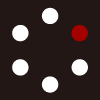
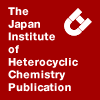
HETEROCYCLES
An International Journal for Reviews and Communications in Heterocyclic ChemistryWeb Edition ISSN: 1881-0942
Published online by The Japan Institute of Heterocyclic Chemistry
e-Journal
Full Text HTML
Received, 17th February, 2014, Accepted, 25th March, 2014, Published online, 4th April, 2014.
DOI: 10.3987/COM-14-S(K)8
■ Novel Intramolecular Cyclization-Skeletal Reorganization of 2-Arylthiazoles under Photoirradiation
Noriyoshi Arai,* Moe Mizota, and Takeshi Ohkuma*
Division of Chemical Process Engineering, Graduate School of Engineering, Hokkaido University, Kita 13 Nishi 8, Kita-ku, Sapporo, Hokkaido 060-8628, Japan
Abstract
Photoirradiation of 2-arylthiazoles derivatives linked with an alkene moiety through a three-atom spacer was investigated. The main products were unexpected tetrahydrofuran-fused thiazepine derivatives with concomitant formation of [2+2] cycloaddition products and regioisomeric thiazoles. The product distribution was little influenced by the reaction conditions and substituents of the substrates.The photochemical behavior of 5-membered heteroaromatic compounds is one of the major research areas of organic photochemistry, and has already inspired numerous reports.1–4 Among them, reactions involving carbon-carbon bond formation are of interest from a synthetic point of view. The [2+2] cycloaddition and electrocyclization are the typical examples;2–5 however, the construction of complex molecular frameworks by irradiation of simple molecules has been less developed.6–9 We have reported that photoirradiation of α-arylthiophene derivatives linked with an alkene moiety through a three-atom spacer 1 gave unprecedented cyclobutene-fused perhydrothiapentalene-type compounds 2 in high yields (Eq. 1).10 In the course of our study on the photoreaction of sulfur-containing 5-membered heteroaromatic compounds, we found that 2-arylthiazole derivatives under photoirradiation show interesting behavior that is completely different from the behavior of thiophenes.
Our study began with the photoirradiation of 2-phenylthiazole derivative 3a. Irradiation of a 5 mM solution of 3a in benzene at room temperature through Pyrex® glass gave the unexpected thiazepine 4a in moderate yield, accompanied by the [2+2] addition product 5a and isomerized isothiazole 6a, with small recovery of 3a (2%) (Eq. 2). The relative configuration of 5a was determined by NOE experiment (Figure 1). When Hb was irradiated, clear NOE enhancements were observed for the signals of Ha and protons of Mea, and vice versa. Irradiation at Hc also showed enhancements of the signals of Hd and Meb. This observation indicates that compound 5a has the relative configuration depicted in the figure.
It is well known that photo irradiation of thiazoles causes the isomerization to regioisomers,1,11,12 whereas examples of carbon-carbon bond formation have rarely been reported.13 As far as we know, there has been no report of thiazepine formation from thiazole under photoirradiation.14 We were interested in this extraordinary photoreaction of thiazole derivatives, and decided to investigate the reaction in detail.
Setting 3a as a standard substrate, we carried out the reaction in a range of solvents. The results are summarized in Table 1.
The reaction proceeded in hexane, 1,4-dioxane, ethyl acetate, and acetonitrile, as well as in benzene, to give the products in similar yields and distributions (Entries 1–5). These results clearly show that the reaction is hardly affected by the reaction media, and that it likely proceeds via non-polar intermediates. The reaction proceeded somewhat sluggishly in acetone (Entry 6). Competitive absorption by acetone (λmax = 279 nm, absorption edge ~ 330 nm)15 would likely have caused this result. This result also shows that participation of the triplet state is not important for the reaction. In the reactions in acetonitrile and acetone (Entries 5 and 6), unidentified broad multiplets that ranged 1.3–2.0 and 7.1–7.6 ppm were observed in 1H NMR measurement of the reaction mixtures. The formation of these by-products caused the low material balances.
