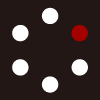
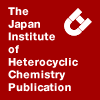
HETEROCYCLES
An International Journal for Reviews and Communications in Heterocyclic ChemistryWeb Edition ISSN: 1881-0942
Published online by The Japan Institute of Heterocyclic Chemistry
e-Journal
Full Text HTML
Received, 2nd July, 2014, Accepted, 29th July, 2014, Published online, 14th August, 2014.
DOI: 10.3987/COM-14-S(K)77
■ Investigation of Pd(II)-Catalyzed Cyclization of Chiral θ-Hydroxy-α,β,γ,δ-unsaturated Dienol
Akiko Ida, Naoyuki Hoshiya, and Jun'ichi Uenishi*
Pharmacetuical Chemistry, Kyoto Pharmaceutical University, 1 Shichono-cho, Misasagi, Yamashina-ku, Kyoto 607-8412, Japan
Abstract
PdCl2(MeCN)2 catalyzed intramolecular cyclization of optically pure θ–hydroxyl-α,β,γ,δ−dienylethanols 1 and 2 are described. cis and trans-2- Benzyloxymethyl-6-(1,3-pentadienyl)tetrahydropyrans 3 and 4 were produced stereoselectively with a ratio of 3:1 in excellent yield. No 1,5-chirality transfer was observed in the cyclization reaction.INTRODUCTION
Substitution in allylic system has been an important area of intensive research in organic synthesis.1 We have reported intramolecular allylic substitution reaction for the formation of saturated oxa-heterocycles using chiral allylic alcohol and Pd(II)-catalyst.2 For example as illustrated in Scheme 1, chiral center on the allylic alcohol is transferred onto the newly generated chiral center on the 2,6-substituted tetrahydropyran ring via an SN2’ reaction process. The stereospecific Pd(II)-catalyzed cyclization of allylic substrate has several advantages including (i) the reaction proceeds cleanly under the mild conditions with high stereoselectivity and excellent chemical yield; (ii) no re-oxidant is required because Pd(0) species are not formed in the catalytic cycle; (iii) hydroxyl group can be used as a leaving group in
the allylic system and water is only a side-product in this reaction. Therefore it is environmentally friendly and used in some natural product syntheses.3
If the intramolecular SN2’ reaction can be applied to an extended conjugate alkenol system, it would be valuable for the stereospecific synthesis of polyenyl substituted oxa-heterocycles by an SN2” reaction. Therefore, we are interested in the possibility of cyclization of chiral dienylethanols 1 and 2 and its stereochemistry. If this intramolecular SN2” reaction proceeds either in syn- or anti-1,5-chirality transfer process, cis or trans 2,6-disubstituted tetrahydropyran 3 or 4 will be form stereospecifically (Scheme 2). Based on this idea, we investigated Pd(II)-catalyzed cyclization of 1 and 2.
The synthesis of the precursors 1 and 2 started with (R)-O-benzylglycidol (Schemes 3 and 4).4 Ring opening of the oxirane ring with butenylmagnesium bromide gave alcohol 6, and successive silylation of 6 with tert-butyl(chloro)dimethylsilane afforded compound 7 in 88% yield in 2 steps. Ozonolysis of terminal alkene gave aldehyde 8 in 88% yield. Formation of dienone with δ-keto-2-pentenephosphoric acid diethyl ester5 by Horner-Wadsworth-Emmons reaction in one step afforded the desired dienone 9 but only in 10% yield. Although the reaction of aldehyde with Horner-Wadsworth-Emmons reagent (EtO)2POCH2CH=CHCO2Et gave dienoic acid ester in good yield,6 that with the corresponding ketone (EtO)2POCH2CH=CRCOCH3 gave no desired dienone product (R=Me)6 or in poor chemical yield (R=H).7 Inseparable diastereomeric mixture of alcohols 10 and 11 was obtained by the reduction of 9 with CeCl3 and NaBH4 in 83% combined yield. We tried to improve the synthesis of 10 and 11 by stepwise reactions. The first Horner-Wadsworth-Emmons reaction of 8 with triethyl phoshonoacetate gave enoic acid ester 12 in 83% yield. However reduction of the ester to the allyl alcohol or enal was unsuccessful to give saturated alcohol 13 with poor yield.
The diastereomers of 10 and 11 were separable by lipase catalyzed kinetic acetylation (Scheme 4). Treatment of 10 and 11 with vinyl acetate in the presence of Candida antarctica lipase gave (R)-acetate 14 in 47% yield and recovery of 10 in 44%. Finally desilylation of 10 with tetrabutylammonium fluoride gave 1 in 90% yield. While, methanolysis of 14 followed by deprotection of silyl ether by the same method for 10 accomplished the synthesis of 2 in 70% yield in 2 steps.
