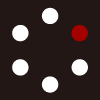
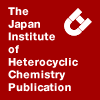
HETEROCYCLES
An International Journal for Reviews and Communications in Heterocyclic ChemistryWeb Edition ISSN: 1881-0942
Published online by The Japan Institute of Heterocyclic Chemistry
e-Journal
Full Text HTML
Received, 15th January, 2014, Accepted, 29th January, 2014, Published online, 6th February, 2014.
DOI: 10.3987/COM-14-12944
■ Synthesis of a Phosphatidylinositol Dimannoside Using 2-(Azidomethyl)benzoate Mannosyl Donors
Shuichi Ohira, Yoshiki Yamaguchi, Takashi Takahashi, and Hiroshi Tanaka*
Department of Applied Chemistry, Graduate School of Science and Technology, Tokyo Institute of Technology, 2-12-1 Ookayama, Meguro-ku, Tokyo 152-8552, Japan
Abstract
We report on the synthesis of an acyl phosphatidylinositol dimannoside using the 2-(azidomethyl)benzoate-protected mannosyl donors. An equatorial-orientated C6 hydroxyl group in inositol exhibited higher reactivity towards glycosylation than the axial-orientated C2 hydroxyl group. Sequential glycosylation of the C6 and C2 hydroxyls with two different thioglycosides allowed the synthesis of a 2,6-di-O-α-mannosyl inositol in one-pot. After incorporation of a fatty acid and an acyl phosphatidyl moiety, all protecting groups involving benzyl ethers, benzylidene acetal and the 2-(azidomethyl)benzoate were removed under hydrogenolysis to provide an acyl phosphatidylinositol dimannoside.Phosphatidylinositol mannosides (PIMs) are components of the mycobacterial cell wall envelope and exert both immunosuppressive and adjuvantive activities.1 PIMs comprised of a 2,6-di-O-α-mannosyl inositol and contain a phosphatidyl group at the C1 hydroxyl group as a core unit are a diverse class of compounds, since they also can be modified with fatty acids and oligomannans.2 In addition, the length of the fatty acids can also vary. To elucidate the structure-activity relationships of PIMs, structurally defined and pure PIM derivatives are needed. However, the possibility that such isolated glycolipids could be heterogeneous and/or contaminated with antigenic compounds cannot be excluded. Therefore, the availability of chemically pure synthesized PIMs as biochemical probes would be highly desirable.3 Most of the established methods for the synthesis of PIM related compounds involves 1) construction of the oligomannosyl inositol, 2) incorporation of the acyl phosphatidyl moiety on the saccharide part. Acyl protecting groups at the C2 hydroxyl group of the mannosyl donors provide us a reliable α-mannosylation. However, it is difficult to selective remove of the acyl protecting groups without decomposition of the fatty acyl moieties. Therefore, the acyl protecting groups were replaced to other protecting groups such as a benzyl ether before installation of the phosphatidyl moiety and fatty acids.
On the other hand, a 2-(azidomethyl)benzoate (AZMB) is a protecting group of a hydroxyl group, which was developed by Sekine and coworkers.4 It can be chemoselectively removed in the presence of ester protecting groups by lactamization initiated from the reduction of the azido group.5 Seeberger and coworkers demonstrated the AZMB ester as an orthogonal and participating C2 protecting group in the synthesis of a protected H-type II pentasaccharide.6 Protection of the mannosyl donor with the AZMB ester would enable to omit the protecting group manipulation and improves an efficiency of the synthesis of the PIM derivatives. Herein we report on an efficient synthesis of the acyl phosphatidylinositol dimannoside 1 using 2-(azidomethyl)benzoate as an O2 protecting group of a mannosyl donor.
The strategy for the synthesis of the acyl phosphatidylinositol dimannoside (PIM2) 1 is shown in Scheme 1. We designed the dimannosyl inositol 2, which contains a 2-naphthylmethyl (NAP) ether and a chloroacetyl ester as a key intermediate. The protecting groups are chemoselectively removable to permit the introduction of a phosphatidyl moiety 4 and the fatty acid 3, respectively. The given protected acyl PIM2 can be directly subjected to deprotection by hydrogenolysis to obtain the acyl PIM2 1. The inositol dimannoside 2 can be prepared from the inositol diol acceptor 5 and the two mannosyl donors 6 and 7 possessing the AZMB ester. According to the reported literature, the equatorial hydroxyl group at the 6 position is more reactive towards glycosylation with 2-acyl mannosyl donors than the axial-orientated one at the 2 position.7 We also examined the synthesis of the inositol dimannoside 2 by a one-pot two-step
glycosylation reaction using the three building blocks 5, 6 and 7. The one-pot glycosylation procedure would be applicable to the synthesis of various diglycosylated inositols.8,9
The synthesis of the dimannosyl inositol 2 is shown in Scheme 2. Treatment of the glycosyl donor 6 and 1.2 equivalent of the diol acceptor 510 with N-iodosuccinimide (NIS) and a catalytic amount of TfOH at -10 oC provided the inositol mannoside 8 in 93% yield with complete α-selectivity as a single isomer. The remaining hydroxyl group was determined by a 1H NMR analysis of the acetylated product 9 from 8 to be located at the C2 position of the inositol. The second glycosylation of inositol 8 at the C2 position with mannoside 7 was also examined (Table 1). Treatment of inositol 8 and 1.2 equivalent of glycosyl donor 7 with NIS and a catalytic amount of TfOH at -10 oC provided the inositol dimannoside 2 in 33% yield with complete α-selectivity as a single isomer. The higher initial reaction temperature resulted in the decomposition of the donor 7. Finally, we found that treatment of the acceptor 8 and 1.5 equivalents of the donor 7 with NIS and a catalytic amount of TfOH at -78 oC, followed by a gradual increase in the reaction temperature to 0 oC resulted in the formation of the inositol dimannoside 2 in 79% yield.
