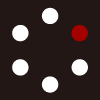
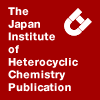
HETEROCYCLES
An International Journal for Reviews and Communications in Heterocyclic ChemistryWeb Edition ISSN: 1881-0942
Published online by The Japan Institute of Heterocyclic Chemistry
e-Journal
Full Text HTML
Received, 5th August, 2014, Accepted, 2nd October, 2014, Published online, 14th October, 2014.
DOI: 10.3987/COM-14-S(K)103
■ Stereoselective Aza-Henry Reaction of Chiral tert-Butanesulfinyl Imines with Methyl or Ethyl 4-Nitrobutanoate: Easy Access to Enantioenriched 6-Substituted Piperidine-2,5-diones
M. Jesús García-Muñoz, Francisco Foubelo,* and Miguel Yus*
Department of Organic Chemistry, Faculty of Sciences and Institute of Organic Synthesis (ISO), University of Alicante, P.O. Box 99, 03080 Alicante, Spain
Abstract
The base-catalyzed addition of 4-nitrobutanoates 6 to N-tert-butanesulfinyl imines 8 under solvent-free reaction conditions proceeded with high face diastereoselectivity. The resulting β-nitroamine derivatives 9 were easily transformed into 6-substituted piperidine-2,5-diones 11 upon removal of the sulfinyl group with concomitant δ-lactam formation and functional group transformation under Nef reaction conditions.Substituted piperidine-2,5-diones are versatile building blocks in organic synthesis because they can be transformed very easily into substituted piperidin-3-ols with high control of the stereochemistry. It is known that the piperidine ring is widely represented in many natural products and can exhibit broad range of biological activities.1 Particularly, systems with the piperidin-2-ol skeleton having substituents at 2-position2 and 6-position3 are of great pharmacological interest. For instance, (+)-febrifugine (1) is a natural product isolated from Dichroa febrifuga,4 a plant used in traditional Chinese medicine to treat malaria (Figure 1). A synthetic propanoic acid derivative 2, with a 3-hydroxyl-2-piperidinyl unit, showed a potent in vitro activity as a GABA receptor binder, similar to that displayed by baclofen.5 Several 2,6-disubstituted piperidin-3-ols have been isolated from the African savanna plant, Prosopis africana. Among them, (+)-prosophylline (3) displays antibiotic and anesthetic properties (Figure 1).6
Most of the enantioselective syntheses of substituted piperidin-3-ols reported until today are based on the chiral pool synthesis using amino acid and sugar derivatives as starting materials.7 Other stereoselective syntheses included as key steps nucleophilic additions of organomagnesium compounds to chiral oximes8 and enzymatic resolution of racemic compounds.2,9 Continuing our interest in the use of N-tert-butanesulfinyl imines10 as electrophiles, we envisioned a straightforward synthesis of 6-substituted piperidin-2,5-diones, based on the diastereoselective aza-Henry reaction of methyl or ethyl 4-nitrobutanoate and these chiral imines.11 Our retrosynthentic analysis for the preparation of target 6-substituted piperidin-2,5-diones is depicted on Scheme 1.
Methyl and ethyl 4-nitrobutanoate (6a and 6b, respectively) were prepared in reasonable yields (42 and 47%, respectively) from the corresponding acrylate 4 and an excess of nitromethane (5) in the presence of 0.1 equivalents of sodium hydroxide at 0 to 23 ºC for 12 h. The reaction is performed without an additional solvent. In spite of working with an excess of nitromethane (5), compounds resulting from double conjugate addition 7 were also isolated as undesirable side reaction products (Scheme 2).