As shown in Table 1, this reaction gave both thiazepine 4a and the [2+2] addition product 5a in similar ratios, almost irrespective of the solvent employed. We next examined the substituent effect at the 2-position of thiazoles on the product distribution. The results are shown in Table 2.
Introduction of 4-fluorophenyl group at the 2-position of thiazole gave better selectivity in favor of thiazepine 4b but the combined yield of 4b and 5b decreased (Entry 2). The substrate with a 4-methoxyphenyl group at the 2-position showed improved selectivity in favor of 4c without a decrease of the yield compared to 3a (Entry 3). However, the cyclized products in this case were unstable and decomposed during chromatographic purification on silica gel. The reactions with 2-methyl and 2-unsubstituted thiazoles hardly proceeded under the same conditions (Entries 4 and 5). This was attributed to the poor absorption of 3d and 3e in the range of >300 nm. Then we tried the reaction in the presence of 9,10-dicyanoanthracene (DCA) as a photosensitizer, which has previously been proven effective in several photocyclizations (Entry 6).16–18 The conversion was somewhat increased in this case, but no identifiable product was obtained.
To obtain more insight into the reaction, the stability of the isolated reaction products (4a, 5a, and 6a) under photoirradiation (in benzene, for 1 h) was checked. Isothiazole 6a was stable under the conditions employed, whereas partial decomposition was observed in the cases of 4a and 5a, to give a mixture of many compounds, including 6a. The inertness of 6a under the reaction conditions was attributed to the difference of photoabsorption between 6a and 3a. The absorption edge of 6a was approximately 300 nm and this compound absorbed almost no light through Pyrex® glass (approximately >300 nm), while the absorption edge of 3a was about 350 nm.
The mechanism of the reaction is not clear at present, and the details should be disclosed by further investigation. We surmise the reaction would proceed through the pathway depicted in Scheme 1. Thiazepine 4a could be formed via biradical species A, which was produced by the addition of the excited thiazole to the olefinic moiety, followed by successive [1,3]-transposition of sulfur to give the thiazepine 4a. A similar intermediate has been postulated in the photocyclization of furan.18 The [2+2] cycloaddition between thiazole and alkene likely gives the tricyclic compound 5a.13 The isomerization to isothiazole 6a would be caused by the formation of the Dewar thiazole B, which is involved as an intermediate in the photoisomerization of thiazole derivatives.1,11
In summary, we demonstrated a novel photochemical transformation of 2-arylthiazoles 3 that gave thiazepine derivatives 4 in moderate yield with concomitant formation of the [2+2] adduct 5 and isothiazole 6. This is an unprecedented category of photoreaction of thiazoles, although there remains room for improvement in the product selectivity and yield. Though the mechanism of the reaction is unclear at this stage, the thiazepine might be formed via a biradical intermediate A, followed by skeletal rearrangement.
EXPERIMENTAL
NMR spectra were obtained on a JEOL JNM-ECS400, and JNM-ECX400P spectrometer. Carbon multiplicity was assigned by a DEPT experiment. IR spectra were recorded on JASCO FT/IR-4100 spectrophotometer. Silica gel column chromatography was performed with Kanto Chemical Co., Inc. Silica Gel 60N (63-210 µm), or Fuji Silisia FL60D. Preparative thin layer chromatography was carried out with Wako Gel B-5F (Wako Pure Chemical Industries, Ltd.). Solvents and reagents were used as received, unless otherwise noted. Mass spectrometry and elemental analyses were carried out at the Instrumental Analysis Division, Equipment Management Center, Creative Research Institution, Hokkaido University.