Next, the one-pot synthesis of the pseudotrisaccharide 2 from the three building blocks, namely, 5, 6 and 7 was examined. A mixture of the inositol 5 and the thioglycoside 6 was treated with NIS and a catalytic amount of TfOH, initially at -78 oC and then gradually increased to 0 oC. After the first glycosylation was complete, the reaction mixture was cooled to -78 oC. To this reaction mixture, the second donor 7 and NIS were added at the same temperature. It should be noted that in the one-pot glycosylation procedure, the
second glycosylation proceeded smoothly at -78 oC to provide the dimannosyl inositol 2 in 88% yield based on 5.
The synthesis of the phosphatidylinositol dimannoside 1 from the dimannosyl inositol 2 is shown in Scheme 3. Treatment of the dimannosyl inositol 2 with thiourea and 2,6-lutidine at 50 oC provided the alcohol 10 in 94% yield. The resulting alcohol 10 was acylated by treatment with stearoyl chloride (3) under basic conditions to provide the stearate 11 in 97% yield. The following procedure was used to introduce the diacyl phosphatidyl unit 4 at the C1 position of the inositol. DDQ was used as the oxidant at 0 oC , the deprotection of 2-naphthylmethyl ether afforded the alcohol 12 in 46% yield with the starting material 11 in 47% yield. When the reaction was run at room temperature, alcohol 12 was obtained in 33% yield with no recovery of the starting material 11. The use of ceric ammonium nitrate (CAN) as the oxidant resulted in the formation of a complex mixture. The resulting alcohol was acylated with the H-phosphonate 411 followed by oxidation with I2, giving the phosphate 13 in 90% yield. Finally, the phosphate 13 was completely deprotected by palladium-catalyzed hydrogenolysis, giving the acyl PIM2 1 in 82% yield. Phosphatidylinositol mannosides are known to exhibit very low solubility in various solvents. We first examined a NMR experiment using various rates of mixture of solvents (CDCl3:CD3OD:D2O), which was reported for analysis of the related compounds.3l However, no sharp NMR spectra of the acyl PIM2 1 was obtained. 1H NMR spectra of the product at 60 oC in DMSO-d6 indicated deprotection of the all protecting groups involving an aromatic ring.
In conclusion, we describe the synthesis of an acyl phosphatidylinositol dimannoside 1 using the 2-(azidomethyl)benzoate-protected mannosyl donors 6 and 7. An equatorial-orientated C6 hydroxyl group in inositol exhibited higher reactivity towards glycosylation with the AZMB protected donor 6 than the axial-orientated C2 hydroxyl group. Sequential glycosylation of the C6 and C2 hydroxyls with two different thioglycosides 6 and 7 allowed the synthesis of a 2,6-di-α-mannosyl inositol in one-pot. After incorporation of a fatty acid and an acyl phosphatidyl moiety, all protecting groups involving benzyl ethers, benzylidene acetal and the 2-(azidomethyl)benzoate were simultaneously removed under hydrogenolysis to provide a phosphatidylinositol dimannoside 1. This approach would be effective for the synthesis of various PIMs related compounds.
EXPERIMENTAL
General
NMR spectra were recorded on a JEOL Model ECP-400 (400 MHz for 1H, 100 MHz for 13C) and Bruker ADVANCE-600 spectrometer (600 MHz for 1H) instrument in the indicated solvent. Chemical shifts are reported in units parts per million (ppm) relative to the signal (0.00 ppm) for internal tetramethylsilane for solutions in CDCl3. 1H NMR spectra data are reported as follows: CDCl3 (7.26 ppm) or DMSO-d6 (2.50 ppm). 13C NMR spectra data are reported relative to CDCl3 (77.0 ppm). 31P NMR spectra data are reported relative to H3PO4 (0.00 ppm) as external standard. Multiplicities are reported by using the following abbreviations: s; singlet, d; doublet, t; triplet, q; quartet, m; multiplet, br; broad, J; coupling constants in Hertz. IR spectra was recorded on a Perkin-Elmer Spectra One FT-IR spectrophotometer. Only the strongest and/or structurally important peaks are reported as the IR data given in cm-1. Optical rotation was measured on a JASCO model P-1020 polarimeter. All reactions were monitored by thin-layer chromatography carried out on 0.2 mm E. Merck silica gel plates (60F-254) with UV light, visualized by 10% ethanolic phosphomolybdic acid, p-anis aldehyde solution or 0.5% ninhydrin n-butanol solution. Daiso silica gel or Merck silica gel was used for a column chromatography. ESI-TOF Mass spectra were measured with P.E. Biosystems TK-3500 Biospectrometry Workstation mass spectrometers and Waters LCT PremierTM XE. HRMS(ESI-TOF) were calibrated with angiotensin Ⅰ (SIGMA), bradykinin (SIGMA), and neurotensin (SIGMA) as an internal standard.
