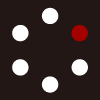
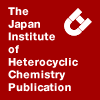
HETEROCYCLES
An International Journal for Reviews and Communications in Heterocyclic ChemistryWeb Edition ISSN: 1881-0942
Published online by The Japan Institute of Heterocyclic Chemistry
e-Journal
Full Text HTML
Received, 26th February, 2014, Accepted, 25th March, 2014, Published online, 3rd April, 2014.
DOI: 10.3987/COM-14-S(K)11
■ Studies on the Effect of N-Heterocyclic Carbene as a Ligand for Nickel(II)-Catalyzed Polymerization of Thiophenes
Atsunori Mori,* Makoto Fujio, and Shunsuke Tamba
Chemical Science & Technology, Graduate School of Engineering, Kobe University, 1-1 Rokkodai-cho, Nada-ku, Kobe 657-8501, Japan
Abstract
Effect of N-heterocyclic carbene (NHC) ligand in the nickel-catalyzed polythiophene synthesis is studied. One-pot protocol to form nickel-NHC ligand with the corresponding HCl salt of NHC and Ni(acac)2 in the presence of a base and following deprotonation and polymerization smoothly affords poly(3-hexylthiophen-2,5-diyl) (2a). Among NHC ligands examined, imidazolidine derived NHC bearing 2,6-diisopropylphenyl group as an N-substituent is found to be the optimum ligand.INTRODUCTION
N-Heterocyclic carbene (NHC) attracts much attention in transition metal catalysis.1 Remarkable effects of the carbene as a ligand in a variety of metal complexes have been shown enhancing the catalytic activity, which improve efficiencies of metal-catalyzed coupling reactions2 and thus leads to development of synthesis of advanced organic materials.3 We have recently shown that dehydrochlorinative cross coupling polymerization of 2-chloro-3-substituted thiophenes (1) leading to highly regioregular poly(3-substituted thiophene)s (P3RT 2) in a head-to-tail manner.4 The reaction takes place highly efficiently with a nickel(II) catalyst bearing NHC ligand N,N'-(2,6-diisopropylphenyl)imidazolium (IPr) whereas bidentate phosphine exhibited inferior activity. Although several bidentate phosphines such as DPPE (1,2-diphenylphoshinoethane) and DPPP (1,3-diphenylphosphinopropane) have been revealed as an effective ligand of nickel(II) in the Grignard metathesis (GRIM)5,6 and dehydrobrominative polymerizations7 of 2,5-dibromo-3-substitued thiophene and 2-bromo-3-substituted thiophene, the reaction of less reactive chlorothiophene derivative suggested that the effect of NHC ligand seems superior. Our interest accordingly turned to the effect of NHC as a ligand for the nickel catalyst. However, the limited nickel(II) complex NiCl2(PPh3)IPr is readily available, while it is difficult to simply prepare other related nickel complexes bearing NHC ligands. It is thus intriguing to perform in situ preparation protocol of nickel NHC complex with the corresponding imidazolium (imidazolidinium) salt via dehydrochlorination leading to NHC, which would allow to examine a variety of NHC ligand bearing different substituents. Herein, we describe a facile method for the in situ generation of NiIINHC complexes to study the ligand effect for the polythiophene synthesis.
