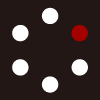
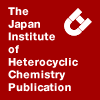
HETEROCYCLES
An International Journal for Reviews and Communications in Heterocyclic ChemistryWeb Edition ISSN: 1881-0942
Published online by The Japan Institute of Heterocyclic Chemistry
e-Journal
Full Text HTML
Received, 17th July, 2014, Accepted, 25th August, 2014, Published online, 28th August, 2014.
DOI: 10.3987/COM-14-S(K)86
■ Stereoselective Construction of 1β-Azide- and 1β-Cyano-2-deoxyribose Derivatives
Hiromichi Fujioka,* Takahiro Moriya, Kazuhisa Okamoto, Yutaka Minamitsuji, Yoshifumi Ueyama, Nao Matsumoto, and Kenichi Murai
Graduate School of Pharmaceutical Science, Osaka University, 1-6 Yamadaoka, Suita, Osaka 560-0871, Japan
Abstract
A new method has been developed for the β-selective introduction of azido and cyano groups at the anomeric position of 2-deoxyribose derivatives. This method proceeds via the formation of a collidinium salt intermediate and allows for the stereoselective construction of 1β-azido- and 1β-cyano-2-deoxy-D-ribose derivatives. 2-Deoxy-D-ribose compounds bearing an acetoxy or tert-butoxycarbonyloxy group at their anomeric position performed well as starting materials for the formation of the corresponding 1β-azide and 1β-cyanide derivatives, respectively. 1H NMR studies of the salt intermediates revealed that the nucleophilic substitution reaction of the salt intermediates proceeded in a SN2-fashion.INTRODUCTION
The azido group is a versatile functional group in synthetic chemistry, which can be readily converted to amino group.1 Azides can also be converted to triazoles by their reaction with alkynes using click chemistry.2 The cyano group is another versatile functional group, which can be transformed to a variety of different functional groups, including aldehydes, carboxylic acids, amides and amines.1 Since D-ribose and 2-deoxy-D-ribose derivatives are constituents of RNA and DNA, respectively, their 1β-azido and 1β-cyano derivatives could be useful building blocks for the synthesis of modified RNA and DNA molecules. For this reason, significant research efforts have been directed towards the development of synthetic methods for the introduction of azido and cyano groups at the anomeric positions of D-ribose and 2-deoxy-D-ribose derivatives. Among the many different methods currently available for these transformations, the Lewis acid catalyzed reactions of 1-O-acylsugars with TMSN3 and TMSCN have been successfully applied to ribose sugars. Notably, this particular method allows for the stereoselective introduction of the azido and cyano groups at the anomeric position of the ribose through the neighboring group effect of the 2-hydroxy group.3 In 2-deoxyribose, however, it is not possible to achieve the stereoselective introduction of these nucleophiles using this method, because of the lack of a 2-hydroxy group, and the β-stereoselective introduction of nucleophiles using 1-O-acyl 2-deoxy-D-ribose derivatives is therefore particularly challenging.
In fact, the Lewis acid mediated reaction of a 1-O-acetyl derivative with TMSN3 affords the corresponding α-azide product as the major isomer [Scheme 1, (a)].4 Similar levels of stereoselectivity are also observed for the introduction of a cyano group using this method, with the α-cyano products being obtained as the major isomer [Scheme 1, (b)].5 To the best of our knowledge, the only method available for the selective synthesis of the β-azide and β-cyanide products involves the reaction of α-chloro derivatives with CsN3 or NaCN, respectively, in polar solvents [Scheme 1, (c) (d)]. There are, however, several disadvantages, to this method, including limited substrate scope and poor selectivity.6,7 The development of a new method allowing for the stereoselective introduction of β-azido and β-cyano groups at the anomeric position of 2-deoxyribose derivatives is therefore highly desired.
Herein, we report the development of a new method for the stereoselective synthesis of 1β-azido- and 1β-cyano-2-deoxyribose derivatives selectively from 1-acyloxy or 1-carbonate 2-deoxyriboses.
RESULTS AND DISCUSSION
Introduction of azido group
We have recently reported a new method for the introduction of nucleophiles at the anomeric position of 6-membered oxacyclic compounds.8 This particular method proceeded via the formation of pyridinium salt intermediates, which were prepared from the corresponding 1-acetoxy derivatives using a pre-activation method. We subsequently examined the application of this pre-activation method to 1-O-acetyl-2-deoxy-D-ribose derivative 1a.9 We initially applied the optimum conditions from our previously reported work with 6-membered oxacyclic compounds (i.e. TMSOTf and 2-p-tolylpyridine) to compound 1a. Disappointingly, however, when 2-p-tolylpyridine was used as a base, the azide product 2a was obtained in high yield with very poor selectivity (Scheme 2).