3,4,5-Tri-O-benzyl-1-O-naphthylmethyl-D-myo-inositol (5)
To a stirred solution of 3,4,5-tri-O-benzyl-D-myo-inositol (500 mg, 1.11 mmol) in dry toluene (16.7 mL) was added dibutylstannanone (304 mg, 1.22 mmol) at room temperature. After being stirred at 140 ˚C for 5 h, 2-(bromomethyl)naphthalene (368 mg, 1.66 mmol), cesium fluoride (253 mg, 1.66 mmol) and a catalytic amount of TBAI were added to the reaction mixture at 80 ˚C. After being stirred at the same temperature for 10 h, the reaction mixture was poured into ice-cooled saturated aq. NaHCO3. The aqeous layer was extracted with two portions of EtOAc. The combined extract was washed with 1 M HCl, saturated aq. NaHCO3 and brine, dried over MgSO4, filtered and evaporated in vacuo. The residue was purified by a column chromatography on silica gel with 70:30 hexane:EtOAc to give the naphthylmethyl ether 5 (511 mg, 0.865 mmol, 78%). [α]D21 -1.65 (c 0.330, CHCl3); 1H NMR (400 MHz, CDCl3) δ 7.90-7.70 (4H, m), 7.55-7.40 (3H, m), 7.40-7.20 (15H, m), 4.93-4.78 (6H, m), 4.67 (2H, s), 4.23 (1H, br-t, J = 2.7 Hz), 4.10 (1H, dt, J = 9.7, 2.0 Hz), 3.97 (1H, t, J= 9.7 Hz), 3.38 (1H, dd, J = 9.2, 2.4 Hz), 3.32 (1H, t, J = 9.7 Hz), 3.27 (1H, dd, J = 9.7, 2.9 Hz), 2.51 (1H, d, J = 2.0 Hz), 2.48 (1H, s); 13C NMR (100 MHz, CDCl3) δ 138.7, 137.8, 135.2, 133.2, 133.1, 128.4, 128.3, 127.9, 127.7, 127.5, 126.7, 126.2, 126.1, 125.8, 82.9, 80.9, 79.9, 78.9, 75.8, 75.4, 72.6, 72.5, 67.1; IR (KBr) ν max = 3444, 2864, 1602, 1496, 1453, 1360, 1119, 1086, 1069, 1027 (cm-1); HRMS (ESI-TOF) Calcd for C38H39O6 [M+H]+ 591.2747, found 591.2748.
6-O-(2-O-(2’-Azidomethylbenzoyl)-3-O-benzyl-4,6-O-benzylidene-α-D-mannopyranosyl)-3,4,5-tri-O-benzyl-1-O-naphthylmethyl-D-myo-inositol (8)
A mixture of the naphthylmethyl ether 5 (38.9 mg, 65.9 μmol) and pulverized activated MS4A (55.0 mg) in dry CH2Cl2 (1.10 mL) was stirred at room temperature for 1 h under argon to remove a trace amount of water. Then the reaction mixture was cooled to -10 ˚C. N-Iodosuccinimide (16.1 mg, 71.3 μmol) and a catalytic amount of trifluoromethanesulfonic acid (0.500 μL, 5.49 μmol) was added at the same temperature. After being stirred at the same temperature for 1 h, the reaction mixture was neutralized with triethylamine and filtered through a pad of Celite. The filtrate mixture was poured into a mixture of saturated aq. NaHCO3 and 10% aq. Na2S2O3 with cooling. The aqueous layer was extracted with two portions of EtOAc. The combined extract was washed with saturated aq. NaHCO3 and 10% aq. Na2S2O3 and brine, dried over MgSO4, filtered and evaporated in vacuo. The residue was purified by a column chromatography on silica gel with 80:20 hexane:EtOAc to give the mannoside 8 (55.8 mg, 51.1 μmol, 93%). [α]D21 +12.2 (c 0.515, CHCl3); 1H NMR (400 MHz, CDCl3) δ 7.79-7.70 (5H, m), 7.55-7.05 (31H, m), 5.56 (1H, dd, J = 2.9, 1.5 Hz), 5.51 (1H, s), 5.47 (1H, d, J = 1.5 Hz), 4.93-4.58 (12H, m), 4.26-3.88 (10H, m), 3.64 (1H, t, J = 10.1 Hz), 3.39 (1H, dd, J = 9.7, 2.9 Hz), 3.36-3.26 (2H, m), 2.44 (1H, s); 13C NMR (100 MHz, CDCl3) δ 166.6, 138.5, 137.8, 137.7, 134.4, 133.2, 133.1, 128.7, 128.6, 128.5, 128.3, 127.9, 127.8x2, 127.7, 127.6, 127.5, 127.4, 127.2, 126.3x2, 126.1, (101.4, 98.5, anomeric, benzylidene), 81.4, 80.7, 80.3, 79.4, 78.2, 75.8, 75.7, 75.2, 73.5, 72.6, 72.2, 72.0, 71.6, 68.5, 66.3, 63.9, 40.8; IR (KBr) ν max =3418, 2924, 2865, 2099, 1718, 1496, 1452, 1353, 1254, 1128, 1061, 1032 (cm-1); HRMS (ESI-TOF) Calcd for C66H64N3O12 [M+H]+ 1090.4490, found 1090.4459.