The precursor 1 was subjected to PdCl2(MeCN)2 catalyzed reaction in THF at room temperature to afford a mixture of 3 and 4 with a cis:trans ratio of 3:1 in 78% yield (Scheme 5). Similarly, the reaction of 2 under the same reaction conditions gave a mixture of 3 and 4 with a 3:1 ratio in 89% yield. They were separable by HPLC on silica gel. The conformational analysis by proton NMR revealed that compound 3 is a cis isomer and 4 is a trans isomer. Selected chemical shifts and relations of two axial substituents in NOESY spectra are shown in Scheme 5. Two axial protons next to the ring oxygen atom in 3 appear at 3.62 and 3.88 ppm. Equatorial and axial protons at the same positions in 4 are observed at 3.92 and 4.44 ppm. These chemical shifts are relatively matched with those in similar compounds.2b These structures are also confirmed by the NOESY spectra. NOe relation in 3 is observed between two axial protons and that between the axial proton at 4.44 ppm and the axial methylene protons at 3.42 and 3.52 ppm in 4.
Based on these results, the reaction proceeded non-stereospecific but stereoselective. Therefore, expected 1,5-chirality transfer did not occur. More stable cis isomer was formed favorably in a 3:1 ratio in both cases. Considering the stereochemistry for the formation of 3, a conformer I with diequatorial substituents in the six membered chairlike transition state favorably gives rise to 2,6-cis substituted tetrahydropyran ring (Scheme 6). For the formation of 4, two conformers II and III are possible for the formation of 2,6-trans substituted tetrahydropyran ring. Conformational analysis of 4 suggests that the reaction might proceed through conformer II and lead trans isomer 4.
We propose the overall mechanistic sequence of 1,5-substitution as shown in Scheme 7. In marked contrast to the case of allyl alcohol, the hydroxyl group at θ-position is not able to make a ligand exchange with the coordinate hydroxyl group on Pd-π-complex in the case of dienylethanol. Therefore, the reaction undergoes 6-membered chairlike transition state rather than the pathway in ligand exchange of the nucleophilic hydroxyl group and oxypalladation. However, 1,4-oxypalladation does take place with no interaction of the nucleophilc hydroxyl group with Pd-π-complex and successive syn-elimination of Pd(OH)Cl gives the trans-trans-dienyl substituent on the tetrahydropyran ring. Finally, formation of water by the reaction of Pd(OH)Cl and HCl regenerates PdCl2 in the catalytic cycle.
CONCLUSION
In conclusion, we have found that stereoselective ring closure takes place in the cyclization of dienylethanol substrates 1 and 2. The stereochemistry of cyclization can be explained by a 6-membered chairlike transition state via 1,4-oxypalladation process. It is noted that the hydroxy group of dienyl alcohol can play a good leaving property by the activation of Pd(II)-catalyst and 1,5-substitution occurs stereoselectively in a 3:1 ratio.
EXPERIMENTAL
General. 1H NMR and 13C NMR spectra were measured on JEOL JNM-AL-300 (300 MHz and 75 MHz), spectrometer. Chemical shifts (δ) are reported in parts per million (ppm) relative to the resonance of the solvent or to tetramethylsilane (0.00 ppm) for 1H NMR spectra and for 13C NMR spectra. IR spectra were recorded on a JASCO FT/IR-410 spectrophotometer. UV spectra were measured by a JASCO V-530 spectrometer. Low and high-resolution mass spectra (LRMS and HRMS) were obtained on a JEOL JMS 303HF spectrometer using fast atom bombardment (FAB) ionization. Silica gel (230–400 mesh) was used for flash chromatography. Analytical thin-layer chromatography (TLC) was performed on glass pre-coated with silica gel (0.25 mm thickness). All moisture sensitive reactions were carried out under an argon atmosphere. THF was dried over sodium/benzophenone ketyl, and CH2Cl2 was dried over P2O5, and they were distilled prior to use.