A variety of different bases were then screened against the reaction to evaluate their impact on the selectivity (Table 1). In our previous work,8 TMSN3 and tetra-n-butylammonium difluorotriphenylsilicate (TBAT) were used for the azidation reaction. Given that tetra-n-butylammonium fluoride (TBAF) and TBAT provided similar yields and selectivity (Table 1, entry 1 and Scheme 2), TBAF was used as a fluoride source in all of the following experiments because of ease of removal from the product 2a. The use of pyridine and 2-ethylpyridine as a base in the reaction did not result in any improvement in the selectivity (entries 2 and 3), and 2,2’-bipyridyl (entry 4) and 2-chloropyridine (entry 5) resulted in only moderate improvements in the selectivity. The use of 2-acetylpyridine led to a significant improvement in the selectivity of the reaction (entry 6). Pleasingly, the use of 2,4,6-collidine, which resulted in the formation of enol ethers in 6-membered substrates,8 afforded the highest selectivity of all of the bases tested in the current study (entry 7) although a small amount of enol ether 3 was formed as a by-product (see Figure 1, Charts 3 and 4). The use of much bulkier 2,6-di-tert-butylpyridine as a base was unsuccessful, most likely because it could not form pyridinium-salt intermediate, and no azide-containing product was obtained from the reaction (entry 8).
Having identified 2,4,6-collidine as the optimum base of β-selectivity, we proceeded to investigate the scope and generality of the reaction using various 1-O-acetyl-2-deoxy-D-ribose derivatives (Table 2). Substrates 1b and 1c bearing acid-labile functional groups such as tert-butyldimethylsilyl (entry 1) and trityl (entry 2) groups gave the corresponding β-azide isomers 2b and 2c as the major products, respectively. Even the substrate 1d, which has been reported to give the α-isomer as the major product under Lewis acidic conditions (see Scheme 1), gave the β-isomer 2d with high selectivity (entry 3).
Introduction of cyano group
Based on our success with the β-selective introduction of an azido functional group at the anomeric position of 2-deoxyribose derivatives, we proceeded to investigate the application of this method to the selective formation of 1β-cycano-2-deoxyribose derivatives (Table 3). Treatment of the 1-acetoxy derivative 1a with TMSOTf and 2,4,6-collidine gave the salt intermediate, which was treated with TMSCN to give the cyano compound 2e in a low yield with the α-isomer being formed as the major product (Table 3, entry 1). TBAF and TBAT were then evaluated as activating agents for the TMSCN. The addition of TBAF proved to be unsuccessful, with only a trace amount of the desired product being formed in this reaction (entry 2). In contrast, the addition of TBAT to the reaction resulted in the formation of the desired product, albeit in a low yield, with high β-selectivity (entry 3). It is noteworthy that the reactions with TBAF and TBAT also resulted in the regeneration of the starting material 1a from the salt intermediate.10
The regeneration of starting material 1a in these cases can be rationalized by the presence of TMSOAc, which would be produced as a by-product during the formation of the salt A (Scheme 3). TMSOAc would be activated by TBAF or TBAT to give an acetate anion, which would react with the salt A at a faster rate than TMSCN to give 1a. The reaction would not occur in the case of TMSN3 because of high nucleophilicity of the azide anion.
In the attempt to reduce the nucleophilicity of the by-product formed during this reaction, we investigated the use of several different groups as leaving groups at the anomeric position of the starting material (Table 4). The use of a benzoyloxy (Table 4, entry 1) or p-fluorobenzoyloxy group (entry 2) as the leaving group resulted in a small improvement in the yield, but the starting materials 1e and 1f were also regenerated in both cases. Furthermore, the use of a strongly electron-withdrawing p-nitrobenzoyloxy group was unsuccessful, with none of the salt intermediate being formed (entry 3). Substrate 1h bearing a Boc group was then examined with the expectation that the resulting TMSOCO2tBu by-product would decompose to give carbon dioxide and TMSOtBu, which would be only weakly nucleophilic because of its bulkiness. Pleasingly, the application of the optimized conditions to 1h afforded the desired product 2e in moderate yield without the regeneration of 1h (entry 4). Furthermore, the use of TESOTf instead of TMSOTf led to an improvement in the yield of the desired product (entry 5).
The optimized conditions were subsequently applied to the substrate 1i bearing acid-labile TBS ether groups at its 3 and 5-positions. This reaction gave the desired product 2f in a moderate yield with high β-selectivity and highlighted the mild nature of the reaction conditions (Scheme 4).
Mechanistic consideration
To develop a deeper understanding of the different selectivities observed with different bases (see Table 1), we measured the 1H NMR spectra of the salts formed from several different bases.11 The 1H NMR spectrum of the reaction mixture obtained using pyridine (Figure 1, Chart 1) showed that the ratio of the two salts was 42:58 based on the ratio of the signals belonging to the α and β anomeric protons, respectively. The relative stereochemistries of the salts were determined by NOE experiments. The use of 2,2’-bipyridyl as a base led to an increase in the ratio of the α-isomer, with the 1H NMR spectrum of the salt showing a ratio was 65:35 for the α and β isomers (Chart 2). The use of 2,4,6-collidine as a base also afforded the α-isomer as the major isomer. Although the enol ether 312 was accompanied by salts in this case, and one olefinic proton of the enol ether overlapped with the anomeric proton of one salt isomer (Chart 3), the amount of olefinic proton was estimated from other olefinic proton of the enol ether 3 (Chart 4). By deducting the integral for this signal from the overlapping integral, the ratio of the α and β salts was determined to be 78:22.