2-O-Acetyl-6-O-(2-O-(2’-azidomethylbenzoyl)-3-O-benzyl-4,6-O-benzylidene-α-D-mannopyranosyl)-3,4,5-tri-O-benzyl-1-O-naphthylmethyl-D-myo-inositol (9)
To a stirred solution of the mannoside 8 (6.00 mg, 5.50 μmol) in dry CH2Cl2 (1.00 mL) and dry pyridine (0.890 μL, 11.0 μmol) was added acetic anhydride (1.00 μL, 6.60 μmol) at 0 ˚C. After being stirred at room temperature for 2 h, the reaction mixture was poured into ice-cooled 1 M HCl. The aqueous layer was extracted with two portions of EtOAc. The combined extract was washed with saturated aq. NaHCO3 and brine, dried over MgSO4 and evaporated in vacuo. The residue was purified by a column chromatography on silica gel with 85:15 hexane:EtOAc to give the acetate 9 (6.1 mg, 5.39 μmol, 98%). [α]D21 +5.67 (c 0.105, CDCl3); 1H NMR (400 MHz, CDCl3) δ 7.92-7.80 (3H, m), 7.73 (1H, s), 7.60-7.03 (32H, m), 5.89 (1H, br-t, J = 2.4 Hz), 5.58 (1H, dd, J = 3.0, 1.4 Hz), 5.51 (1H, s), 5.43 (1H, br-d, J = 1.4 Hz), 4.95-4.49 (11H, m), 4.16-3.85 (8H, m), 3.64 (1H, t, J = 10.1, 9.7 Hz), 3.42-3.50 (2H, m), 3.35 (1H, t, J = 9.7 Hz), 2.19 (3H, s); IR (KBr) ν max = 3450, 2924, 2855, 2102, 1744, 1723, 1497, 1454, 1372, 1259, 1233, 1259, 1090, 1028 (cm-1); HRMS (ESI-TOF) Calcd for C68H69N4O13 [M+NH4]+ 1149.4861, found 1149.4867.
2-O-(2’-Azidomethylbenzoyl)-3,4-di-O-benzyl-6-O-chloroacetyl-α-D-mannopyranosyl)-6-O-(2-O-(2’-azidomethylbenzoyl)-3-O-benzyl-4,6-O-benzylidene-α-D-mannopyranosyl)-2-O-(3,4,5-tri-O-benzyl-1-O-naphthylmethyl-D-myo-inositol (2)
A mixture of the mannoside 7 (172 mg, 0.250 mmol), the inositol 8 (182 mg, 0.167 mmol) and pulverized activated MS4A (167 mg) in dry CH2Cl2 (3.34 mL) was stirred at room temperature for 1 h under argon to remove a trace amount of water. Then the reaction mixture was cooled to -78 ˚C. N-Iodosuccinimide (75.0 mg, 0.333 mmol) and a catalytic amount of trifluoromethanesulfonic acid (1.48 μL, 16.7 μmol) was added at the same temperature. After being stirred at the same temperature for 6 h with being allowed to 0 ˚C, the reaction mixture was neutralized with triethylamine and filtered through a pad of Celite. The filtrate mixture was poured into a mixture of saturated aq. NaHCO3 and 10% aq. Na2S2O3 with cooling. The aqueous layer was extracted with two portions of EtOAc. The combined extract was washed with saturated aq. NaHCO3 and10% aq. Na2S2O3 and brine, dried over MgSO4, filtered and evaporated in vacuo. The residue was purified by a column chromatography on silica gel with 85:15 hexane:EtOAc to give the inositol dimannoside 2 (220 mg, 0.131 mmol, 79%). [α]D21 +7.66 (c 1.00, CDCl3); 1H NMR (400 MHz, CDCl3) δ 7.86 (1H, d, J = 7.7 Hz), 7.79 (1H, d, J = 7.2 Hz), 7.72-7.00 (48H, m), 5.75-5.71 (3H, br-s), 5.58 (1H, s), 5.21 (1H, br-s), 4.96-4.25 (18H, m), 4.32 (1H, br-s), 4.30-4.20 (3H, m), 4.17-4.04 (5H, m), 3.93 (1H, br-d, J = 10.1 Hz), 3.92-3.80 (4H, m), 3.76 (1H, t, J = 10.1, 9.2 Hz), 3.49 (1H, dd, J = 9.2, 2.4 Hz), 3.41 (1H, t, J = 9.7 Hz), 3.35 (1H, dd, J = 9.7, 2.9 Hz); 13C NMR (100 MHz, CDCl3) δ 166.7, 165.4, 165.0, 138.3, 138.0, 137.8, 137.7, 137.6, 137.5, 137.4, 137.3, 134.2, 133.0, 132.7, 132.6, 131.3, 131.2, 129.2, 129.1, 129.0, 128.9, 128.7, 128.6, 128.4, 128.3, 128.2, 128.1, 127.9, 127.8, 127.6, 127.5, 127.4, 127.2, 126.3, 126.1, 126.0, 125.9, 125.2, 101.4, 99.3, 98.8, 81.4, 81.2, 81.0, 78.7, 78.4, 77.2, 76.1, 76.0, 75.7, 75.1, 74.8, 73.7, 73.6, 72.7, 72.6, 72.5, 72.0, 71.9, 71.4, 71.3, 70.2, 69.5, 68.7, 64.3, 64.2, 52.7, 52.6, 40.5, 29.6; IR (KBr) ν max = 3450, 3064, 3032, 2929, 2872, 2102, 1722, 1454, 1289, 1257, 1129, 1087 (cm-1); HRMS (ESI-TOF) Calcd for C96H95N7O19Cl [M+NH4]+ 1684.6371, found 1684.6360.