Starting materials
The starting materials were prepared from known compounds according to conventional transformations, including the Williamson etherification of known 2-aryl-5-hydroxymethylthiazoles.19,20 A typical example is as follows:
5-(3-Methyl-2-butenyloxy)methyl-2-phenylthiazole (3a)
To a flask containing a dispersion of NaH (95.8 mg, 60% oil dispersion, 2.4 mmol, washed with hexane before use) in DMF (1 mL) was added a solution of 5-hydroxymethyl-2-phenylthiazole (269.7 mg, 1.41 mmol) in DMF (2 mL) at 0 °C with stirring. After stirring at rt for 30 min, 1-chloro-3-methyl-2-butene (280 µL, 2.5 mmol) was added at 0 °C, and the mixture was stirred for 2 h at rt. The reaction mixture was quenched by addition of cold water, followed by extraction with hexane–EtOAc (1:1, 20 mL x 3). The combined extracts were successively washed with water and brine, and dried with anhydrous sodium sulfate. Purification of the crude material by silica-gel column chromatography (hexane–EtOAc, 7:1 to 4:1) gave 3a as pale yellow oil (350.5 mg, 96%).
IR (KBr) 2975, 2865, 1456, 1433, 1377, 1357, 1068, 974, 763, 689 cm–1. 1H NMR (400 MHz, CDCl3) δ 1.68 (s, 3H), 1.77 (s, 3H), 4.04 (d, J = 6.8 Hz, 2H), 4.71 (s, 2H), 5.36–5.40 (m, 1H), 7.41–7.47 (m, 3H), 7.71 (s, 1H), 7.92–7.95 (m, 2H). 13C NMR (100 MHz, CDCl3) δ 18.0 (CH3), 25.7 (CH3), 63.7 (CH2), 66.2 (CH2), 120.3 (CH), 126.3 (CH), 128.8 (CH), 129.9 (CH), 133.6 (C), 135.8 (C), 137.9 (C), 142.1 (CH), 168.9 (C). HRMS (ESI+) m/z 260.1106 (M+H+), calcd for C15H18NOS: 260.1104.
2-(4-Fluorophenyl)-5-(3-methyl-2-butenyloxy)methylthiazole (3b)
Faintly yellow solid. Mp 39.5–41.0 °C (CH2Cl2). IR (KBr) 2971, 2851, 1600, 1509, 1443, 1226, 1066, 999, 981, 856, 835 cm–1. 1H NMR (400 MHz, CDCl3) δ 1.68 (s, 3H), 1.77 (s, 3H), 4.04 (d, J = 6.8 Hz, 2H), 4.70 (s, 2H), 5.35–5.40 (m, 1H), 7.11–7.16 (m, 2H), 7.68 (s, 1H), 7.90–7.94 (m, 2H). 13C NMR (100 MHz, CDCl3) δ 18.1 (CH3), 25.8 (CH3), 63.7 (CH2), 66.4 (CH2), 116.0 (d, JC–F = 22.5 Hz, CH), 120.3 (CH), 128.2 (d, JC–F = 8.5 Hz, CH), 130.0 (C), 136.0 (C), 138.1 (C), 142.2 (CH), 163.8 (d, JC–F = 250 Hz, C), 167.8 (C). HRMS (ESI+) m/z 278.1006 (M+H+), calcd for C15H17FNOS: 278.1009.
2-(4-Methoxyphenyl)-5-(3-methyl-2-butenyloxy)methylthiazole (3c)
Yellow oil. IR (KBr) 2933, 2853, 1607, 1515, 1450, 1254, 1173, 1069, 1034, 975, 834 cm–1. 1H NMR (400 MHz, CDCl3) δ 1.67 (s, 3H), 1.77 (s, 3H), 3.86 (s, 3H), 4.03 (d, J = 7.3 Hz, 2H), 4.68 (s, 2H), 5.36–5.39 (m, 1H), 6.95 (d, J = 8.6 Hz, 2H), 7.64 (s, 1H), 7.86 (d, J = 8.6 Hz, 2H). 13C NMR (100 MHz, CDCl3) δ 18.0 (CH3), 25.8 (CH3), 55.3 (CH3), 63.8 (CH2), 66.2 (CH2), 114.2 (CH), 120.4 (CH), 126.6 (C), 126.6 (CH), 127.8 (CH), 134.8 (C), 138.0 (C), 142.0 (CH), 161.1 (C), 169.0 (C). HRMS (ESI+) m/z 290.1209 (M+H+), calcd for C16H20NO2S: 290.1209.