Taken together, the results of this study demonstrate that the use of the bulky base 2,4,6-collidine, provided the highest ratio of the α-intermediate, which underwent an SN2-substitution reaction with an azide or a cyanide anion to give the corresponding β-substituted products as the major isomer with high selectivity (Scheme 5).
CONCLUSION
We have successfully developed a new method for the selective formation of 1β-azido- and 1β-cyano-2-deoxy-D-ribose derivatives via the formation of collidinium salt intermediates. Furthermore, NMR studies of the salt intermediates formed from different bases revealed that the nucleophilic substitution reaction of the salt intermediates proceeds in a SN2-fashion. Since the azido- and cyano groups can be readily converted to a various functional groups, this method provides a synthetic platform for the construction of a broad range of 2-deoxy-D-ribose derivatives that could be used to make DNA analogs.
EXPERIMENTAL
General Information
Infrared spectra (IR) were recorded on a Shimadzu FTIR 8400 spectrometer using a diffuse reflectance measurement of samples dispersed in KBr powder. 1H NMR and 13C NMR spectra were recorded on a JEOL JNM-LA 500, JNM-ECS 400, JNM-AL 300, and JNM-EX 270 spectrometers in CDCl3 using tetramethylsilane as an internal reference standard. NMR data have been reported as follows: chemical shift in ppm (δ), multiplicity (s = singlet, d = doublet, t = triplet, q = quartet, m = multiplet, brs = broad singlet), coupling constant (Hz) and integration. Mass spectra were obtained on a Shimadzu GCMS-QP 5000 mass spectrometer with an ionization voltages of 70 eV. Column chromatography and TLC were carried out on Merck Silica gel 60 (230-400 mesh) and Kanto Kagaku silica gel 60N (40-50 µm, spherical, neutral), and Merck silica gel F254 plates (0.25 mm), respectively. All of the commercially available reagents used in this study were used as supplied without further purification.
Experiments in Scheme 2
Compounds 1a13 and 2a14 are known compounds.
3,5-Di-O-benzyl-2-deoxy-D-ribofuranosyl azide (2a)
2-p-Tolylpyridine (92 μL, 0.538 mmol) and TMSOTf (65 μL, 0.360 mmol) were added subsequently to a solution of 1a (64.0 mg, 0.180 mmol) in CH2Cl2 (1.8 mL) at 0 oC under N2, and the resulting mixture was stirred for 5 min at the same temperature. TMSN3 (72 μL, 0.544 mmol) and TBAT (295.1 mg, 0.547 mmol) were then added sequentially to the mixture, and the resulting solution was stirred at rt for 5 min. The mixture was then treated with sat. NaHCO3 aq. , and the resulting solution was extracted with CH2Cl2. The organic layer was dried over Na2SO4 and evaporated in vacuo. The residue was purified by SiO2 column chromatography using benzene/Et2O = 5/1 (v/v) then n-hexane/EtOAc/MeOH = 10/1/0.2 (v/v/v) as eluent to give 2a (58.2 mg, 95%, α : β = 45:55)
α-2a: colorless oil; IR (KBr): 2864, 2108, 1207 cm-1; 1H NMR (500 MHz, CDCl3): δ 2.03 (1H, dd, J = 14.0, 1.0 Hz), 2.21-2.26 (1H, m), 3.50 (2H, d, J = 4.0 Hz), 4.05-4.07 (1H, m), 4.41-4.43 (1H, m), 4.47-4.54 (4H, m), 5.53 (1H, d, J = 5.5 Hz), 7.23-7.35 (10H, m); 13C NMR (125 MHz, CDCl3): δ 38.3, 69.9, 71.4, 73.4, 78.7, 84.4, 92.1, 127.55, 127.67, 127.71, 128.3, 137.6, 137.8.
β-2a: colorless oil; IR (KBr): 2864, 2112, 1236 cm-1; 1H NMR (500 MHz, CDCl3): δ 2.05-2.10 (1H, m), 2.16-2.21 (1H, m), 3.52-3.60 (2H, m), 4.13-4.16 (1H, m), 4.25-4.28 (1H, m), 4.45-4.57 (4H, m), 5.54 (1H, dd, J = 6.0, 4.0 Hz), 7.22-7.36 (10H, m); 13C NMR (125 MHz, CDCl3): δ 38.6, 70.7, 71.6, 73.4, 79.3, 83.7, 92.0, 127.59, 127.62, 127.7, 128.3, 128.4, 137.6, 137.9.
General Procedure for the Introduction of an Azido Group to the 2-Deoxyribose Derivatives (Method A)
Pyridines (3.0 eq.) and TMSOTf (2.0 eq.) were successively added dropwise to a solution 2-deoxyribose derivatives in CH2Cl2 (0.1 M) at 0 oC under N2, and the resulting mixture was stirred at the same temperature. After checking the disappearance of the starting material and the formation high polar compound on TLC, TMSN3 (3.0 eq.) and TBAF (3.0 eq.) were added successively to the solution at -40 oC, and the resulting solution was stirred at the same temperature. After checking the disappearance of the high polar compound on TLC, sat. NaHCO3 aq. was added to the mixture, and the resulting solution was extracted with CH2Cl2. The organic layer was dried over Na2SO4 and evaporated in vacuo. The residue was purified by SiO2 column chromatography. Ratio of the diastereomeric isomers was determined by 1H NMR.