As depicted in Scheme 1, our approach to the synthesis of target 6-substituted piperidine-2,5-diones commenced with the diastereoselective aza-Henry reaction of methyl or ethyl 4-nitrobutanoate (6a or 6b, respectively) with N-tert-butanesulfinyl imines 8. These chiral imines 8 were easily accessible from (R)-tert-butanesulfinamide and the corresponding aldehyde.12 First, imine 8a (derived from 3-phenylpropanal) was taken as the model compound in order to determine the optimal reaction conditions to carry out the coupling of nitro compounds 6 with chiral imines 8. Sodium bicarbonate has recently been used successfully in our group as a promoter of the condensation of nitromethane (5) and nitroethane with sulfinyl imines 8.10t The reaction of 3 equivalents of methyl 4-nitrobutanoate (6a) with 8a in the presence of 2 equivalents of sodium bicarbonate at 60 ºC for 3 days led to the expected aza-Henry product 9aa in relatively low yield (Table 1, entry 1). Ethyl 4-nitrobutanoate (6b), under the same reaction conditions, produced 9ab even in lower yield (Table 1, entry 2). Very low yield was also obtained when the reaction was performed under sonication for 7 h, using the same reaction mixture of 6a, 8a and sodium bicarbonate (Table 1, entry 3). Interestingly, yield was significantly improved (from 37 to 80%) when the coupling of 6a and 8a was performed in the presence of 0.2 equivalents of sodium hydroxide as a base at 40 °C for 24 h (Table 1, entry 4). Fortunately, the sodium hydroxide promoted reaction of imine 8a with ethyl 4-nitrobutanoate (6b) led to expected adduct 9ab in a satisfactory 76% isolated yield (Table 1, entry 5). In order to broaden the scope of the sodium hydroxide promoted aza-Henry reaction, we applied the optimized conditions depicted in Table 1, entries 4 and 5, to N-tert-butanesulfinyl imines 8b and 8c. Thus, the reaction of imine 8b derived from isovaleraldehyde with methyl and ethyl 4-nitrobutanoate (6) gave compounds 9ba and 9bb in 69 and 50% yield, respectively (Table 1, entries 6 and 7). Meanwhile, the reactions of nitro compounds 6 with chiral imine 8c derived from nonanal produced the expected adducts 9ca and 9cb in near 50% yield (Table 1, entries 8 and 9). In these reactions, two stereogenic centers are generated, and diastereomeric ratios shown on Table 1 refer to the face selectivity. Regarding the second stereogenic center, an almost 1:1 mixture of epimers were always obtained, due probably to the reaction conditions (prolonged reaction times in the presence of a base), considering that an acidic proton remains in 9, so epimerization could take place rapidly. Nucleophilic additions of nitro compounds 6 to chiral imines 8 takes place with relatively high diastereoselectivity, diastereomeric ratios ranging in all cases from 87:13 (Table 1, entry 5) to 98:2 (Table 1, entry 6). The stereochemical pathway of these nucleophilic additions to chiral sulfinyl imines was previously studied in depth by us10t and others.13 It was always found that in the case of chiral imines 8 with R configuration at the sulphur atom, the attack of the nucleophile occurs predominantly to the Si-face of the imine, so the configuration assigned to the newly created stereogenic center bonded to the nitrogen atom in compounds 9 is based in this assumption.
Compounds of type 9 were previously prepared by García Ruano, Cid and co-workers, following a slightly different strategy. They performed first the nucleophilic addition of nitromethane (5) to a chiral N-p-toluenesulfinyl imine, and subsequently, the DBU-promoted Michael addition of the resulting β-nitroamino derivative to the corresponding acrylate. These compounds were later transformed into the 6-substituted 5-nitropiperidin-2-ones as shown in Scheme 3.14
We found also of interest, to reach compounds 9 through a one-pot multicomponent assembling of nitromethane (5), chiral imines 8 and acrylates 4. Benefits of the multicomponent processes use to include decreased operational costs associated with time and labor. For that reason we studied first this reaction with chiral aldimine 8a as a model imine. Different reaction conditions were applied (stoichiometry, temperature, reaction time, base, reagents addition order, solvent) and the highest yield of the expected adducts 9aa and 9ab were obtained when the imine 8a reacted first with 3 equivalents of nitromethane (5) in the presence of 0.5 equivalents of sodium hydroxide at 40 ºC for 24 h, and after that, 4 equivalents of the corresponding acrylate 4 were added maintaining the reaction mixture at the same temperature for 24 additional hours. The methyl ester derivative 9aa was obtained in 30% yield and the ethyl derivative 9ab in 35% yield (Scheme 4). The here described one-pot methodology does not seem to be superior to the multistep strategy previously commented.
Removal of the tert-butanesulfinyl group in compounds 9 was achieved under acidic conditions. Further treatment of the initially formed ammonium chloride salt with an aqueous saturated solution of sodium bicarbonate produced the free amine, which underwent rapid intramolecular cyclization to give 6-substituted 5-nitropiperidin-2-one 10 as a mixture of diastereoisomers in an almost 3:1 ratio, trans-isomer being the major component of the mixture. Pure diastereoisomers were obtained after column chromatography purification. In general, yields were slightly higher in the case of the methyl ester derivatives (Table 2). Finally, compounds 10, as a diastereomeric mixture, were transformed into piperidine-2,5-diones 11 under typical Nef reaction conditions. First treatment with sodium methoxide in methanol to generate the corresponding nitronate at room temperature, followed by addition of a methanolic solution of sulfuric acid and final hydrolysis at low temperature. After that, the expected dione 11 was formed along with the corresponding dimethyl acetal, since the protonation of the nitronate was performed in a mixture of methanol and water. A second hydrolysis at 100 ºC in water under acidic conditions of the residue resulting after removing of volatile solvents gave the expected piperidine-2,5-dione 11 as a single isomer (Table 2).