2-Methyl-5-(3-methyl-2-butenyloxy)methylthiazole (3d)
Light yellow oil. IR (KBr) 2972, 2926, 2856, 1674, 1536, 1472, 1446, 1377, 1359, 1161, 1068, 967, 903 cm–1. 1H NMR (400 MHz, CDCl3) δ 1.66 (s, 3H), 1.76 (s, 3H), 2.69 (s, 3H), 3.99 (d, J = 6.8 Hz, 2H), 4.62 (s, 2H), 5.33–5.37 (m, 1H), 7.48 (s, 1H). 13C NMR (100 MHz, CDCl3) δ 17.9 (CH3), 19.2 (CH3), 25.7 (CH3), 63.6 (CH2), 66.0 (CH2), 120.3 (CH), 135.2 (C), 137.8 (C), 140.8 (CH), 166.9 (C). HRMS (ESI+) m/z 198.0950 (M+H+), calcd for C10H16NOS: 198.0947.
5-(3-Methyl-2-butenyloxy)methylthiazole (3e)
Light yellow oil. IR (KBr) 2973, 2912, 2857, 1673, 1521, 1407, 1377, 1358, 1068, 873 cm–1. 1H NMR (400 MHz, CDCl3) δ 1.67 (s, 3H), 1.76 (s, 3H), 4.01 (d, J = 7.1 Hz, 2H), 4.71 (s, 2H), 5.34–5.38 (m, 1H), 7.79 (s, 1H), 8.79 (s, 1H). 13C NMR (100 MHz, CDCl3) δ 17.8 (CH3), 25.6 (CH3), 63.2 (CH2), 66.2 (CH2), 120.1 (CH), 135.7 (C), 137.8 (C), 141.7 (CH), 153.5 (CH). HRMS (ESI+) m/z 184.0793 (M+H+), calcd for C9H14NOS: 184.0791.
General procedure for the photocyclization and physical data of the products
The reaction of 3a is representative.
In a Pyrex® test tube (or reaction vessel (for preparative scale)) were charged 3a (13.3 mg, 0.0513 mmol) and benzene (10 mL, degassed by N2 bubbling for 30 min), and air present in the tube was replaced with argon by several cycles of rapid evacuation/Ar introduction. The solution was irradiated by a 100 W high-pressure mercury lamp (UVL-100HA; Riko) externally for 2 h. After the solution was evaporated, pyrazine (30 μL of 0.132 M solution in benzene, 4.0 μmol) was added to the mixture as an internal standard. The yields of 4a, 5a, and 6a were estimated by 1H NMR integrals. The pure sample for analysis could be isolated by chromatographic purification with case-dependent loss due to decomposition.
1,3,3a,4-Tetrahydro-4,4-dimethyl-6-phenylfuro[3,4-e][1,3]thiazepine (4a)
Light yellow oil. IR (KBr) 2965, 1750, 1727, 1671, 1602, 1551, 1447, 1368, 1222, 1096, 1056, 930, 767, 693 cm–1. 1H NMR (400 MHz, CDCl3) δ 1.52 (s, 3H), 1.57 (s, 3H), 3.31 (br m, 1H), 3.81 (dd, J = 9.4, 5.1 Hz, 1H), 3.93 (dd, J = 9.4, 7.6 Hz, 1H), 4.47–4.51 (m, 1H), 4.60–4.64 (m, 1H), 6.98–7.00 (m, 1H), 7.24–7.28 (m, 1H), 7.36–7.43 (m, 3H), 7.94–7.97 (m, 2H). 13C NMR (100 MHz, CDCl3) δ 26.3 (CH3), 31.7 (CH3), 54.0 (CH), 63.7 (C), 70.7 (CH2), 71.4 (CH2), 128.2 (CH), 128.3 (CH), 128.6 (CH), 130.6 (CH), 133.1 (C), 140.7 (C), 162.5 (C). HRMS (ESI+) m/z 260.1106 (M+H+), calcd for C15H18NOS: 260.1109.