RESULTS AND DISCUSSION
We first examined the reaction of NHC ligand with NiCl2(PPh3)2 for the in situ formation of Ni-NHC complex and addition of the thus formed solution to the metalated thiophene, which was generated by the reaction of 2-chloro-3-hexylthiophenbe (1a) with Knochel-Hauser base chloromagnesium 2,2,6,6-tetramethylpiperidide lithium chloride salt (TMPMgCl·LiCl).8 However, the polymerization reaction did not proceed smoothly compared with the similar reaction to that with NiCl2(PPh3)IPr probably because of insufficient ligand exchange. Thus, use of nickel(II) acetylacetonate Ni(acac)29 was employed for the precatalyst, which induced the polymerization efficiently. The reaction was carried out in the following protocol (Scheme 2): Addition of TMPMgCl·LiCl (0.55 mmol) to a solution of imidazole or imidazolidine HCl salt (0.005 mmol) in THF to result in dehydrochlorination by the magnesium amide lead to form free NHC ligand. Ni(acac)2 (0.005 mmol) was then added after stirring for 30 min. To the resulting mixture of the formed catalytic nickel complex in the presence of excess remaining Knochel-Hauser base addition of 2-chloro-3-hexylthiophene (1a, 0.5 mmol) followed to undergo deprotonation to form the corresponding metalated thiophene to initiate the polymerization. For comparison of the reactivity of NHC ligand, the reaction was performed at a lower temperature 0 °C and terminated after stirring for 1 h. As shown in Table 1, conversion of 1a into poly(3-hexylthiophen-2,5-diyl) (2a) and the average molecular weight (Mn) and molecular weight distribution (Mw/Mn) were summarized. The imidazolidinium salt 3a corresponding to IPr was found to afford P3HT in 32% conversion and indicated reasonable Mn and Mw/Mn values when the polymerization occurred in a living manner. The related reaction with NiCl2(PPh3)IPr also showed similar conversion to the above case. Use of IMes and SIMes ligands bearing 2,4,6-trimethylphenyl group as N-substituents resulted in inferior conversion with the formation of low molecular weight oligomers. By contrast, the reaction with SIPr indicated the improved reactivity resulting in 48% conversion. In addition, the reaction with several bidentate phosphines DPPE and DPPP was also examined. These phosphine ligands were shown to be ineffective for the polymerization of chlorothiophene 1 although the difference of reactivity had been hardly found in the reaction with a more reactive bromothiophene.
With the reaction protocol using Ni(acac)2, the reaction of chlorothiophene 1a was subjected to the complete conversion to P3HT. Polymerization was found to complete by the reaction at room temperature for 5 h with 1 mol % of the nickel catalyst and the molecular weight of the obtained polymer agreed with the theoretical value based on the ratio of monomer feed/catalyst loading.10 The reaction with the different ratio of 1a/catalyst also resulted to show the linear relationship as represented in Figure 1.
The in situ protocol was also found to be available for the polymerization of 2-bromo-3-hexylthiophene with SIPr as a NHC ligand to result in >95% conversion (isolated yield: 51%) at room temperature for 5 h to afford 2a (Mn = 18300, Mw/Mn = 1.45). However, the attempted polymerization of 2,5-dibromo-3-hexylthiophene, which was carried out by the addition of iPrMgCl·LiCl5 instead of Knochel-Hauser base, was found unsuccessful suggesting that in situ and one-shot GRIM metalation inhibited the nickel-catalyzed cross-coupling polymerization.
In summary, we have examined the effect of NHC ligand in the polythiophene synthesis to find that SIPr is highly effective. In situ generation of the nickel complex bearing NHC ligand with Ni(acac)2 in the presence of Knochel-Hauser base was successfully performed and following one-shot deprotonative formation of metalated thiophene and nickel-catalyzed polymerization sequence lead to deliver poly(3-substituted thiophene) derivatives in a facile manner.
EXPERIMENTAL
General. All the reactions were carried out under nitrogen atmosphere. 1H NMR (300 MHz) and 13C NMR (75 MHz) spectra were measured on Varian Gemini 300. The chemical shifts were expressed in ppm with CHCl3 (7.26 ppm for 1H) or CDCl3 (77.0 ppm for 13C) as internal standards. SEC (size exclusion chromatography) analyses were performed with a standard HPLC system equipped with a UV detector at 30 °C using CHCl3 as an eluent with a Tosoh TSKgel GMHHR-M. Molecular weights and molecular weight distributions were estimated on the basis of the calibration curve obtained by 8 standard polystyrenes. For thin layer chromatography (TLC) analyses throughout this work, Merck precoated TLC plates (silica gel 60 F254) were used. TMPMgCl·LiCl was prepared by following the literature procedure12 and stored in the freezer as a 1.0 M THF solution. THF (anhydrous grade) was purchased from Kanto Chemicals Co., Ltd. and stored under nitrogen atmosphere. Other materials were purchased and used without further purification.