In summary, enantioenriched 6-substituted piperidine-2,5-diones 11 were prepared in few synthetic operations from commercially available starting materials. The methodology presented here comprised as key steps a diastereoselective addition of a 4-nitrobutanoate to a chiral sulfinyl imine, and a Nef reaction for the transformation of the nitro group into ketone. Studies are currently in progress trying to find reaction conditions for the stereoselective transformation of 6-substituted piperidine-2,5-diones 11 into 2-substituted piperidin-3-ols 12 and 2,6-disubstituted piperidin-3-ols 13 (Scheme 5), compounds of potential biological activity.
EXPERIMENTAL
All chemicals were commercially available (Acros, Aldrich). TLC was performed on Merck silica gel 60 F254, using aluminum plates and visualized with phosphomolybdic acid (PMA) stain. Chromatographic purification was performed by flash chromatography using Merck silica gel 60 (0.040-0.063 mm) and hexane/EtOAc as eluent. Low-resolution electron impact (EI) mass spectra were obtained at 70eV on Agilent GC/MS-5973N apparatus equipped with a HP-5MS column (Agilent technologies, 30 m × 0.25 mm) and high resolution mass spectra (HRMS-ESI) were obtained on a Waters LCT Premier XE apparatus equipped with a time of flight (TOF) analyzer and the samples were ionized by ESI techniques and introduced through an ultra-high pressure liquid chromatograph (UPLC) model Waters ACQUITY H CLASS. IR spectra were measured (film) with a Nicolet Impact 510 P-FT Spectrometer. NMR spectra were recorded with a Bruker AC-300 using CDCl3 as the solvent and TMS as internal standard. Optical rotations were measured on a Perkin Elmer 341 polarimeter. Starting aldimines 8a,15 8b16 and 8c17 were prepared according to the procedures reported in the literature from (R)-tert-butanesulfinamide and the corresponding aldehyde.
Preparation of 4-nitrobutanoates 6. General procedure.
To a solution of NaOH (20 g, 0.5 mmol) in nitromethane (1.525 g, 1.3 mL, 25 mmol) was added the corresponding acrylate 4 (5 mmol) at 0 ºC. The reaction mixture was stirred for 12 h and the system was allowed to reach room temperature. Then, the resulting mixture was hydrolyzed with H2O (20 mL) and extracted with EtOAc (3 × 15 mL). The organic layer was washed with brine (2 × 10 mL), dried over anhydrous MgSO4 and evaporated (15 Torr). The resulting residue was purified by distillation under vacuum to give pure products 6. Yields are given in Scheme 2. Physical and spectroscopic data follow.
Methyl 4-nitrobutanonate (6a).18 Colourless oil; bp 120-122 ºC (2,4 mbar); Rf 0.45 (hexane/EtOAc: 3/1); IR ν (film) 2991, 2951, 1731, 1548, 1436, 1372, 1200, 1171 cm-1; 1H NMR (300 MHz, CDCl3) δ 4.49 (2H, t, J = 6.6 Hz, CH2), 3.71 (3H, s, Me), 2.48 (2H, t, J = 6.8 Hz, CH2), 2.40-2.21 (2H, m, CH2); 13C NMR (75 MHz, CDCl3) δ 172.3 (C), 74.3 (CH2), 51.9 (Me), 30.2, 22.3 (CH2); LRMS (EI) m/z 116 (M+-MeO, 35%), 100 (9), 88 (11), 69 (10), 59 (100).
Ethyl 4-nitrobutanonate (6b).19 Colourless oil; bp 123-125 ºC (2,4 mbar); Rf 0.46 (hexane/EtOAc: 3/1); IR ν (film) 2983, 2946, 2908, 1728, 1550, 1435, 1376, 1177, 1027 cm-1; 1H NMR (300 MHz, CDCl3) δ 4.49 (2H, t, J = 6.6 Hz, CH2), 4.16 (2H, q, J = 7.1 Hz, CH2), 2.47 (2H, t, J = 6.8 Hz, CH2), 2.32 (2H, quint, J = 6.8 Hz, CH2), 1.27 (3H, t, J = 7.1 Hz, Me); 13C NMR (75 MHz, CDCl3) δ 171.8 (C), 74.3, 60.9, 30.5, 22.4 (CH2), 14.1 (Me); LRMS (EI) m/z 116 (M+-MeO, 35%), 100 (9), 88 (11), 69 (10), 59 (100).