(2S)-1-Benzyloxyhept-6-en-2-ol (6).8 To Mg turning (1.78 g, 73.08 mmol) in Et2O (39 mL) was added dropwise a solution of 4-bromo-1-butene (7.36 mL, 73.08 mmol) in Et2O (32 mL) under an argon atomosphere at 50 °C. After 2 h, the mixture was cooled to room temperature and was added to a suspension of CuBr-Me2S (0.25 g, 1.22 mmol) in THF (39 mL) at –78 °C. The mixture was stirred for 30 min, and then added a solution of 5 (4.0 g, 24.36 mmol) in THF (13 mL) at the same temperature. After 1.5 h saturated aq. solution of NH4Cl (20 mL) was added carefully. The mixture was extracted with EtOAc for two times, the combined organic phases were dried over MgSO4 and the solvent was removed under vacuum. The crude product was purified by column chromatography on silica gel eluted with (20% EtOAc in hexane) to give 6 (5.13 g) in 95% yield. Colorless oil; Yield 95%; Rf = 0.30 (20% EtOAc in hexane); [α]D22 3.7 (c 1.00, CHCl3), –10.0 (c 0.50, EtOH), 3.2 (c 1.00, toluene); 1H-NMR (270 MHz, CDCl3) δ 7.37-7.29 (5H, m), 5.80 (1H, ddt, J = 16.9, 10.1, 6.7 Hz), 5.02 (1H, br d, J = 16.9 Hz), 4.95 (1H, br d, J = 10.1 Hz), 4.56 (2H, s), 3.82 (1H, m), 3.51 (1H, dd, J = 9.4, 3.1 Hz), 3.32 (1H, dd, J = 9.4, 8.0 Hz), 2.35 (1H, br), 2.11-2.06 (2H, m), 1.60-1.41 (4H, m); 13C-NMR (100 MHz, CDCl3) δ 138.6, 137.9, 128.4, 127.8, 127.7, 114.6, 74.6, 73.3, 70.2, 33.6, 32.5, 24.7.
(2S)-(1-Benzyloxyhept-6-en-2-yloxy)tert-butyldimethylsilane (7).9 To a mixture of alcohol 6 (1.96 g, 8.89 mmol) and imidazole (2.66 g, 39.12 mmol) in DMF (10 mL) was added TBDMSCl (1.61 g, 10.67 mmol) at room temperature and it was stirred for 20 h. Solvent was removed under reduced pressure and water (40 mL) was added to the residue. The mixture was extracted with CH2Cl2 for three times (30 mL X3) and combined extract was washed with water and brine. The extract was dried over MgSO4, and evaporated to give crude product which was purified by column chromatography on silica gel eluted with 10% EtOAc in hexane. Compound 7 (2.78 g) was obtained in 93% yield as colorless oil. Yield 93%; Rf = 0.63 (10% EtOAc in hexane); [α]D22 –13.8 (c 1.00, CHCl3); 1H-NMR (400 MHz, CDCl3) δ 7.35-7.28 (5H, m), 5.79 (1H, ddt, J = 17.7, 10.1, 6.5 Hz), 5.01 (1H, dq, J = 17.7, 1.6 Hz), 4.94 (1H, dq, J = 10.1, 1.6 Hz), 4.52 (2H, s), 3.78 (1H, m), 3.39 (1H, dd, J = 9.6, 5.7 Hz), 3.35 (1H, dd, J = 9.6, 5.4 Hz), 2.08-2.01 (2H, m), 1.61-1.40 (4H, m), 0.84 (9H, s), 0.05 (3H, s), 0.04 (3H, s); 13C-NMR (100 MHz, CDCl3) δ 138.9, 138.5, 128.3, 127.6, 127.5, 114.4, 74.8, 73.3, 71.4, 34.2, 33.8, 25.9, 24.5, 18.2, –4.4, –4.8.
(2S)-6-Benzyloxy-5-(tert-butyldimethylsilyl)oxyhexanal (8).10 To a solution of 7 (3.0 g, 8.97 mmol) in CH2Cl2 (66 mL) was bubbled ozone at –78 °C until the starting material was consumed which was monitored on tlc. An excess of ozone was removed out by nitrogen stream at the same temperature. Then, the mixture was quenched with PPh3 (2.8 g, 10.76 mmol) and the mixture was allowed to warm up to the room temperature. Solvent was removed under reduced pressure and the residual material was purified by column chromatography on silica gel eluted with (5% EtOAc in hexane) to give 8 (2.66 g) in 88% yield. Colorless oil; Rf = 0.33 (10% EtOAc in hexane); [α]D21 –16.6 (c 1.00, CHCl3); 1H-NMR (400 MHz, CDCl3) δ 9.75 (1H, t, J = 1.7 Hz), 7.37-7.27 (5H, m), 4.51 (2H, s), 3.83 (1H, m), 3.41 (1H, dd, J = 9.5, 5.4 Hz), 3.34 (1H, dd, J = 9.5, 5.8 Hz), 2.43 (2H, td, J = 7.2, 1.7 Hz), 1.80-1.41 (4H, m), 0.87 (9H, s), 0.05 (3H, s), 0.04 (3H, s); 13C-NMR (100 MHz, CDCl3) δ 202.6, 138.3, 128.3, 127.6, 127.6, 74.4, 73.3, 71.0, 43.9, 34.0, 25.9, 18.1, –4.4, –4.8.