Experiments in Table 1
Entry 1: This reaction was carried out using method A with 1a (36.3 mg, 0.102 mmol), CH2Cl2 (1.0 mL), 2-p-tolylpyridine (52 μL, 0.304 mmol), TMSOTf (37 μL, 0.205 mmol), TMSN3 (40 μL, 0.304 mmol) and TBAF (1.0 M THF solution, 0.30 mL, 0.300 mmol) to give 2a (31.0 mg, 90%, α : β = 42:58). Column chromatography: hexane/EtOAc = 4/1 (v/v)
Entry 2: This reaction was carried out using method A with 1a (29.3 mg, 0.082 mmol), CH2Cl2 (0.82 mL), pyridine (20 μL, 0.247 mmol), TMSOTf (37 μL, 0.205 mmol), TMSN3 (32 μL, 0.243 mmol) and TBAF (1.0 M THF solution, 0.25 mL, 0.250 mmol) to give 2a (24.5 mg, 88%, α : β = 58:42). Column chromatography: hexane/EtOAc = 4/1 (v/v)
Entry 3: This reaction was carried out using method A with 1a (47.5 mg, 0.133 mmol), CH2Cl2 (1.3 mL), 2-ethylpyridine (46 μL, 0.402 mmol), TMSOTf (48 μL, 0.266 mmol), TMSN3 (88 μL, 0.669 mmol) and TBAF (1.0 M THF solution, 0.67 mL, 0.670 mmol) to give 2a (42.2 mg, 93%, α : β = 49:51). Column chromatography: benzene/Et2O = 50/1 (v/v)
Entry 4: This reaction was carried out using method A with 1a (49.8 mg, 0.140 mmol), CH2Cl2 (1.4 mL), 2,2’-bipyridyl (65.6 mg, 0.420 mmol), TMSOTf (51 μL, 0.282 mmol), TMSN3 (55 μL, 0.418 mmol) and TBAF (1.0 M THF solution, 0.42 mL, 0.420 mmol) to give 2a (44.4 mg, 93%, α : β = 39:61). Column chromatography: benzene/Et2O = 50/1 (v/v)
Entry 5: This reaction was carried out using method A with 1a (37.7 mg, 0.106 mmol), CH2Cl2 (1.1 mL), 2-chloropyridine (30 μL, 0.317 mmol), TMSOTf (38 μL, 0.210 mmol), TMSN3 (42 μL, 0.319 mmol) and TBAF (1.0 M THF solution, 0.32 mL, 0.320 mmol) to give 2a (31.7 mg, 88%, α : β = 34:66). Column chromatography: benzene/Et2O = 50/1 (v/v)
Entry 6: This reaction was carried out using method A with 1a (47.3 mg, 0.133 mmol), CH2Cl2 (1.3 mL), 2-acetylpyridine (45 μL, 0.401 mmol), TMSOTf (48 μL, 0.266 mmol), TMSN3 (52 μL, 0.395 mmol) and TBAF (1.0 M THF solution, 0.40 mL, 0.400 mmol) to give 2a (41.5 mg, 92%, α : β = 20:80). Column chromatography: benzene/Et2O = 50/1 (v/v)
Entry 7: This reaction was carried out using method A with 1a (63.2 mg, 0.177 mmol), CH2Cl2 (1.8 mL), 2,4,6-collidine (70 μL, 0.531 mmol), TMSOTf (64 μL, 0.354 mmol), TMSN3 (70 μL, 0.532 mmol) and TBAF (1.0 M THF solution, 0.53 mL, 0.530 mmol) to give 2a (47.5 mg, 79%, α : β = 12:88) and 3 (6.3 mg, 12%). Column chromatography: hexane/EtOAc = 8/1 (v/v) Compound 3 is a known compound (Compound 15 in ref. 12).
Experiments in Table 2
Compounds 1b15, 1c16, 1d4 and 2d4 are known compounds.
3,5-Di-O-tert-butyldimethylsilyl-2-deoxy-D-ribofuranosyl azide (2b): This reaction was carried out using method A with 1b (42.4 mg, 0.105 mmol), CH2Cl2 (1.0 mL), 2,4,6-collidine (41 μL, 0.311 mmol), TMSOTf (38 μL, 0.210 mmol), TMSN3 (41 μL, 0.312 mmol) and TBAF (1.0 M THF solution, 0.31 mL , 0.310 mmol) to give 2b (29.9 mg, 74%, α : β = 13 : 87). Column chromatography: hexane/EtOAc = 10/1 (v/v)
Colorless oil; IR (KBr): 2930, 2112, 1253 cm-1; 1H NMR (400 MHz, CDCl3): δ 0.05-0.09 (12H, m), 0.87-0.92 (18H, m), 1.88 (1/8H, dt, J = 13.6, 2.4 Hz), 1.95-2.09 (14/8H, m), 2.27-2.34 (1/8H, m), 3.60-3.70 (2H, m), 3.87-3.91 (7/8H, m), 4.10-4.12 (1/8H, m), 4.32-4.35 (1/8H, m), 4.39-4.43 (7/8H, m), 5.37 (1/8H, dd, J = 6.6, 2.4 Hz), 5.51 (7/8H, dd, J = 5.8, 4.2 Hz); HRMS (FAB): calcd for C17H37N3O3Si2Na [M+Na]+ 410.2271, found 410.2264.