Synthesis of P3HT by in situ formed nickel(II) NHC complex by the reaction of Ni(acac)2, NHC·HCl salt, and Knochel Hauser base: To a solution of 1,3-di(2,6-diisopropyl)phenylimidazolidinium chloride (SIPr·HCl, 2.1 mg, 0.005 mmol) in 2.0 mL THF was added chloromagnesium 2,2,6,6-tetramethylpiperidine lithium chloride salt (TMPMgCl·LiCl) (0.55 mL, 1 M THF solution, 0.55 mmol). Nickel(II) acetylacetonate (1.3 mg, 0.005 mmol) was added to the mixture and stirring was continued at room temperature for 30 min. The solution was diluted with 2.5 mL THF and addition of 2-chloro-3-hexylthiophene (1a, 0.10 g, 0.5 mmol) was followed at 0 °C to initiate deprotonation/polymerization. The reaction was terminated by addition of HCl/MeOH to form dark purple precipitate, which was filtered off on a suction funnel. The residue was repeatedly washed with MeOH and then hexanes. The combined filtrate was extracted CHCl3 and the organic layer was dried over anhydrous sodium sulfate. Removal of solvent left a crude oil, which was subjected to 1H NMR analysis to estimate the conversion by measuring the remaining amount of 1a (ca. 90% conv.). The crude oil was poured into MeOH with vigorous stirring to form dark purple solid. The mixture was filtered off and the residue was dried under reduced pressure to afford polythiophene 2a.13 The obtained polymer was subjected to SEC analysis.
ACKNOWLEDGEMENTS
This work was partially supported by Kakenhi (B) (No. 25288049) by JSPS (Japan Society for Promotion of Science). S. T. thanks JSPS Fellowship for Young Scientists.
References
1. V. P. W. Böhm, T. Weskamp, C. W. K. Gstöttmayr, and W. A. Herrmann, Angew. Chem. Int. Ed., 2000, 39, 1602. CrossRef
2. F. Diederich and P. J. Stang, Eds. Metal-Catalyzed Cross-Coupling Reaction; Wiley-VCH: Weinheim, 1998. CrossRef
3. a) I. Osaka and R. D. McCullough, Acc. Chem. Res., 2008, 41, 1202; CrossRef b) K. Takimiya, S. Shinamura, I. Osaka, and E. Miyazaki, Adv. Mater., 2011, 23, 4347; CrossRef c) A. Pron, P. Gawrys, M. Zagorska, D. Djurado, and R. Demadrille, Chem. Soc. Rev., 2010, 39, 2577; CrossRef d) D. J. Schipper and K. Fagnou, Chem. Mater., 2011, 23, 1594; CrossRef e) K. Okamoto, J. Zhang, J. B. Housekeeper, S. R. Marder, and C. K. Luscombe, Macromolecules, 2013, 46, 8059. CrossRef
4. a) S. Tamba, K. Shono, A. Sugie, and A. Mori, J. Am. Chem. Soc., 2011, 133, 9700; CrossRef See also: b) K. Fuji, S. Tamba, K. Shono, A. Sugie, and A. Mori, J. Am. Chem. Soc., 2013, 135, 12208; CrossRef c) S. Tamba, K. Ide, K. Shono, A. Sugie, and A. Mori, Synlett, 2013, 24, 1133. CrossRef
5. a) H. A. Bronstein and C. K. Luscombe, J. Am. Chem. Soc., 2009, 131, 12894; CrossRef b) N. Doubina, A. Ho, A. K.-Y. Jen, and C. K. Luscombe, Macromolecules, 2009, 42, 7670; CrossRef c) S. D. Boyd, A. K. Y. Jen, and C. K. Luscombe, Macromolecules, 2009, 42, 9387; CrossRef d) E. L. Lanni and A. J. McNeil, J. Am. Chem. Soc., 2009, 131, 16573; CrossRef e) R. Tkachov, V. Senkovskyy, H. Komber, J.-U. Sommer, and A. Kiriy, J. Am. Chem. Soc., 2010, 132, 7803; CrossRef f) E. L. Lanni and A. J. McNeil, Macromolecules, 2010, 43, 8039; CrossRef g) V. Senkovskyy, M. Sommer, R. Tkachov, H. Komber, W. T. S. Huck, and A. Kiriy, Macromolecules, 2010, 43, 10157; CrossRef h) N. Doubina, S. A. Paniagua, A. V. Soldatova, A. K.