(S,3E,5E)-11-Benzyloxy-10-(tert-butyldimethylsilyl)oxyundeca-3,5-dien-2-one (9). To a cooled solution of (E)-diethyl 4-oxopent-2-enylphosphonate (981 mg, 4.46 mmol) in THF (13 mL) at –40 °C was dropped a THF solution of LiHMDS (1.3 M in THF, 3.2 mL, 4.18 mmol) slowly and the mixture was further stirred for 20 min. Then, a solution of aldehyde 8 (937 mg, 2.78 mmol) in THF (13 mL) was added dropwise at the same temperature. The mixture was stirred overnight at –20 °C. Water (30 mL) was added to the mixture and the mixture was extracted with EtOAc three times (30 mL X3). The organic extract was washed with water and brine, dried over MgSO4, and condensed in vacuo. The residual oil was purified by column chromatography on silica gel eluted with (5% EtOAc in hexane) to provide 9 (118 mg) in 10% yield. Colorless oil; Rf = 0.26 (10% EtOAc in hexane); [α]D22 –14.4 (c 1.00, CHCl3); IR (neat) ㎝-1: 1669; 1H-NMR (270 MHz, CDCl3) δ 7.34-7.29 (5H, m), 7.10 (1H, m), 6.18-6.15 (2H, m), 6.05 (1H, d, J = 15.6 Hz), 4.52 (2H, s), 3.82 (1H, m), 3.40 (1H, dd, J = 9.5, 5.4 Hz), 3.34 (1H, dd, J = 9.5, 5.7 Hz), 2.26 (3H, s), 2.22-2.16 (2H, m), 1.58-1.44 (4H, m), 0.88 (9H, s), 0.05 (3H, s), 0.04 (3H, s); 13C-NMR (100 MHz, CDCl3) δ 198.8, 145.4, 144.0, 138.3, 129.0, 128.9, 128.3, 127.6, 127.5, 74.6, 73.3, 71.1, 34.1, 33.1, 27.1, 25.9, 24.3, 18.1, –4.4, –4.8; LR-MS (EI) m/z (rel. int. %) 402 (M+, 1.2), 345 (3), 281 (11), 149 (16), 91 (base); HR-MS (EI) m/z calcd for C24H38O3Si (M)+ 402.2590, found 402.2582.
(3E,5E,10S)-11-Benzyloxy-10-(tert-butyldimethylsilyl)oxyundeca-3,5-dien-2-ol (10+11). To a stirred solution of 9 (160 mg, 0.40 mmol) and CeCl3・7H2O (148 mg, 0.40 mmol) in MeOH (4.5 mL) was added NaBH4 (15 mg, 0.40 mmol) by several portions at room temperature. After 5 min the mixture was quenched with acetone (0.1 mL) and diluted with water (20 mL). The whole mixture was extracted with Et2O (30 mL X3) and ethereal extract was washed with water and brine. The extract was dried over MgSO4 and condensed. The residue was chromatographed on silica gel eluted with 5% EtOAc in hexane to give unseparable diastereomeric mixtures of 10 and 11 (134 mg) in 83% yield. Colorless oil; Rf = 0.34 (20% EtOAc in hexane).
(S,E)-Ethyl 6-benzyloxy-5-(tert-butyldimethylsilyl)oxyhex-2-enoate (12). To a cooled solution of triethyl phosphonoacetate (360 mg, 1.61 mmol) in THF (5 mL) at 0 °C was added NaH (60%, 59 mg, 1.48 mmol) slowly. Then, a solution of aldehyde 8 (450 mg, 1.34 mmol) in THF (6 mL) was added dropwise at the same temperature. The mixture was stirred 30 min at rt. Water (30 mL) was added to the mixture and the mixture was extracted with Et2O three times (30 mL X3). The organic extract was washed with water and brine, dried over MgSO4, and condensed in vacuo. The residual oil was purified by column chromatography on silica gel eluted with (5% EtOAc in hexane) to provide 12 (453 mg) in 83% yield. Colorless oil; Rf = 0.47 (10% EtOAc in hexane); [α]D22 –13.0 (c 1.00, CHCl3); IR (neat) cm-1: 1721, 1256; 1H-NMR (400 MHz, CDCl3) δ 7.36-7.25 (5H, m), 6.95 (1H, dt, J = 15.6, 6.9 Hz), 5.81 (1H, dt, J = 15.6, 1.6 Hz), 4.51 (2H, s), 4.18 (2H, q, J = 7.1 Hz), 3.82 (1H, m), 3.39 (1H, dd, J = 9.5, 5.4 Hz), 3.34 (1H, dd, J = 9.5, 5.7 Hz), 2.22-2.17 (2H, m), 1.63-1.54 (2H, m), 1.52-1.41 (2H, m), 1.29 (3H, t, J = 7.1 Hz) 0.87 (9H, s), 0.05 (3H, s), 0.04 (3H, s); 13C-NMR (100 MHz, CDCl3) δ 166.7, 149.1, 138.3, 128.3, 127.6, 127.5, 121.4, 74.6, 73.3, 71.1, 60.1, 34.1, 32.3, 25.9, 23.6, 18.1, 14.3, –4.4, –4.8.; LR-MS (EI) m/z (rel. int. %) 406 (M+, 0.1), 349 (6), 285 (18), 183 (19), 91 (base); HR-MS (EI) m/z calcd for C23H38O4Si (M)+ 406.2539, found 406.2534.