3a,4,4a,5-Tetrahydro-4,4-dimethyl-2-phenyl-7H-furo[3',4':2,3]cyclobuta[1,2-d][1,3]thiazole (5a)
Faintly yellow oil. IR (KBr) 2960, 2861, 1594, 1446, 1065, 957, 941, 912, 767, 702, 690 cm–1. 1H NMR (400 MHz, CDCl3) δ 1.14 (s, 3H), 1.24 (s, 3H), 2.63 (d, J = 5.9 Hz, 1H), 3.55 (d, J = 9.4 Hz, 1H), 3.70 (dd, J = 10.4, 5.9 Hz, 1H), 3.98 (d, J=9.4 Hz, 1H), 4.08 (d, J = 10.4 Hz, 1H), 4.77 (d, J = 0.8 Hz, 1H), 7.40–7.50 (m, 3H), 7.79–7.82 (m, 2H). 13C NMR (100 MHz, CDCl3) δ 22.8 (CH3), 24.6 (CH3), 43.5 (C), 57.5 (CH), 61.9 (C), 69.7 (CH2), 72.8 (CH2), 90.6 (CH), 128.4 (CH), 128.5 (CH), 130.6 (CH), 133.3 (CH), 133.2 (C), 168.3 (C). HRMS (ESI+) m/z 260.1105 (M+H+), calcd for C15H18NOS: 260.1109.
4-(3-Methylbut-2-enyloxy)methyl-5-phenylisothiazole (6a)
Light yellow oil. IR (KBr) 2971, 2914, 2855, 1486, 1444, 1062, 769, 700 cm–1. 1H NMR (400 MHz, CDCl3) δ 1.66 (s, 3H), 1.75 (s, 3H), 4.07 (d, J = 7.2 Hz, 2H), 4.74 (s, 2H), 5.38–5.41 (m, 1H), 7.37–7.48 (m, 3H), 7.69 (d, J = 7.2 Hz, 2H), 8.80 (s, 1H). 13C NMR (100 MHz, CDCl3) δ 18.0 (CH3), 25.8 (CH3), 63.7 (CH2), 66.8 (CH2), 120.3 (CH), 128.1 (CH), 128.5 (CH), 128.7 (CH), 130.4 (C), 134.4 (C), 138.3 (C), 151.7 (CH), 153.4 (C). HRMS (ESI+) m/z 282.0924 (M+Na+), calcd for C15H17NNaOS: 282.0929.
6-(4-Fluorophenyl)-1,3,3a,4-tetrahydro-4,4-dimethylfuro[3,4-e][1,3]thiazepine (4b)
Yellow oil. IR (KBr) 2961, 2925, 2854, 1752, 1599, 1502, 1231, 1155, 1057, 1036, 842, 809 cm–1. 1H NMR (400 MHz, CDCl3) δ 1.51 (s, 3H), 1.56 (s, 3H), 3.29 (br m, 1H), 3.80 (dd, J = 9.4, 5.0 Hz 1H), 3.93 (dd, J = 9.4, 7.2 Hz, 1H), 4.48 (d, J = 13.5 Hz, 1H), 4.62 (dt, Jd = 13.5, Jt = 1.8 Hz, 1H), 6.96 (d, J = 1.8 Hz, 1H), 7.03–7.08 (m, 2H), 7.96–7.99 (m, 2H). 13C NMR (100 MHz, CDCl3) δ 26.2 (CH3), 31.7 (CH3), 53.9 (CH), 63.9 (C), 70.7 (CH2), 71.4 (CH2), 115.1 (d, JC–F = 21.7 Hz, CH), 128.6 (CH), 130.3 (d, JC–F = 8.4 Hz, CH), 133.3 (C), 136.9 (C), 161.1 (C), 164.4 (d, JC–F = 251 Hz, C). HRMS (ESI+) m/z 278.1006 (M+H+), calcd for C15H17FNOS: 278.1009.