-Y. Jen, S. R. Marder, and C. K. Luscombe, Macromolecules, 2011, 44, 512; CrossRef i) A. Smeets, P. Willot, J. D. Winter, P. Gerbaux, T. Verbiest, and G. Koeckelberghs, Macromolecules, 2011, 44, 6017; CrossRef j) N. Doubina, S. A. Paniagua, A. V. Soldatova, A. K. Y. Jen, S. R. Marder, and C. K. Luscombe, Macromolecules, 2011, 44, 512; CrossRef k) R. S. Loewe, S. M. Khersonsky, and R. D. McCullough, Adv. Mater., 1999, 11, 250; CrossRef l) A. Yokoyama, R. Miyakoshi, and T. Yokozawa, Macromolecules, 2004, 37, 1169; CrossRef m) R. Miyakoshi, A. Yokoyama, and T. Yokozawa, J. Am. Chem. Soc., 2005, 127, 17542. CrossRef
6. For reviews: a) T. Yokozawa and A. Yokoyama, Chem. Rev., 2009, 109, 5595; CrossRef b) R. D. McCullough, Adv. Mater., 1998, 10, 93; CrossRef c) Z. J. Bryan and A. J. McNeil, Macromolecules, 2013, 46, 8395. CrossRef
7. a) S. Tamba, S. Tanaka, Y. Okubo, S. Okamoto, H. Meguro, and A. Mori, Chem. Lett., 2011, 40, 398; CrossRef b) S. Tamba, S. Mitsuda, F. Tanaka, A. Sugie, and A. Mori, Organometallics, 2012, 31, 2263; CrossRef c) S. Tanaka, S. Tamba, D. Tanaka, A. Sugie, and A. Mori, J. Am. Chem. Soc., 2011, 133, 16734; CrossRef d) S. Tamba, K. Fuji, H. Meguro, S. Okamoto, T. Tendo, R. Komobuchi, A. Sugie, T. Nishino, and A. Mori, Chem. Lett., 2013, 40, 281; CrossRef e) K. Nakamura, S. Tamba, A. Sugie, and A. Mori, Chem. Lett., 2013, 40, 1200; CrossRef f) S. Tamba, K. Fuji, K. Nakamura, and A. Mori, Organometallics, 2014, 33, 12; CrossRef g) A. Mori, K. Ide, S. Tamba, S. Tsuji, Y. Toyomori, and T. Yasuda, Chem. Lett., 2014, 43, 640; For a review, see: h) A. Mori, J. Synth. Org. Chem. Jpn., 2011, 69, 1202. CrossRef
8. a) A. Krasovskiy, V. Krasovskaya, and P. Knochel, Angew. Chem. Int. Ed., 2006, 45, 2958; CrossRef b) W. Lin, O. Baron, and P. Knochel, Org. Lett., 2006, 8, 5673; CrossRef c) G. C. Clososki, C. J. Rohbogner, and P. Knochel, Angew. Chem. Int. Ed., 2007, 46, 7681; CrossRef d) C. J. Rohbogner, G. C. Clososki, and P. Knochel, Angew. Chem. Int. Ed., 2008, 47, 1503; CrossRef e) A. H. Stoll and P. Knochel, Org. Lett., 2008, 10, 113; CrossRef f) M. Mosrin and P. Knochel, Org. Lett., 2008, 10, 2497; CrossRef g) F. M. Piller and P. Knochel, Org. Lett., 2009, 11, 445. CrossRef
9. Acac: acetylacetonate. a) K. Matsubara, S. Miyazaki, Y. Koga, Y. Nibu, T. Hashimura, and T. Matsumoto, Organometallics, 2008, 27, 6020; CrossRef See also: b) K. Matsubara, K. Ueno, and Y. Shibata, Organometallics, 2006, 25, 3422; CrossRef c) W. A. Herrmann, G. Gerstberger, and M. Spiegler, Organometallics, 1997, 16, 2209. CrossRef
10. The reaction with IPr·HCl under similar conditions also resulted in 90% conv. (43% isolated yield) with Mn = 13100 (Mw/Mn = 1.40) at the 1 mol % catalyst loading.
11. The reaction was carried out with 0.5, 1.0, 2.0, 3.0 mol % of nickel catalyst toward 2-chloro-3-hexylthiophene (1a) to result in giving conversion of 90% (Mn = 29900, Mw/Mn = 1.48), 90% (Mn = 14900, Mw/Mn = 1.35), 92% (Mn = 7800, Mw/Mn = 1.28), and 94% (Mn = 5090, Mw/Mn = 1.29), respectively.
12. H.-Q. Do and O. Daugulis, J. Am. Chem. Soc., 2009, 131, 17052. CrossRef
13. The obtained polymer was confirmed to be identical with the authentic sample (ref 5) by measurements of 1H and 13C NMR analyses.