8-Benzyloxy-7-(tert-butyldimethylsilyl)oxyoctan-1-ol (13). To a cooled suspension of LiAlH4 (19 mg, 0.49 mmol) in Et2O (5 mL) at –78 °C was dropped a solution of ester 12 (200 mg, 0.49 mmol) in Et2O (2 mL) under argon atomosphere slowly. The mixture was stirred overnight at the same temperature. Sat. aq. potassium sodium tartrate (20 mL) was added to the mixture and the mixture was extracted with Et2O three times (10 mL X3). The organic extract was washed with water and brine, dried over MgSO4, and condensed in vacuo. The residual oil was purified by column chromatography on silica gel eluted with (20% EtOAc in hexane) to provide 13 (50 mg) in 28% yield. Colorless oil; Rf = 0.25 (20% EtOAc in hexane); [α]D22 –1.0 (c 0.15, CHCl3); IR (neat) cm-1: 3377; 1H-NMR (400 MHz, CDCl3) δ 7.36-7.25 (5H, m), 4.52 (2H, s), 3.81 (1H, m), 3.63 (2H, t, J = 6.6 Hz), 3.39 (1H, dd, J = 9.5, 5.6 Hz), 3.35 (1H, dd, J = 9.5, 5.4 Hz), 1.57-1.22 (10H, m), 0.88 (9H, s), 0.05 (3H, s), 0.04 (3H, s); 13C-NMR (100 MHz, CDCl3) δ 138.5, 128.3, 127.6, 127.5, 74.8, 73.3, 71.5, 63.1, 34.6, 32.7, 29.6, 25.9, 25.7, 25.2, 18.2, –4.4, –4.8; LR-MS (EI) m/z (rel. int. %) 366 (M+, 0.1), 245 (23), 217 (10), 201 (15), 91 (base); HR-MS (EI) m/z calcd for C21H38O3Si (M)+ 366.2590, found 366.2585.
Lipase catalyzed kinetic acetylation; preparation of 14 and 10. To a mixture of 10 and 11 (134 mg, 0.33 mmol) in diisopropyl Et2O (3.3 mL), Novozyme 435 (13.4 mg, 10 wt%), vinyl acetate (0.32 mL, 3.48 mmol) and MS 4Å (134 mg) were added successively. The mixture was stirred overnight at room temperature and filtered through celite pad. The filterate was condensed and acetate and alcohol were separated by column chromatography on silica gel. Elution with 5% EtOAc in hexane gave 14 (69 mg) in 47% yield and elution with 15% EtOAc in hexane gave recovery of 10 (59 mg) in 44% yield.
14; Colorless oil; Rf = 0.28 (5% EtOAc in hexane); [α]D22 +25.3 (c 0.60, CHCl3); IR (neat) cm-1: 1737, 1242; 1H-NMR (270 MHz, CDCl3) δ; 7.37-7.28 (5H, m), 6.20 (1H, dd, J = 15.2, 10.4 Hz), 5.99 (1H, dd, J = 15.0, 10.4 Hz), 5.70 (1H, dt, J = 15.0, 7.0 Hz), 5.54 (1H, dd, J = 15.2, 6.9 Hz), 5.37 (1H, dq, J = 6.9, 6.4 Hz), 4.51 (2H, s), 3.81 (1H, m), 3.39 (1H, dd, J = 9.1, 5.1 Hz), 3.33 (1H, dd, J = 9.1, 5.2 Hz), 2.11-2.06 (2H, m), 2.04 (3H, s), 1.55-1.35 (4H, m), 1.32 (3H, d, J = 6.4 Hz), 0.87 (9H, s), 0.04 (3H, s), 0.04 (3H, s); 13C-NMR (100 MHz, CDCl3) δ 170.4, 138.4, 136.2, 132.1, 129.8, 129.3, 128.3, 127.6, 127.5, 74.7, 73.3, 71.3, 70.9, 34.2, 32.7, 25.9, 24.7, 21.4, 20.3, 18.2, –4.4, –4.8; LR-MS m/z (rel. int. %) 446 (M+, 0.1), 237 (55), 163 (41), 145 (39), 91 (base); HR-MS (EI) m/z calcd for C26H42O4Si (M)+ 446.2852, found 446.2850.