β -2b: 13C NMR (100 MHz, CDCl3): δ -5.50, -5.46, -4.9, -4.8, 17.9, 18.4, 25.7, 25.9, 41.1, 63.0, 71.7, 87.6, 91.6. (For 13C NMR, data of β-isomer were shown)
3-O-Acetyl-5-O-trityl-2-deoxy-D-ribofuranosyl azide (2c): This reaction was carried out using method A with 1c (46.5 mg, 0.101 mmol), CH2Cl2 (1.0 mL), 2,4,6-collidine (40 μL, 0.304 mmol), TMSOTf (37 μL, 0.205 mmol), TMSN3 (40 μL, 0.304 mmol) and TBAF (1.0 M THF solution, 0.30 mL, 0.300 mmol) to give 2c (32.8 mg, 73%, α : β = 15 : 85). Column chromatography: hexane/EtOAc = 4/1 (v/v)
Colorless oil; IR (KBr): 3061, 2928, 2110, 1738, 1234 cm-1; 1H NMR (400 MHz, CDCl3): δ 2.04 (51/20H, s), 2.08 (9/20H, s), 2.14-2.28 (37/20H, m), 2.57-2.64 (3/20H, m), 3.20 (3/20H, A in ABqd, J = 10.4, 3.6 Hz), 3.25-3.32 (34/20H, m), 3.38 (3/20H, B in ABqd, J = 10.4, 4.0 Hz), 4.18-4.22 (17/20H, m), 4.38-4.41 (3/20H, m), 5.21-5.26 (1H, m), 5.62 (17/20H, t, J = 5.8 Hz), 5.68 (3/20H, d, J = 6.0 Hz), 7.23-7.52 (15H, m); HRMS (EI): calcd for C26H25N3O4 [M]+ 443.1845, found 443.1846.
β-2c : 13C NMR (100 MHz, CDCl3): δ 21.0, 38.4, 64.0, 75.1, 84.0, 86.9, 92.1, 127.1, 127.9, 128.7, 143.6, 170.2. (For 13C NMR, data of β-isomer were shown)
3,5-Di-O-acetyl-2-deoxy-D-ribofuranosyl azide (2d)
This reaction was carried out using method A with 25 (29.6 mg, 0.114 mmol), CH2Cl2 (1.1 mL), 2,4,6-collidine (45 μL, 0.342 mmol), TMSOTf (41 μL, 0.227 mmol), TMSN3 (45 μL, 0.342 mmol) and TBAF (1.0 M THF solution, 0.34 mL, 0.340 mmol) to give 2d (20.8 mg, 75%, α : β = 14:86). Column chromatography: hexane/EtOAc = 2/1 (v/v)
Colorless oil; IR (KBr): 2957, 2114, 1744, 1259 cm-1; 1H NMR (400 MHz, CDCl3): δ 2.02-2.12 (43/7H, m), 2.21-2.28 (12/7H, m), 2.38-2.46 (1/7H, m), 4.14-4.19 (1H, m), 4.25-4.36 (13/7H, m), 4.42-4.45 (1/7H, m), 5.09-5.12 (1/7H, m), 5.20-5.24 (6/7H, m), 5.57-5.61 (1H, m); HRMS (FAB): calcd for C9H13N3O5Na [M+Na]+ 266.0753, found 266.0751.
β-2d: 13C NMR (100 MHz, CDCl3): δ 20.8, 20.9, 38.3, 63.8, 74.4, 82.5, 91.9, 170.4, 170.7. (For 13C NMR, data of β-isomer were shown)
General Procedure for the Introduction of a Cyano Group to the 2-Deoxyribose Derivatives (Method B)
Pyridines (3.0 eq.) and silyl triflate (2.0 eq.) were successively added dropwise to a solution 2-deoxyribose derivatives in CH2Cl2 (0.1 M) at 0 oC under N2, and the resulting mixture was stirred at the same temperature. After checking the disappearance of the starting material and the formation high polar compound on TLC, TMSCN (5.0 eq.) and TBAT (5.0 eq.) were added successively to the solution, and the resulting solution was stirred at the same temperature for 3 h and then at rt. After checking the disappearance of the high polar compound on TLC, sat. NaHCO3 aq. was added to the mixture, and the resulting solution was extracted with CH2Cl2. The organic layer was dried over Na2SO4 and evaporated in vacuo. The residue was purified by SiO2 column chromatography. Ratio of the diastereomeric isomers was determined by 1H NMR.
Experiments in Table 4
Compound 2e17 is a known compound.
Compounds 1e-h were synthesized from a known compound 4.10 Compounds 1e-h were obtained as distereomeric mixtures at anomeric position.
1-O-Benzoyl-3,5-di-O-benzyl-2-deoxy-D-ribofuranose (1e)
BzCl (0.23 mL, 1.98 mmol), i-Pr2NEt (0.33 mL, 1.94 mmol), and DMAP (10.0 mg, 0.082 mmol) were added to a solution of 4 (406.5 mg, 1.29 mmol) in CH2Cl2 (10 mL) at 0 oC under N2, and the resulting mixture was stirred at rt for 5 h. Sat. NaHCO3 aq. was added to the mixture, and the resulting solution was extracted with CH2Cl2. The organic layer was dried over Na2SO4 and evaporated in vacuo. The residue was purified by SiO2 column chromatography using hexane/EtOAc = 8/1 (v/v) as an eluent to give 1e (377.2 mg, 70%).