One-pot glycosylation
A mixture of the mannosyl donor 6 (460 mg, 0.755 mmol), the inositol acceptor 5 (446 mg, 0.755 mmol) and pulverized activated MS4A (755 mg, 1.0 g/mmol) in dry CH2Cl2 (11.3 mL) was stirred at room temperature for 1 h under argon to remove a trace amount of water. Then the reaction mixture was cooled to -78 ˚C. N-Iodosuccinimide (221 mg, 0.982 mmol) and a catalytic amount of trifluoromethanesulfonic acid (6.72 μL, 75.5 μmol) were added at the same temperature. After being stirred at the same temperature for 3 h, the reaction mixture was warmed to 0 ˚C. After being stirred for 30 min, the reaction mixture was cooled to -78 ˚C. After 5 min, a solution of the second mannosyl donor 7 (779 mg, 1.13 mmol) in dry CH2Cl2 (3.78 mL) and N-iodosuccinimide (340 mg, 1.51 mmol) was added to the reaction mixture. After being stirred at the same temperature for 2 h, the reaction mixture was neutralized with triethylamine and filtered through a pad of Celite. The filtrate mixture was poured into a mixture of saturated aq. NaHCO3 and 10% aq. Na2S2O3 with cooling. The aqueous layer was extracted with two portions of EtOAc. The combined extract was washed with saturated aq. NaHCO3 and10% aq. Na2S2O3 and brine, dried over MgSO4, filtered and evaporated in vacuo. The residue was purified by a column chromatography on silica gel with 85:15 hexane:EtOAc to give the inositol dimannoside 2 (1.18 g, 0.664 mmol, 88% based on 5).
2-O-(2-O-(2’-Azidomethylbenzoyl)-3,4-di-O-benzyl-α-D-mannopyranosyl)-6-O-(2-O-(2’-azidomethyl-benzoyl)-3-O-benzyl-4,6-O-benzylidene-α-D-mannopyranosyl)-3,4,5-tri-O-benzyl-1-O-naphthylmethyl-D-myo-inositol (10)
To a stirred solution of the inositol dimannoside 2 (220 mg, 0.132 mmol) in dry DMF (1.32 mL) and dry 2,6-lutidine (23.5 μL, 0.158 mmol) was added thiourea (15.0 mg, 0.197 mmol) at room temperature. After being stirred at 50 ˚C for 10 h, the reaction mixture was poured into ice-cooled 1 M HCl. The aqueous layer was extracted with two portions of EtOAc. The combined extract was washed with saturated aq. NaHCO3 and brine, dried over MgSO4, filtered and evaporated in vacuo. The residue was purified by a column chromatography on silica gel with 70:30 hexane:EtOAc to give the alcohol 10 (196 mg, 0.123 mmol, 94%). [α]D21 -2.46 (c 2.35, CDCl3); 1H NMR (400 MHz, CDCl3) δ 7.88 (1H, d, J = 7.7 Hz), 7.81 (1H, d, J = 7.7 Hz), 7.70-7.00 (48H, m), 5.74-5.68 (3H, m), 5.58 (1H, s), 5.21 (1H, s), 4.94-4.26 (18H, m), 4.24 (1H, br-s), 4.26-4.18 (3H, m), 4.15-4.04 (4H, m), 3.94-3.84 (3H, m), 3.75 (1H, t, J = 10.1 Hz); 13C NMR (100 MHz, CDCl3) δ 165.4, 165.3, 138.4, 138.3, 138.0, 137.8, 137.7, 137.5, 137.4, 137.0, 134.4, 133.0, 132.8, 132.7, 131.4, 131.3, 129.8, 129.3, 129.2, 128.7, 128.5, 128.4, 128.3, 128.2, 128.1, 127.9, 127.8, 127.7, 127.6, 127.5, 126.3, 126.0, 125.3, 101.4, 99.3, 99.0, 81.4, 81.3, 81.0, 78.7, 78.4, 77.2, 76.2, 76.0, 75.7, 75.2, 75.1, 73.8, 73.7, 73.6, 72.7, 72.6, 72.4, 72.0, 71.5, 70.3, 69.3, 68.7, 64.2, 61.7, 52.8, 52.7, 52.5; IR (KBr) ν max = 3477, 3064, 3032, 2872, 2925, 2103, 1721, 1497, 1454, 1366, 1258, 1167, 1086, 1028 (cm-1); HRMS (ESI-TOF) Calcd for C94H94N7O18 [M+NH4]+ 1608.6655, found 1608.6665.