10; Colorless oil; Rf = 0.28 (5% EtOAc in hexane); [α]D20 –13.9 (c 1.00, CHCl3); IR (neat) cm-1: 3367; 1H-NMR (400 MHz, CDCl3) δ 7.36-7.27 (5H, m), 6.16 (1H, dd, J = 15.1, 10.3 Hz), 6.00 (1H, dd, J = 15.0, 10.3 Hz), 5.68 (1H, dt, J = 15.0, 7.3 Hz), 5.62 (1H, dd, J = 15.1, 6.6 Hz), 4.51 (2H, s), 4.33 (1H, m), 3.82 (1H, m), 3.39 (1H, dd, J = 9.5, 5.6 Hz), 3.35 (1H, dd, J = 9.5, 5.5 Hz), 2.10-2.05 (2H, m), 1.56-1.41 (4H, m), 1.28 (3H, d, J = 6.4 Hz), 0.88 (9H, s), 0.05 (3H, s), 0.04 (3H, s); 13C-NMR (100 MHz, CDCl3) δ 138.4, 135.3, 134.7, 130.0, 129.6, 128.3, 127.6, 127.5, 74.7, 73.3, 71.3, 68.7, 34.2, 32.7, 25.9, 24.8, 23.3, 18.2, –4.4, –4.8; LR-MS m/z (rel. int. %) 404 (M+, 0.2), 329 (14), 237 (23), 145 (20), 91 (base); HR-MS (EI) m/z calcd for C24H40O3Si (M)+ 404.2747, found 404.2752.
(2R,3E,5E,10S)-11-Benzyloxy-10-(tert-butyldimethylsilyl)oxyundeca-3,5-dien-2-ol (11). To a solution of 14 (69 mg, 0.15 mmol) in MeOH (0.8 mL) was added anhydrous K2CO3 (32 mg, 0.23 mmol) and the mixture was stirred for 1 h at room temperature. The reaction mixture was filtered and the filtrate was condensed in vacuo. The residue was purified by column chromatography on silica gel eluted with 5% EtOAc in hexane to give alcohol 11 (51 mg) in 82% yield. Colorless oil; [α]D20 –14.3 (c 1.00, CHCl3); IR (neat) cm-1: 3397; 1H-NMR (400 MHz, CDCl3) δ 7.36-7.27 (5H, m), 6.16 (1H, dd, J = 15.3, 10.3 Hz), 6.00 (1H, dd, J = 15.0, 10.3 Hz), 5.68 (1H, dt, J = 15.0, 7.3 Hz), 5.62 (1H, dd, J = 15.3, 6.6 Hz), 4.51 (2H, s), 4.33 (1H, m), 3.82 (1H, m), 3.39 (1H, dd, J = 9.5, 5.6 Hz), 3.35 (1H, dd, J = 9.5, 5.5 Hz), 2.10-2.05 (2H, m), 1.56-1.41 (4H, m), 1.28 (3H, d, J = 6.4 Hz), 0.88 (9H, s), 0.05 (3H, s), 0.04 (3H, s); 13C-NMR (100 MHz, CDCl3) δ 138.4, 135.3, 134.7, 130.0, 129.6, 128.3, 127.6, 127.5, 74.7, 73.3, 71.3, 68.7, 34.2, 32.7, 25.9, 24.8, 23.3, 18.2, –4.4, –4.8.; LR-MS m/z (rel. int. %) 404 (M+, 0.2), 329 (12), 237 (22), 145 (19), 91 (base); HR-MS (EI) m/z calcd for C24H40O3Si (M)+ 404.2747, found 404.2755.
Desilylation of compounds 10 and 11. A mixture of silyl ether 10 (47 mg, 0.12 mmol) in THF (0.6 mL) and TBAF (1.0 M in THF, 0.34 mL, 0.34 mmol) was stirred at room temperature overnight. Water (10 mL) was added to the mixture and it was extracted with Et2O (30 mL X3). Organic extracts were combined, washed with brine, dried over MgSO4 and condensed. The crude product was purified by column chromatography on silica gel eluted with 50% EtOAc in hexane to give diol 1. Syn diol 1 was obtained in 90% yield from 10. Compound 2 was obtained in 85% yield from 11.
(2S,3E,5E,10S)-11-Benzyloxyundeca-3,5-diene-2,10-diol (1); Colorless oil; Rf = 0.30 (40% EtOAc in hexane); [α]D20 –4.5 (c 0.50, EtOH); IR (neat) cm-1: 3389; 1H-NMR (400 MHz, CDCl3) δ 7.38-7.26 (5H, m), 6.15 (1H, dd, J = 15.2, 10.6 Hz), 6.01 (1H, dd, J = 15.0, 10.6 Hz), 5.68 (1H, dt, J = 15.0, 7.2 Hz), 5.62 (1H, dd, J = 15.2, 6.6 Hz), 4.55 (2H, s), 4.33 (1H, m), 3.81 (1H, m), 3.50 (1H, dd, J = 9.4, 3.0 Hz), 3.31 (1H, dd, J = 9.4, 7.9 Hz), 2.31 (1H, brs), 2.13-2.08 (2H, m), 1.61-1.42 (4H, m), 1.28 (3H, d, J = 6.4 Hz); 13C-NMR (100 MHz, CDCl3) δ 137.9, 134.8, 129.9, 129.8, 128.5, 128.3, 127.8, 127.7, 74.6, 73.3, 70.2, 68.6, 32.5, 32.5, 25.1, 23.3; LR-MS (FAB) m/z 313 (M+Na)+; HR-MS (FAB) m/z calcd for C18H26O3 Na (M+Na)+ 313.1780, found 313.1783.