Colorless oil; IR (KBr): 2865, 2250, 1714, 1271 cm-1; 1H NMR (400 MHz, CDCl3): δ 2.34-2.52 (2H, m), 3.55-3.64 (2H, m), 4.20-4.23 (1/2H, m), 4.34-4.40 (1H, m), 4.50-4.58 (9/2H, m), 6.62-6.63 (1H, m), 7.21-7.38 (12H, m), 7.50-7.54 (1H, m), 7.93 (1H, d, J = 6.0 Hz), 8.05 (1H, d, J = 6.0 Hz); 13C NMR (100 MHz, CDCl3): δ 38.1, 39.0, 70.0, 70.7, 71.2, 71.7, 73.3, 73.5, 78.88, 78.90, 84.2, 85.0, 99.2, 99.4, 127.49, 127.53, 127.55, 127.59, 127.61, 127.7, 128.16, 128.25, 128.28, 128.32, 128.4, 129.6, 129.8, 130.0, 130.2, 132.9, 133.0, 137.7, 137.91, 137.93, 138.0, 165.4, 165.8; HRMS (FAB): calcd for C26H26O5Na [M+Na]+ 441.1678, found 441.1671.
3,5-Di-O-benzyl-1-O-fluorobenzoyl-2-deoxy-D-ribofuranose (1f)
p-FBzCl (0.18 mL, 1.53 mmol), i-Pr2NEt (0.26 mL, 1.53 mmol), and DMAP (10.0 mg, 0.082 mmol) were added to a solution of 4 (327.4 mg, 1.04 mmol) in CH2Cl2 (5.2 mL) at 0 oC under N2, and the resulting mixture was stirred at rt for 4 h. Sat. NaHCO3 aq. was added to the mixture, and the resulting solution was extracted with CH2Cl2. The organic layer was dried over Na2SO4 and evaporated in vacuo. The residue was purified by SiO2 column chromatography using hexane/ EtOAc = 4/1 (v/v) as an eluent to give 1f (357.2 mg, 79%).
Colorless oil; IR (KBr): 2865, 2250, 1714, 1271 cm-1; 1H NMR (400 MHz, CDCl3): δ 2.34-2.53 (2H, m), 3.55-3.65 (2H, m), 4.21-4.24 (1/3H, m), 4.35-4.40 (4/3H, m), 4.50-4.60 (13/3H, m), 6.59-6.62 (1H, m), 7.00-7.05 (1H, m), 7.24-7.37 (11H, m), 7.90-7.95 (4/3H, m), 8.02-8.06 (2/3H, m); 13C NMR (100 MHz, CDCl3): δ 38.1, 39.0, 70.0, 70.6, 71.3, 71.8, 73.3, 73.5, 78.7, 78.9, 84.2, 85.2, 99.3, 99.5, 115.4 (d, J = 22.0 Hz), 115.5 (d, J = 22.0 Hz), 126.2 (d, J = 2.9 Hz), 126.5 (d, J = 2.9 Hz), 127.55, 127.60, 127.66, 127.68, 127.72, 127.8, 128.33, 128.38, 128.41, 128.5, 132.2 (d, J = 9.5 Hz), 132.4 (d, J = 9.5 Hz), 137.7, 137.9, 138.0, 164.49, 164.50, 166.0 (d, J = 215.5 Hz); HRMS (FAB): calcd for C26H25O5FNa [M+Na]+ 459.1584, found 459.1586.
3,5-Di-O-benzyl-1-O-nitrobenzoyl-2-deoxy-D-ribofuranose (1g)
p-NO2BzCl (737.6 mg, 3.96 mmol), i-Pr2NEt (0.67 mL, 3.96 mmol), and DMAP (40.5 mg, 0.331 mmol) were added to a solution of 4 (1.04 g, 3.31 mmol) in CH2Cl2 (17 mL) at 0 oC under N2, and the resulting mixture was stirred at rt for 4 h. Sat. NaHCO3 aq. was added to the mixture, and the resulting solution was extracted with CH2Cl2. The organic layer was dried over Na2SO4 and evaporated in vacuo. The residue was purified by SiO2 column chromatography using hexane/EtOAc = 4/1 (v/v) as an eluent to give 1g (1.21 g, 79%).
Colorless oil; IR (KBr): 2359, 1728, 1526, 1348, 1273 cm-1; 1H NMR (400 MHz, CDCl3): δ 2.36-2.45 (3/2H, m), 2.50-2.55 (1/2H, m), 3.54-3.63 (2H, m), 4.24 (1/2H, dt, J = 4.8, 2.2 Hz), 4.36-4.39 (1/2H, m), 4.41-4.60 (5H, m), 6.60-6.64 (1H, m), 7.22-7.38 (10H, m), 8.01-8.04 (1H, m), 8.12-8.20 (3H, m); 13C NMR (100 MHz, CDCl3): δ 38.2, 39.0, 70.0, 70.3, 71.3, 71.9, 73.3, 73.5, 78.1, 78.8, 84.4, 85.5, 100.1, 123.36, 123.39, 127.50, 127.56, 127.66, 127.72, 127.8, 127.9, 128.3, 128.4, 128.5, 130.7, 130.9, 135.3, 135.6, 137.6, 137.75, 137.77, 137.81, 150.40, 150.44, 163.6, 164.0; HRMS (EI): calcd for C26H25NO7 [M]+ 463.1631, found 463.1644.