2-O-(2-O-(2’-Azidomethylbenzoyl)-3,4-di-O-benzyl-6-O-stearoyl-α-D-mannopyranosyl)-6-O-(2-O-(2’-azidomethylbenzoyl)-3-O-benzyl-4,6-O-benzylidene-α-D-mannopyranosyl)-3,4,5-tri-O-benzyl-1-O-naphthylmethyl-D-myo-inositol (11)
To a stirred solution of the alcohol (57.0 mg, 35.8 μmol) in dry CH2Cl2 (1.00 mL) and dry pyridine (8.69 μL, 0.107 mmol) was added stearoyl chloride (22.4 mg, 71.6 μmol) and a catalytic amount of DMAP at 0 ˚C. After being stirred at room temperature for 1 h, the reaction mixture was poured into ice-cooled 1 M HCl. The aqueous layer was extracted with two portions of EtOAc. The combined extract was washed with saturated aq. NaHCO3 and brine, dried over MgSO4, filtered and evaporated in vacuo. The residue was purified by a column chromatography on silica gel with 85:15 hexane:EtOAc to give the stearoyl ester 11 (64.3 mg, 34.6 μmol, 97%). [α]D21 -10.3 (c 1.05, CHCl3); 1H NMR (400 MHz, CDCl3) δ 7.86 (1H, d, J = 7.7 Hz), 7.80 (1H, d, J = 7.7 Hz), 7.73-7.11 (48H, m), 5.75-5.70 (3H, m), 5.58 (1H, s), 5.25 (1H, br-d, J = 1.4 Hz), 4.94-4.38 (18H, m), 4.34 (1H, br-s), 4.30-4.19 (3H, m), 4.17-4.04 (5H, m), 3.98 (1H, br-d, J = 10.1 Hz), 3.92-3.80 (2H, m), 3.75 (1H, t, J = 10.1 Hz), 3.46 (1H, dd, J = 9.2, 2.4 Hz), 3.40 (1H, t, J = 9.7 Hz), 3.33 (1H, dd, J = 9.7, 2.9 Hz), 2.19 (2H, t, J = 7.7 Hz), 1.29-1.14 (30H, m), 0.85 (3H, t, J = 7.2 Hz); 13C NMR (100 MHz, CDCl3) δ 173.3, 165.4, 165.0, 138.4, 138.1, 138.0, 137.7, 137.6, 137.5, 137.4, 134.2, 133.0, 132.7, 131.3, 131.2, 129.2, 129.1, 128.9, 128.7, 128.4x2, 128.3x2, 128.2, 128.1, 127.9x2, 127.8x2, 127.6, 127.5x2, 127.4, 126.3, 126.0, 125.9, 125.2, (101.4, 99.3, 98.9, anomeric, benzylidene), 81.4, 81.2, 81.0, 78.7, 78.2, 77.2, 76.1, 76.0, 75.7, 75.1, 73.8, 73.6, 73.5, 72.4, 72.0, 71.4, 71.3, 70.3, 70.0, 68.8, 68.7, 64.2, 62.7, 52.7, 52.6, 34.0, 31.8, 29.7, 29.4, 29.3, 29.2x2, 29.0, 24.8, 22.6, 14.1; IR (KBr) ν max = 3065, 2920, 2854, 2103, 1722, 1500, 1257, 1089, 736, 698; HRMS (ESI-TOF) Calcd for C112H128N7O19 [M+NH4]+ 1874.9265, found 1874.9259.
2-O-(2-O-(2’-Azidomethylbenzoyl)-3,4-di-O-benzyl-6-O-stearoyl-α-D-mannopyranosyl)-6-O-(2-O-(2’-azidomethylbenzoyl)-3-O-benzyl-4,6-O-benzylidene-α-D-mannopyranosyl)-3,4,5-tri-O-benzyl-D-myo-inositol (12)
To a stirred solution of the naphthylmethyl ether 11 (60.0 mg, 32.3 μmol) in dry CH2Cl2 (2.00 mL) and saturated aq. NaHCO3 (600 μL) was added an excess amount of DDQ at 0 ˚C. After being stirred at the same temperature for 5 h, the reaction mixture was poured into ice-cooled saturated aq. NaHCO3. The aqueous layer was extracted with two portions of CH2Cl2. The combined extract was washed with saturated aq. NaHCO3 and brine, dried over MgSO4, filtered and evaporated in vacuo. The residue was purified by a column chromatography on silica gel with 80:20 hexane:EtOAc to give the alcohol 12 (26.0 mg, 15.1 μmol, 46%). [α]D21 +10.4 (c 0.365, CHCl3); 1H NMR (400 MHz, CDCl3) δ 8.08 (1H, dd, J = 7.8, 1.4 Hz), 8.03 (1H, dd, J = 8.3, 1.0 Hz), 7.60-7.16 (41H, m), 5.69 (1H, br-t, J = 2.4, 2.0 Hz), 5.62 (1H, s), 5.60 (1H, dd, J = 3.0, 1.5 Hz), 5.57 (1H, br-d, J = 1.5 Hz), 5.40 (1H, br-d, J = 1.5 Hz), 4.60-4.95 (15H, m), 4.53 (1H, d, J = 10.7 Hz), 4.31-4.20 (2H, m), 4.20-3.96 (7H, m), 3.92 (1H, t, J = 9.8 Hz), 3.86 (1H, t, J = 9.8, 9.3 Hz), 3.74 (1H, t, J = 9.8 Hz), 3.70-3.63 (1H, m), 3.43-3.35 (2H, m), 2.54 (1H, br-d, J = 5.4 Hz), 2.25-2.17 (2H, m), 1.65-1.50 (2H, m), 1.35-1.12 (28H, m), 0.88 (3H, t, J = 6.8 Hz); IR (KBr) ν max = 3428, 2925, 2854, 2104, 1723, 1454, 1259, 1086 (cm-1); HRMS (ESI-TOF) Calcd for C101H120N7O19 [M+NH4]+ 1734.8639, found 1734.8610.