Preparation of β-nitroamine derivatives 9 from nitrobutanoates 6 and chiral imines 8. General procedure.
A mixture of the corresponding nitrocompound 6 (6.0 mmol), NaOH (16 mg, 0.4 mmol), and the corresponding N-tert-butanesulfinyl imine 8 (2.0 mmol) was stirred at 40 ºC for 24 h. The resulting mixture was hydrolyzed with H2O (15 mL) and extracted with EtOAc (3 × 15 mL). The organic layer was washed with brine (2 × 10 mL), dried over anhydrous MgSO4, and evaporated (15 Torr). The residue was purified by column chromatography (silica gel) to yield pure compounds 9. Yields are given in Table 1. Physical and spectroscopic data follow.
(4R*,5R,RS)-Methyl N-(tert-butanesulfinyl)-5-amino-4-nitro-7-phenylheptanoate (9aa). Mixture of diastereoisomers (1:1); yellow oil; Rf 0.31 (hexane/EtOAc: 1/1); IR ν (film) 2947, 2927, 2868, 1734, 1546, 1454, 1437, 1364, 1174, 1052, 749, 700 cm-1; 1H NMR (300 MHz, CDCl3) δ 7.35-7.10 (10H, m, ArH), 5.00-4.91 (1H, m, CH), 4.90-4.82 (1H, m, CH), 4.32 (1H, d, J = 8.6 Hz, NH), 4.27 (1H, d, J = 9.5 Hz, NH), 3.69 (3H, s, Me), 3.68 (3H, s, Me), 3.64-3.46 (2H, m, CH2), 2.97-2.77 (2H, m, CH2), 2.77-2.56 (2H, m, CH2), 2.54-2.19 (8H, m, 4 × CH2), 1.95-1.78 (4H, m, 2 × CH2) , 1.31 [9H, s, Me3C], 1.28 [9H, s, Me3C]; 13C NMR (75 MHz, CDCl3) δ 172.3, 140.2 (C), 128.6, 128.4, 128.3, 126.3, 90.6, 89.6, 58.5, 57.8 (CH), 56.8, 56.7 (C), 51.9 (Me), 35.6, 32.4, 32.0, 31.8, 30.2, 29.6, 26.1, 25.0 (CH2), 22.8, 22.7 (Me); LRMS (EI) m/z 264 [M+-Ph(CH2)2-Me, 33%], 232 (11), 231 (16), 218 (25), 217 (58), 207 (12), 185 (27), 183 (19), 158 (15), 143 (37), 141 (16), 139 (28), 133 (21), 132 (29), 129 (22), 128 (20), 115 (26), 91 (100), 77 (20), 65 (16), 55 (21); HRMS (ESI) calcd for C18H29N2O5S (M+H) 385.1797, found 385.1791.
(4R*,5R,RS)-Ethyl N-(tert-butanesulfinyl)-5-amino-4-nitro-7-phenylheptanoate (9ab). Mixture of diastereoisomers (1:1); yellow oil; Rf 0.28 (hexane/EtOAc: 1/1); IR ν (film) 2981, 2958, 2918, 1731, 1547, 1455, 1368, 1241, 1182, 1047, 700 cm-1; 1H NMR (300 MHz, CDCl3) δ 7.36-7.07 (10H, m, ArH), 4.93 (1H, ddd, J = 9.0, 6.2, 2.5 Hz, CH), 4.87 (1H, dt, J = 6.9, 4.2 Hz, CH), 4.31 (1H, d, J = 8.6 Hz, NH), 4.24 (1H, d, J = 9.5 Hz, NH), 4.13 (2H, q, J = 7.1 Hz, CH2), 4.12 (2H, q, J = 7.1 Hz, CH2), 3.66-3.43 (2H, m, 2 × CH), 2.95-2.74 (2H, m, CH2), 2.76-2.53 (2H, m, CH2), 2.52-2.15 (7H, m, 3 × CH2, CHH), 2.14-1.97 (1H, m, CHH), 1.95-1.77 (3H, m, CH2, CHH), 1.73-1.58 (1H, m, CHH), 1.30 [9H, s, Me3C], 1.27 [9H, s, Me3C], 1.24 (6H, t, J = 7.2 Hz, 2 × Me); 13C NMR (75 MHz, CDCl3) δ 171.9, 140.3 (C), 128.6, 128.4, 128.3, 126.3, 90.6, 89.6 (CH), 60.9, 60.8 (CH2), 58.5, 57.8 (CH), 56.8, 56.6 (C), 35.7, 32.4, 32.0, 31.8, 30.4, 29.7, 26.1, 25.0 (CH2), 22.8, 22.7, 14.1 (Me); LRMS (EI) m/z 313 [M+-Me2C=CH2-Et, 1%], 182 (47), 157 (20), 144 (14), 143 (100), 141 (25), 129 (32), 128 (28), 115 (20), 111 (13), 91 (67); HRMS (ESI) calcd for C19H31N2O5S (M+H) 399.1954, found 399.1941.