(2R,3E,5E,10S)-11-Benzyloxyundeca-3,5-diene-2,10-diol (2); Colorless oil; Rf = 0.30 (40% EtOAc in hexane); [α]D20 –26.2 (c 0.50, EtOH); IR (neat) cm-1: 3389; 1H-NMR (400 MHz, CDCl3) δ 7.38-7.26 (5H, m), 6.15 (1H, dd, J = 15.2, 10.6 Hz), 6.01 (1H, dd, J = 15.0, 10.6 Hz), 5.68 (1H, dt, J = 15.0, 7.2 Hz), 5.62 (1H, dd, J = 15.2, 6.6 Hz), 4.55 (2H, s), 4.33 (1H, m), 3.81 (1H, m), 3.50 (1H, dd, J = 9.4, 3.0 Hz), 3.31 (1H, dd, J = 9.4, 7.9 Hz), 2.31(1H, brs), 2.13-2.08 (2H, m), 1.61-1.42 (4H, m), 1.28 (3H, d, J = 6.4 Hz); 13C-NMR (100 MHz, CDCl3) δ 137.9, 134.8, 129.9, 129.8, 128.5, 128.3, 127.8, 127.7, 74.6, 73.3, 70.2, 68.6, 32.5, 32.5, 25.1, 23.3; LR-MS (FAB) m/z 313 (M+Na)+; HR-MS (FAB) m/z calcd for C18H26O3 Na (M+Na)+ 313.1780, found 313.1787.
Pd-catalyzed cyclization of 3 and 4. To a solution of diol 1 (30 mg, 0.104 mmol) in THF (0.3 mL) was added PdCl2(MeCN)2 (4.0 mg, 15.4 µmol) and the mixture was stirred at room temperature overnight. Water (5 mL) and Et2O (20 mL) were added and the ethereal layer was washed with brine. The organic extract was dried over MgSO4 and condensed. The crude material was purified by silica gel column chromatography eluted with 10% EtOAc in hexane to give a mixture of compounds 3 and 4 (22 mg) in 78% yield. The diastereomeric ratio was identified by proton NMR to be 3:1. They were separated by HPLC to give less polar isomer 3 and polar isomer 4. The reaction of 2 under the same conditions gave a mixture of 3 and 4 (26 mg) in 89% yield in a 3:1 ratio.
(2S,6R)-2-Benzyloxymethyl-6-[(1E,3E)-penta-1,3-dienyl]tetrahydro-2H-pyran (3); Colorless oil; Yields in several attempts, 55–70%; Rf = 0.33 (5% EtOAc in hexane); [α]D20 –4.6 (c 0.63, EtOH); 1H-NMR (400 MHz, CDCl3) δ 7.35-7.25 (5H, m), 6.18 (1H, dd, J = 15.4, 10.3 Hz), 6.02 (1H, ddq, J = 15.0, 10.3, 1.3 Hz), 5.67 (1H, dq, J = 15.0, 6.8 Hz), 5.58 (1H, dd, J = 15.4, 6.2 Hz), 4.60 (1H, d, J = 12.3 Hz), 4.55 (1H, d, J = 12.3 Hz), 3.88 (1H, m), 3.63 (1H, m), 3.52 (1H, dd, J = 10.0, 5.8 Hz), 3.42 (1H, dd, J = 10.0, 4.6 Hz), 1.88 (1H, m), 1.74 (3H, dd, J = 6.8, 1.3 Hz), 1.64-1.49 (3H, m), 1.39-1.26 (2H, m); 13C-NMR (100 MHz, CDCl3) δ 138.6, 131.7, 131.3, 130.7, 129.6, 128.5, 127.9, 127.7, 78.1, 77.0, 73.8, 73.5, 31.8, 28.2, 23.3, 18.3; LR-MS (EI) m/z (rel. int. %) 272 (M+, 4), 181 (10), 91 (base), 67 (30); HR-MS (EI) m/z calcd for C18H24O2 (M)+ 272.1776, found 272.1769.