2-O-(2-O-(2’-Azidomethylbenzoyl)-3,4-di-O-benzyl-6-O-stearoyl-α-D-mannopyranosyl)-6-O-(2-O-(2’-azidomethylbenzoyl)-3-O-benzyl-4,6-O-benzylidene-α-D-mannopyranosyl)-3,4,5-tri-O-benzyl-1-O-(1,2-di-O-stearoyl-sn-3-O-glycerylphosphoryl)-D-myo-inositol (13)
A mixture of the alcohol 12 (12.6 mg, 7.33 μmol) and the H-phosphonate 4 (25.2 mg, 36.7 μmol) was coevaporated with dry pyridine and dried under a high vacuum overnight. To the above mixture in dry CH2Cl2 (1.00 mL) and dry pyridine (147 μL) was added pivaloyl chloride (8.93 μL, 73.3 μmol) at room temperature. After being stirred at the same temperature for 3 h, a solution of iodine (9.31 mg, 36.7 μmol) in a mixture of pyridine/water (19:1, 1.00 mL) was added to oxidize P(III) to P(V). After being stirred for 2 h, the reaction mixture was then diluted with CHCl3 and washed with 10% aqueous Na2S2O3. The aqueous layer was extracted with two portions of CHCl3. The combined extract was washed with water and dried over Na2SO4, filtered and evaporated in vacuo. The residue was purified by a column chromatography on NEt3-treated silica gel with 98:2 CHCl3:MeOH to give the phosphoryl ester 13 (15.9 mg, 6.59 μmol, 90%). [α]D21 +7.70 (c 0.405, CHCl3); 1H NMR (400 MHz, CDCl3) δ 8.06 (1H, d, J = 7.7 Hz), 8.02 (1H, d, J = 7.3 Hz), 7.60-7.07 (41H, m), 5.90 (1H, br-t, J = 1.5, 1.4 Hz), 5.83 (1H, br-t, J = 2.4, 2.0 Hz), 5.68 (1H, br-s), 5.58 (1H, s), 5.53 (1H, br-s), 5.28-5.19 (1H, br-m), 4.94-4.56 (15H, m), 4.80 (1H, br-s), 4.46 (1H, d, J = 10.6 Hz), 4.38-4.16 (7H, m), 4.13 (1H, dd, J = 9.2, 2.4 Hz), 4.12-4.04 (1H, m), 4.04-3.94 (3H, m), 3.90-3.74 (5H, m), 3.54-3.42 (2H, m), 2.88 (6H, q, J = 7.2 Hz), 2.22-2.10 (6H, m), 1.56-1.44 (6H, m), 1.34-1.12 (84H, m), 1.10 (9H, t, J = 7.2 Hz), 0.88 (9H, t, J = 7.2 Hz); 13C NMR (100 MHz, CDCl3) δ 167.3, 138.6, 138.1, 137.9, 137.8, 137.7, 137.5x2, 137.2, 137.0, 135.1, 132.6, 131.2, 129.3, 129.2, 129.0, 128.7, 128.6, 128.5, 128.4x2, 128.3x2, 128.2x2, 128.1, 128.0x3, 127.9x3, 127.8x2, 127.7x2, 127.6x2, 127.5, 127.3, 126.9, 126.4, 126.3, 126.2, 126.1, 126.0, (101.5, 99.1, 98.6, anomeric, benzylidene), 81.3, 81.0, 80.8, 78.9, 78.7, 78.2, 77.8, 75.5, 75.2, 73.8, 73.7, 72.1, 71.9, 70.7, 68.3, 67.2, 63.8, 52.6, 40.8, 29.7; 31P NMR (100 MHz, CDCl3) δ -0.26; IR (KBr) ν max = 3429, 2925, 2854, 2104, 1725, 1455, 1255, 1088, 735, 697 (cm-1).
6-O-(α-D-Mannopyranosyl)-2-O-(6-O-stearoyl-α-D-mannopyranosyl)-1-O-(1,2-di-O-stearoyl-sn-3-O-glycerylphosphoryl)-D-myo-inositol sodium salt (AcPIM2) (1)
To a stirred solution of the phosphoryl ester 13 (16.7 mg, 6.68 μmol) in CH2Cl2 (500 μL) and MeOH (500 μL) was added DoweX cation exchange resin Na form at room temperature. After being stirred at the same temperature for 5 h, the reaction mixture was filtered and evaporated in vacuo. The residue was used for the next reaction without further purification. The above residue in EtOAc:THF:EtOH:H2O (2.00 mL, 2:1:1:1) was added Pd/C (1.00 mg). The reaction mixture was hydrogenolyzed for 10 h under H2 gas atmosphere. The reaction mixture was filtered through a pad of Celite and evaporated in vacuo. The residue was purified by Iatrobeads 6RS-8030 with 40:20:20:20 EtOAc:THF:EtOH:H2O to give AcPIM2 1 (8.0 mg, 5.47 μmol, 2 steps 82%). 1H NMR was measured after conversion of triethylammonium salt. 1H NMR (600 MHz, DMSO-d6) δ 5.13-5.05 (2H, m), 4.90 (1H, d, J = 2.4 Hz), 4.57-3.95 (7H, m), 3.86-3.38 (13H, m), 3.22-3.16 (2H, m), 3.10-3.02 (6H, m), 2.31-2.22 (4H, m), 1.57-1.48 (4H, m), 1.32-1.19 (93H, m), 0.85 (9H, t, J = 6.8 Hz), 31P NMR (100 MHz, DMSO-d6) δ -0.47.
ACKNOWLEDGEMENTS
This work was supported by a Grant-in-Aid for Scientific Research (B) (Grant-in-aid No. 23310150) from the Ministry of Education, Culture, Sports, Science, and Technology.