3,5-Di-O-benzyl-1-O- tert-butoxycarbonyl-2-deoxy-D-ribofuranose (1h)
Boc2O (0.24 mL, 1.11 mmol), NEt3 (0.31 mL, 2.24 mmol), and DMAP (10.0 mg, 0.082 mmol) were added to a solution of 4 (231.4 mg, 0.736 mmol) in CH2Cl2 (3.7 mL) at 0 oC under N2, and the resulting mixture was stirred at rt for 2 h. Water was added to the mixture, and the resulting solution was extracted with CH2Cl2. The organic layer was dried over Na2SO4 and evaporated in vacuo. The residue was purified by SiO2 column chromatography using hexane/EtOAc = 4/1 (v/v) as an eluent to give 1h (257.6 mg, 84%).
Colorless oil; IR (KBr): 2980, 1746, 1278, 1256 cm-1; 1H NMR (400 MHz, CDCl3): δ 1.46 (6H, s), 1.49 (3H, s), 2.20-2.42 (2H, m), 3.52-3.63 (2H, m), 4.10-4.13 (1/3H, m), 4.24-4.32 (4/3H, m), 4.41-4.44 (1/3H, m), 4.46-4.59 (4H, m), 6.21-6.23 (1H, m), 7.22-7.35 (10H, m); 13C NMR (100 MHz, CDCl3): δ 27.7, 27.8, 37.9, 38.5, 69.8, 70.9, 71.3, 71.7, 73.39, 73.44, 78.3, 78.8, 82.2, 82.5, 84.1, 84.4, 100.78, 100.82, 127.58, 127.60, 127.66, 127.68, 127.73, 128.33, 128.36, 128.39, 137.8, 137.9, 138.0, 138.1, 152.3, 152.8; HRMS (EI): calcd for C24H30O6 [M]+ 414.2042, found 414.2056.
3,5-Di-O-benzyl-2-deoxy-D-ribofuranosyl cyanide (2e)
Entry 1: This reaction was carried out using method B with 1e (35.3 mg, 0.084 mmol), CH2Cl2 (0.85 mL), 2,4,6-collidine (33 μL, 0.251 mmol), TMSOTf (31 μL, 0.172 mmol), TMSCN (53 μL, 0.424 mmol) and TBAT (227.0 mg , 0.420 mmol) to give 2e (6.2 mg, 23%, α : β = 5 :>95). Column chromatography: hexane/EtOAc = 4/1 (v/v)
Entry 2: This reaction was carried out using method B with 1f (49.4 mg, 0.113 mmol), CH2Cl2 (1.1 mL), 2,4,6-collidine (45 μL, 0.342 mmol), TMSOTf (41 μL, 0.227 mmol), TMSCN (71 μL, 0.568 mmol) and TBAT (305.4 mg , 0.566 mmol) to give 2e (9.3 mg, 25%, α : β = 5 : >95). Column chromatography: hexane/EtOAc = 4/1 (v/v)
Entry 4: This reaction was carried out using method B with 1h (37.1 mg, 0.090 mmol), CH2Cl2 (0.9 mL), 2,4,6-collidine (35 μL, 0.266 mmol), TMSOTf (32 μL, 0.177 mmol), TMSCN (56 μL, 0.448 mmol) and TBAT (241.6 mg , 0.448 mmol) to give 2e (13.6 mg, 44%, α : β = 17:83). Column chromatography: hexane/EtOAc = 4/1 (v/v)
Entry 5: This reaction was carried out using method B with 1h (35.3 mg, 0.085 mmol), CH2Cl2 (0.85 mL), 2,4,6-collidine (34 μL, 0.258 mmol), TESOTf (39 μL, 0.173 mmol), TMSCN (53 μL, 0.424 mmol) and TBAT (229.9 mg , 0.426 mmol) to give 2e (17.0 mg, 62%, α : β = 15:85). Column chromatography: hexane/EtOAc = 4/1 (v/v)
Experiments in Scheme 4
Compound 2f15 is a known compound.
Compound 1i was synthesized from a known compound 5.15
1-O-tert-Butoxycarbonyl-3,5-di-O-tert-butyldimethylsilyl-2-deoxy-D-ribofuranose (1i)
Boc2O (31 μL, 0.143 mmol), NEt3 (39 μL, 0.281 mmol), and DMAP (5.0 mg, 0.041 mmol) were added to a solution of 5 (33.9 mg, 0.093 mmol) in CH2Cl2 (0.5 mL) at 0 oC under N2, and the resulting mixture was stirred at rt for 2 h. Water was added to the mixture, and the resulting solution was extracted with CH2Cl2. The organic layer was dried over Na2SO4 and evaporated in vacuo. The residue was purified by SiO2 column chromatography using hexane/ EtOAc = 15/1 (v/v) as an eluent to give 1i (31.3 mg, 72%).