2-(4-Fluorophenyl)-3a,4,4a,5-tetrahydro-4,4-dimethyl-7H-furo[3',4':2,3]cyclobuta[1,2-d][1,3]thiazole (5b)
Yellow oil. IR (KBr) 2961, 2860, 1606, 1507, 1263, 1234, 1156, 1065, 957, 942, 912, 842 cm–1. 1H NMR (400 MHz, CDCl3) δ 1.13 (s, 3H), 1.23 (s, 3H), 2.63 (d, J = 6.3 Hz, 1H), 3.55 (d, J = 9.4 Hz, 1H), 3.69 (dd, J = 10.3, 6.3 Hz, 1H), 3.98 (d, J = 9.4 Hz, 1H), 4.08 (d, J = 10.3 Hz, 1H), 4.75 (s, 1H), 7.11 (t, J = 8.5 Hz, 2H), 7.80 dd, J = 8.5, 5.4 Hz, 2H). 13C NMR (100 MHz, CDCl3) δ 22.8 (CH3), 24.6 (CH3), 43.5 (C), 57.5 (CH), 62.3 (C), 69.7 (CH2), 72.8 (CH2), 90.7 (CH), 115.6 (d, JC–F = 22.6 Hz, CH), 129.5 (C), 130.5 (d, JC–F = 8.4 Hz, CH), 164.5 (d, JC–F = 253 Hz, C), 166.9 (C). HRMS (ESI+) m/z 278.1007 (M+H+), calcd for C15H17FNOS: 278.1009.
5-(4-Fluorophenyl)-4-(3-methylbut-2-enyloxy)methylisothiazole (6b)
Colorless oil. IR (KBr) 3065, 2914, 2861, 1672, 1605, 1497, 1224, 1159, 1064, 882, 844 cm–1. 1H NMR (400 MHz, CDCl3) δ 1.67 (s, 3H), 1.76 (s, 3H), 4.07 (d, J = 6.8 Hz, 2H), 4.70 (s, 2H), 5.37–5.41 (m, 1H), 7.12–7.18 (m, 2H), 7.66–7.71 (m, 2H), 8.79 (s, 1H). 13C NMR (100 MHz, CDCl3) δ 18.0 (CH3), 25.8 (CH3), 63.4 (CH2), 66.8 (CH2), 115.4 (d, JC–F = 20.6 Hz, CH), 120.2 (CH), 129.9 (C), 130.5 (d, JC–F = 8.4 Hz, CH), 138.4 (C), 151.7 (CH), 152.6 (C), 162.7 (d, JC–F = 248 Hz, C). HRMS (ESI+) m/z 278.1008 (M+H+), calcd for C15H17FNOS: 278.1009.
1,3,3a,4-Tetrahydro-6-(4-methoxyphenyl)-4,4-dimethylfuro[3,4-e][1,3]thiazepine (4c)
3a,4,4a,5-Tetrahydro-2-(4-methoxyphenyl)-4,4-dimethyl-7H-furo[3',4':2,3]cyclobuta[1,2-d][1,3]thia-zole (5c)
We could isolate neither 4c nor 5c due to the rapid decomposition of these compounds during chromatographic purification. The yields shown in Table 2 were estimated from 1H NMR of the crude mixture by using pyrazine as an internal standard. The assignment of signals was based on the analogy of 4a and 5a.
5-(4-Methoxyphenyl)-4-(3-methylbut-2-enyloxy)methylisothiazole (6c)
Faintly yellow oil. IR (KBr) 2972, 2914, 2858, 1605, 1497, 1224, 1158, 1064, 844 cm–1. 1H NMR (400 MHz, CDCl3) δ 1.67 (s, 3H), 1.76 (s, 3H), 3.86 (s, 3H), 4.07 (d, J = 6.7 Hz, 2H), 4.71 (s, 2H), 5.38–5.41 (m, 1H), 6.97–7.00 (m, 2H), 7.62–7.66 (m, 2H), 8.78 (s, 1H). 13C NMR (100 MHz, CDCl3) δ 18.0 (CH3), 25.8 (CH3), 55.3 (CH3), 63.7 (CH2), 66.8 (CH2), 113.9 (CH), 120.3 (CH), 127.0 (C), 128.9 (C), 130.0 (CH), 138.2 (C), 151.5 (CH), 153.3 (C), 159.5 (C). HRMS (ESI+) m/z 290.1208 (M+H+), calcd for C16H20NO2S: 290.1209.