References
1. (a) P. J. Brennan, Tuberculosis, 2003, 83, 91; CrossRef (b) V. J. Quesniaux, M. D. Nicolle, D. Torres, L. Kremer, Y. Guérardel, J. Nigou, G. Puzo, F. Erard, and B. Ryffel, J. Immunol., 2004, 172, 4425; (c) K. Fischer, E. Scotet, M. Niemeyer, H. Koebernick, J. Zerrahn, S. Maillet, R. Hurwitz, M. Kursar, M. Bonneville, S. H. E. Kaufmann, and U. E. Schaible, Proc. Natl. Acad. Sci. USA, 2004, 101, 10685. CrossRef
2. (a) J. B. Torrelles, A. K. Azad, and L. S. Schlesinger, J. Immunol., 2006, 177, 1805; (b) G. D. Sprott, C. J. Dicaire, K. Gurnani, S. Sad, and L. Krishnan, Infect. Immun., 2004, 72, 5235; CrossRef (c) M. Gilleron, J. Nigou, D. Nicolle, V. Quesniaux, and G. Puzo, Chem. Biol., 2006, 13, 39. CrossRef
3. (a) C. J. J. Elie, R. Verduyn, C. E. Dreef, D. M. Brounts, G. A. Van der Marel, and J. H. Van Boom, Tetrahedron, 1990, 46, 8243; CrossRef (b) Y. Watanabe, T. Yamamoto, and T. Okazaki, Tetrahedron, 1997, 53, 903; CrossRef (c) A. Stadelmaier and R. R. Schmidt, Carbohydr. Res., 2003, 338, 2557; CrossRef (d) K. N. Jayaprakash, J. Lu, and B. Fraser-Reid, Bioorg. Med. Chem. Lett., 2004, 14, 3815; CrossRef (e) A. Stadelmaier, M. B. Biskup, and R. R. Schmidt, Eur. J. Org. Chem., 2004, 3292; CrossRef (f) X. Liu, B. L. Stocker, and P. H. Seeberger, J. Am. Chem. Soc., 2006, 128, 3638; (g) G. D. Ainge, H. Davis, G. S. Larsen, G. F. Painter, G. S. Gill, and J. L. Harper, Bioorg. Med. Chem., 2006, 14, 5632; CrossRef (h) G. D. Ainge, N. A. Parlane, M. Denis, C. M. Hayman, D. S. Larsen, and G. F. Painter, Bioorg. Med. Chem., 2006, 14, 7615; CrossRef (i) B. Fraser-Reid, S. R. Chaudhuri, K. L. Jayaprakash, J. Lu, and C. V. S. Ramamurty, J. Org. Chem., 2008, 73, 9732; CrossRef (j) S. Boonyarattanakalin, X. Liu, M. Michieletti, B. Lepenies, and P. H. Seeberger, J. Am. Chem. Soc., 2008, 130, 16791; CrossRef (k) P. S. Patil and S. C. Hung, Chem. Eur. J., 2009, 15, 1091; CrossRef (l) A. Ali, M. R. Wenk, and M. J. Lear, Tetrahedron Lett., 2009, 50, 5664; CrossRef (m) P. S. Patil and S. C. Hung, Org. Lett., 2010, 12, 2618. CrossRef
4. T. Wada, A. Ohkubo, A. Mochizuki, and M. Sekine, Tetrahedron Lett., 2001, 42, 1061. CrossRef
5. N. J. Osborn and R. J. Robinson, Tetrahedron, 1993, 49, 2878. CrossRef
6. K. R. Love, R. B. Andrade, and P. H. Seeberger, J. Org. Chem., 2001, 66, 8165. CrossRef
7. G. Anilkumar, G. Latha, G. Nair, and B. Fraser-Reid, Org. Lett., 2000, 2, 2587. CrossRef
8. (a) L. Yang, Q. Qin, and X.-S. Ye, Asian J. Org. Chem., 2013, 2, 30; (b) H. Tanaka, H. Yamada, and T. Takahashi, Trend in Glycosci. Glycotech., 2007, 19, 183; CrossRef (c) Y. Wang, X.-S. Ye, and L.-H. Zhang, Org. Biomol. Chem., 2007, 5, 2189. CrossRef
9. (a) H. Yamada, T. Harada, and T. Takahashi, J. Am. Chem. Soc., 1994, 116, 7919; CrossRef (b) H. Tanaka, M. Adachi, H. Tsukamoto, T. Ikeda, H. Yamada, and T. Takahashi, Org. Lett., 2002, 4, 4213; CrossRef (c) H. Tanaka, M. Adachi, and T. Takahashi, Tetrahedron Lett., 2004, 45, 1433; CrossRef (d) M. Adachi, H. Tanaka, and T. Takahashi, Synlett, 2004, 609; CrossRef (e) H. Tanaka, M. Adachi, and T. Takahashi, Chem. Eur. J., 2005, 11, 849; CrossRef (f) H. Tanaka, T. Ishida, N. Matoba, H. Tsukamoto, H. Yamada, and T. Takahashi, Angew. Chem. Int. Ed., 2006, 45, 6349; CrossRef (g) H. Tanaka, Y. Tateno, Y. Nishiura, and T. Takahashi, Org. Lett., 2008, 10, 5597; CrossRef (h) H. Tanaka, Y. Tanimoto, T. Kawai, and T. Takahashi, Tetrahedron, 2011, 67, 10011. CrossRef
10. S. L. Bender and R. J. Budhu, J. Am. Chem. Soc., 1991, 113, 9883. CrossRef
11. (a) B. C. Froehler, P. G. Ng, and M. D. Matteucci, Nucleic Acids Res., 1986, 14, 5399; CrossRef (b) P. J. Garegg, I. Lindh, T. Regberg, J. Stawinski, R. Stronberg, and C. Henrichson, Tetrahedron Lett., 1986, 27, 4051. CrossRef