Colorless oil; IR (KBr): 2954, 1746, 1275, 1260 cm-1; 1H NMR (400 MHz, CDCl3): δ 0.04-0.10 (12H, m), 0.83-0.94 (18H, m), 1.48 (9H, s), 2.01-2.06 (4/10H, m), 2.11-2.17 (6/10H, m), 2.24-2.30 (6/10H, m), 2.35-2.41 (4/10H, m), 3.67-3.68 (2H, m), 3.87-3.91 (6/10H, m), 4.08-4.11 (4/10H, m), 4.31-4.35 (4/10H, m), 4.47-4.51 (6/10H, m), 6.10 (4/10H, dd, J = 5.4, 2.0 Hz), 6.17 (6/10H, dd, J = 5.6, 2.0 Hz); 13C NMR (100 MHz, CDCl3): δ -5.49, -5.46, -5.37, -5.32, -4.9, -4.8, -4.74, -4.70, 17.9, 18.28, 18.34, 25.7, 25.8, 25.9, 27.71, 27.74, 27.78, 40.8, 41.2, 62.4, 62.9, 70.8, 71.1, 81.9, 82.3, 87.6, 87.9, 100.3, 100.9, 152.6, 152.7; HRMS (FAB): calcd for C22H46O6Si2Na [M+Na]+ 485.2731, found 485.2719.
3,5-Di-O-tert-butyldimethylsilyl-2-deoxy-D-ribofuranosyl cyanide (2f)
This reaction was carried out using method B with 1i (31.2 mg, 0.067 mmol), CH2Cl2 (0.70 mL), 2,4,6-collidine (27 μL, 0.258 mmol), TESOTf (30 μL, 0.133 mmol), TMSCN (42 μL, 0.336 mmol) and TBAT (181.9 mg , 0.337 mmol) to give 2f (15.0 mg, 60%, α : β = 18:82). Column chromatography: hexane/EtOAc = 15/1 (v/v)
ACKNOWLEDGEMENTS
This work was financially supported by the Hoansha Foundation, the Uehara Memorial Foundation, and Granted-in-aid for scientific research and Platform for Drug Discovery, Informatics, and Structural Life Science from the Ministry of Education, Culture, Sports, Sciences, and Technology of Japan.
References
1. J. Clayden, N. Greeves, and S. Warren, 'Organic Chemistry' 2nd edn.; Oxford University Press, Inc., New York, 2012.
2. H. C. Kolb, M. G. Finn, and K. B. Sharpless, Angew. Chem. Int. Ed., 2001, 40, 2004. CrossRef
3. For example, see: A. Štimac and J. Kobe, Carbohydr. Res., 2000, 324, 149. CrossRef
4. (a) K. Haraguchi and M. M. Greenberg, J. Am. Chem. Soc., 2001, 123, 8636; CrossRef (b) K. Haraguchi, M. O. Delaney, C. J. Wiederholt, A. Sambandam, Z. Hantosi, and M. M. Greeenberg, J. Am. Chem. Soc., 2002, 124, 3263. CrossRef
5. H. Togo, S. Ishigami, M. Fujii, T. Ikuma, and M. Yokoyama, J. Chem. Soc., Perkin Trans. 1, 1994, 2931. CrossRef
6. A. Štimac and J. Kobe, Carbohydr. Res., 2000, 329, 317. CrossRef
7. T. J. Miller, H. D. Farquar, A. Sheybani, C. E. Tallini, A. S. Saurage, F. R. Fronczek, and R. P. Hammer, Org. Lett., 2002, 4, 877. CrossRef
8. H. Fujioka, Y. Minamitsuji, T. Moriya, K. Okamoto, O. Kubo, T. Matsushita, and K. Murai, Chem. Asian J., 2012, 7, 1925. CrossRef
9. Ca. 1/1 to 2/1 diasetereomeric mixture of compounds 1 at the anomeric position was used.
10. The structure of the regenerated 1a was confirmed by 1H NMR.
11. 1H NMR samples of the salts were prepared according to the General Procedure for the Introduction of an Azido Group to the 2-Deoxyribose Derivatives (Method A) using CD2Cl2 in place of CH2Cl2, and recorded on a JEOL JNM-LA 500 at 20 oC.
12. X. F. Bravo, M. Kassou, Y. Díaz, and S. Castillón, Carbohydr. Res., 2001, 336, 83. CrossRef
13. W. Wieranga and H. I. Skulnick, Carbohydr. Res., 1981, 90, 41. CrossRef
14. J. S. Yadav, B. V. S. Reddy, A. S. Reddy, C. S. Reddy, and S. S. Raju, Tetrahedron Lett., 2009, 50, 6631. CrossRef
15. J. H. H. Rothman, J. Org. Chem., 2007, 72, 3945. CrossRef
16. V. I. Mukhanov, M. N. Presbrazhenskaya, and N. N. Suvorov, Zh. Org. Khim., 1974, 10, 666.
17. G. Seitz and J. Z. Lachmann, Naturforsch., B: Chemical Sci., 1999, 54, 549.