ACKNOWLEDGMENTS
This work was supported by JSPS KAKENHI Grant Number 25410105 and the MEXT (Japan) program "Strategic Molecular and Materials Chemistry through Innovative Coupling Reactions" of Hokkaido University.
References
1. M. D'Auria, Targets Heterocycl. Syst., 1998, 2, 233.
2. M. D'Auria, 'Advances in Heterocyclic Chemistry: Photochemical and Photophysical Behavior of Thiophene,' Vol. 104, ed. by A. R. Katritzky, Academic Press, Inc., London, 2011, pp. 127–390.
3. J. W. Pavlik, 'Organic Photochemistry: The Photochemistry of Pyrazoles and Isothiazoles,' ed. by V. Ramamurthy and K. S. Schanze, Marcel Dekker, Inc., New York, 1997, pp. 57–84.
4. M. Abe, 'Handbook of Synthetic Photochemistry: Formation of a Four-membered Ring: Oxetanes,' ed. by A. Albini and M. Fagnoni, Wiley-VCH, Weinheim, 2010, pp. 217–239.
5. T. S. Cantrell, J. Chem. Soc., Chem. Commun., 1972, 155. CrossRef
6. M. T. Crimmins, J. M. Pace, P. G. Nantermet, A. S. Kim-Meade, J. B. Tomas, S. H. Watterson, and A. S. Wagman, J. Am. Chem. Soc., 2000, 122, 8453. CrossRef
7. N. Basarić, Ž. Marinić, and M. Šindler-Kulyk, J. Org. Chem., 2006, 71, 9382. CrossRef
8. K. G. Maskill, J. P. Knowles, L. D. Elliott, R. W. Alder, and K. I. Booker-Milburn, Angew. Chem. Int. Ed., 2013, 52, 1499. CrossRef
9. Reviews: T. Bach and J. P. Hern, Angew. Chem. Int. Ed., 2011, 50, 1000; N. Hoffmann, Chem. Rev., 2008, 108, 1052. CrossRef
10. N. Arai, K. Tanaka, and T. Ohkuma, Org. Lett., 2012, 14, 1488. CrossRef
11. G. Vernin, R. Jauffred, C. Ricard, H. J. M. Dou, and J. Metzger, J. Chem. Soc., Perkin Trans. 2, 1972, 1145. CrossRef
12. C. Riou, G. Vernin, H. J. M. Dou, and J. Metzger, Bull. Soc. Chim. Fr., 1972, 2673.
13. T. Nakano, W. Rodríguez, S. Z. de Roche, J. M. Larrauri, C. Rivas, and C. Pérez, J. Heterocycl. Chem., 1980, 17, 1777. CrossRef
14. Photocycloadditions of benzothiazoles with alkenes are known to give 1,5-benzothiazepines: M. Sindler-Kulyk and D. C. Neckers, Tetrahedron Lett., 1981, 22, 2081; CrossRef G. Kaupp and E. Ringer, Chem. Ber., 1986, 119, 1525. CrossRef
15. J. Catalán and J. P. Catalán, Phys. Chem. Chem. Phys., 2011, 13, 4072. CrossRef
16. C. R. Jones, B. J. Allman, A. Mooring, and B. Spahic, J. Am. Chem. Soc., 1983, 105, 652. CrossRef
17. N. Akbulut, D. Hartsough, J.-I. Kim, and G. B. Schuster, J. Org. Chem., 1989, 54, 2549. CrossRef
18. N. Arai, K. Tanaka, and T. Ohkuma, Tetrahedron Lett., 2010, 51, 1273. CrossRef
19. R. Dahlbom, Acta Chem. Scand., 1953, 7, 885. CrossRef
20. K. Suzuki, T. Yamaguchi, A. Tamura, T. Nishizawa, and M. Yamaguchi, PCT Int. WO 2010004972 A1 20100114, 2010 (Chem. Abstr., 2010, 152, 144657).