(2S,6S)-2-Benzyloxymethyl-6-[(1E,3E)-penta-1,3-dienyl]tetrahydro-2H-pyran (4); Colorless oil; Yields in several attempts, 18–23%; Rf = 0.23 (5% EtOAc in hexane); [α]D20 –14.6 (c 0.25, EtOH); 1H-NMR (400 MHz, CDCl3) δ 7.34-7.26 (5H, m), 6.18 (1H, ddd, J = 15.4, 10.5, 1.3 Hz), 6.07 (1H, ddq, J = 14.8, 10.5, 1.5 Hz), 5.67 (1H, dq, J = 14.8, 6.9 Hz), 5.67 (1H, dd, J = 15.4, 4.5 Hz), 4.59 (1H, d, J = 12.2 Hz), 4.55 (1H, d, J = 12.2 Hz), 4.44 (1H, m), 3.93 (1H, m), 3.52 (1H, dd, J = 10.0, 6.0 Hz), 3.43 (1H, dd, J = 10.0, 4.9 Hz), 1.76 (3H, d, J = 6.9 Hz), 1.71-1.51 (5H, m), 1.43 (1H, m); 13C-NMR (100 MHz, CDCl3) δ 138.6, 132.0, 131.4, 130.8, 129.5, 128.5, 127.8, 127.6, 73.4, 72.8, 72.5, 70.2, 29.3, 27.8, 18.8, 18.3; LR-MS (EI); m/z (rel. int. %) 272 (M+, 28), 181 (67), 91 (base), 67 (28); HR-MS (EI) m/z calcd for C18H24O2 (M)+ 272.1776, found 272.1779.
ACKNOWLEDGEMENTS
This work was financially supported by Grsn-Aid for Scientific Research from the Ministry of Education, Science and Culture, Japan.
References
1. Allylic substitution (a) S. Oliver and P. A. Evans, Synthesis, 2013, 45, 3179; (b) J. F. Hartwig and L. M. Stanley, Acc. Chem. Res., 2010, 43, 1461; CrossRef (c) Z. Lu and S. Ma, Angew. Chem. Int. Ed., 2008, 47, 258; CrossRef (d) B. M. Trost and M. L. Crawley, Chem. Rev., 2003, 103, 2921; CrossRef (e) H. Miyabe and Y. Takemoto, Synlett, 2005, 1641; (f) M. Johannsen and K. A. Jørgensen, Chem. Rev., 1998, 98, 1689; CrossRef (g) M. L. Crawley, In Science of Synthesis, Stereoselective Synthesis; Vol. 3; ed. by P. A. Evans, Thieme, Stuttgart, 2011, 403.
2. (a) J. Uenishi, M. Ohmi, and A. Ueda, Tetrahedron: Asymmetry, 2005, 16, 1299; CrossRef (b) N. Kawai, J. M. Lagrange, M. Ohmi, and J. Uenishi, J. Org. Chem., 2006, 71, 4530; CrossRef (c) N. Kawai, J.-M. Lagrange, and J. Uenishi, Eur. J. Org. Chem., 2007, 2808; CrossRef (d) J. Uenishi, Y. S. Vikhe, and N. Kawai, Chem. Asian J., 2008, 3, 473. CrossRef
3. (a) N. Kawai, S. Hande, and J. Uenishi, Tetrahedron, 2007, 63, 9049; CrossRef (b) S. M. Hande and J. Uenishi, Tetrahedron Lett., 2009, 50, 189; CrossRef (c) S. S. Palimkar, J. Uenishi, and H. Ii, J. Org. Chem., 2012, 77, 388; CrossRef (d) M. Kunitake, T. Oshima, K. Konoki, M. Ebine, K. Torikai, M. Murata, and T. Oishi, J. Org. Chem., 2014, 79, 4948; CrossRef (e) S. Hanessian, T. Focken, and R. Oza, Tetrahedron, 2011, 67, 9870. CrossRef
4. R. Barbe and J. Hasserodt, Tetrahedron, 2007, 63, 2199. CrossRef
5. B. S. Lee, S. Y. Lee, and D. Y. Oh, J. Org. Chem., 2000, 65, 4175. CrossRef
6. P. S. Deore and N. P. Argade, J. Org. Chem., 2014, 79, 2538. CrossRef
7. K. Tatsuta, T. Yamaguchi, Y. Tsuda, Y. Yamaguchi, N. Hattori, H. Nagai, and S. Hosokawa, Tetrahedron Lett., 2007, 48, 4187. CrossRef
8. Although we have carefully check its specific rotation in different solvents, the polarity of the degree in CHCl3 is opposite to that reported in literature. S. Hofman, G. D. Baecke, B. Kenda, and P. J. De Clercq, Synthesis, 1998, 479. CrossRef
9. Racemic material was reported; C. Bonini, L. Chiummiento, M. Funicello, P. Lupattelli, and M. Pullez, Eur. J. Org. Chem., 2006, 80. CrossRef
10. F. M. Uckun, C. Mao, A. O. Vassilev, H. Huang, and S. T. Jan, Bioorg. Med. Chem. Lett., 2000, 10, 541. CrossRef