunuma, M. Furutachi, S. Matsunaga, and M. Shibasaki, J. Am. Chem. Soc., 2010, 132, 1255; CrossRef (h) X. Cheng, S. Vellalath, R. Goddard, and B. List, J. Am. Chem. Soc., 2008, 130, 15786. CrossRef
10. C.-L. Zhu, F.-G. Zhang, W. Meng, J. Nie, D. Cahard, and J.-A. Ma, Angew. Chem. Int. Ed., 2011, 50, 5869. CrossRef
11. Reviews: (a) H. M. L. Davies, and J. R. Manning, Nature, 2008, 451, 417; CrossRef (b) C. G. Espino and J. Du Bois, ‘Modern Rhodium-Catalyzed Organic Reactions,’ ed. by P. A. Evans, Wiley-VHC: Weinheim, 2005; pp. 379−416; CrossRef (c) P. Müller and C. Fruit, Chem. Rev., 2003, 103, 2905. CrossRef
12. C. G. Espino and J. Du Bois, Angew. Chem. Int. Ed., 2001, 40, 598. CrossRef
13. For selected examples of the enantioselective nitrogen transfer reaction with chiral rhodium catalysts, see: (a) D. N. Zalatan and J. Du Bois, J. Am. Chem. Soc., 2008, 130, 9220; CrossRef (b) M. Anada, M. Tanaka, T. Washio, M. Yamawaki, T. Abe, and S. Hashimoto, Org. Lett., 2007, 9, 4559; CrossRef (c) C. Fruit and P. Müller, Tetrahedron: Asymmetry, 2004, 15, 1019. CrossRef
14. (a) A. Padwa and T. Stengel, Org. Lett., 2002, 4, 2137; CrossRef (b) A. Padwa, A. C. Flick, C. A. Leverett, and T. Stengel, J. Org. Chem., 2004, 69, 6377; CrossRef (c) J.-L. Liang, S.-X. Yuan, P. W. H. Chan, and C.-M. Che, Tetrahedron Lett., 2003, 44, 5917. CrossRef
15. For our previous reports on aza-spiroannulation onto indoles, see S. Sato, M. Shibuya, N. Kanoh, and Y. Iwabuchi, Chem. Commun., 2009, 6264. See also ref. 3 (b). CrossRef
16. For a recent report on iron(II)-catalyzed asymmetric intramolecular aza-spiroannulation, see: Y.-Q. Zhang, Y.-A. Yuan, G.-S. Liu, and H. Xu, Org. Lett., 2013, 15, 3910. CrossRef
17. (a) Z. Yuan, J. Trias, and R. J. White, Drug Discovery Today, 2001, 6, 954; CrossRef (b) A. S. Waller and J. M. Clements, Curr. Opin. Drug Discovery Dev., 2002, 5, 785.
18. To the best of our knowledge, only one example has been reported on the aza-spiroannulation of the benzofuran with Rh2(OAc)4 to date, see ref. 15 (b).
19. (a) M. Yamawaki, H. Tsutsui, S. Kitagaki, M. Anada, and S. Hashimoto, Tetrahedron Lett., 2002, 43, 9561; CrossRef (b) M. Yamawaki, M. Tanaka, T. Abe, M. Anada, and S. Hashimoto, Heterocycles, 2007, 72, 709; CrossRef (c) M. Tanaka, Y. Kurosaki, T. Washio, M. Anada, and S. Hashimoto, Tetrahedron Lett., 2007, 48, 8799. CrossRef
20. Three products (two diastereomers of a spirocycle and by-product) and the starting material 3 in the aza-spiroannulation reaction were inseparable by silica gel column chromatograhy. After the treatment of the above mixture with Boc2O, two diastereomers of 9, the by-product 10 were separable and completely purified. All other spirocyclic products were treated with Boc2O for easy purification.
21. Use of an alcohol as the additive in the intramolecular aza-spiroannulation reaction onto indole, see ref. 15 (b).
22. Use of an external nucleophile as the additive in the intra- and intermolecular aziridination reactions, see (a) E. Levites-Agababa, E. Menhaji, L. N. Perlson, and C. M. Rojas, Org. Lett., 2002, 4, 863; CrossRef (b) S. Beaumont, V. Pons, P. Retailleau, R. H. Dodd, and P. Dauban, Angew. Chem. Int. Ed., 2010, 49, 1634. CrossRef
23. (a) R. P. Reddy and H. M. L. Davies, Org. Lett., 2006, 8, 5013; CrossRef (b) H. Wang, D. M. Guptill, A. Varela-Alvarez, D. G. Musaev, and H. M. L. Davies, Chem. Sci., 2013, 4, 2844. CrossRef
24. (a) P. L. Polavarapu and J. He, Anal. Chem., 2004, 76, 61A; CrossRef (b) P. J. Stephens, F. J. Devlin, and J.-J. Pan, Chirality, 2008, 20, 643. CrossRef
25. For selected reports on the determination of the absolute stereostructure of natural products, see: (a) T. Asai, S. Morita, N. Shirata, T. Taniguchi, K. Monde, H. Sakurai, T. Ozeki, and Y. Oshima, Org. Lett., 2012, 14, 5456; CrossRef (b) T. Asai, T. Taniguchi, T. Yamamoto, K. Monde, and Y. Oshima, Org. Lett., 2013, 15, 4320. CrossRef
26. The absolute stereostructures of 16a−16c were determined as shown in Figure 3. For details, see: Supporting Information.
27. For the synthesis of naturally occurring (–)-fumimycin, Rh2(R-TCPTAD)4 should be used for the aza-spiroannulation.