(4R*,5R,RS)-Methyl N-(tert-butanesulfinyl)-5-amino-7-methyl-4-nitrooctanoate (9ba). Mixture of diastereoisomers (1:1); yellow oil; Rf 0.30 (hexane/EtOAc: 1/1); IR ν (film) 3193, 2957, 2929, 2870, 1736, 1548, 1438, 1173, 1028 cm-1; 1H NMR (300 MHz, CDCl3) δ 4.99-4.90 (1H, m, CH), 4.86-4.77 (1H, m, CH), 4.19 (1H, d, J = 9.1 Hz, NH), 4.15 (1H, d, J = 9.9 Hz, NH), 3.71 (3H, s, Me), 3.70 (3H, s, Me), 3.67-3.56 (2H, m, CH), 2.60-2.34 (6H, m, 3 × CH2), 2.33-2.20 (1H, m, CHH), 2.20-2.09 (1H, m, CHH), 1.88-1.70 (2H, m, CH2), 1.55-1.42 (2H, m, CH2), 1.34-1.28 (2H,m, 2 × CH), 1.26 [9H, s, Me3C], 1.25[9H, s, Me3C], 0.93 (3H, d, J = 0.9 Hz, Me), 0.92 (3H, d, J = 0.9 Hz, Me), 0.90 (3H, d, J = 1.9 Hz, Me), 0.89 (3H, d, J = 1.9 Hz, Me); 13C NMR (75 MHz, CDCl3) δ 172.4, 172.3 (C), 91.1, 90.0, 57.8 (CH), 56.8 (C), 56.7 (CH), 56.6 (C), 52.0, 51.9 (Me), 42.6, 39.3, 30.5, 29.7, 26.0, 25.0 (CH2), 24.3, 24.2 (CH), 23.4, 23.0, 22.8, 22.7, 21.3, 21.0 (Me); LRMS (EI) m/z 280 (M+-C4H8, 1%), 153 (29), 143 (15), 138 (11), 111 (100), 97 (83), 84 (13), 83 (19), 82 (19), 69 (18), 55 (27); HRMS (ESI) calcd for C14H29N2O5S (M+H) 337.1797, found 337.1790.
(4R*,5R,RS)-Ethyl N-(tert-butanesulfinyl)-5-amino-7-methyl-4-nitrooctanoate (9bb). Mixture of diastereoisomers (1:1); yellow oil; Rf 0.31 (hexane/EtOAc: 1/1); IR ν (film) 3218, 2958, 2870, 1732, 1546, 1365, 1181, 1051 cm-1; 1H NMR (300 MHz, CDCl3) δ 5.00-4.90 (1H, m, CH), 4.88-4.78 (1H, m, CH), 4.25-4.78 (6H, m, 2 × NH, 2 × CH2), 3.71-3.55 (2H, m, 2 × CH), 2.62-2.18 ( 10H, m, 5 × CH2), 1.87-1.72 (2H, m, 2 × CH), 1.56-1.38 (2H, m, CH2), 1.35-1.16 [24H, m, 2 × Me3C, 2 × Me] 1.01-0.84 (12H, 4 × Me); 13C NMR (75 MHz, CDCl3) δ 172.0, 171.9 (C), 91.1, 89.9 (CH), 60.9, 60.8 (CH2), 57.7 (CH), 56.7 (C), 56.6 (CH), 56.5 (C), 42.5, 39.3, 30.6, 29.9, 26.0, 25.0 (CH2), 24.2, 24.1 (CH), 23.3, 23.0, 22.7, 22.6, 21.2, 21.0, 14.1 (Me); LRMS (EI) m/z 335 (M+-Me, 1%), 216 (38), 185 (10), 170 (56), 169 (71), 157 (20), 141 (100), 124 (13), 123 (94), 113 (47), 111 (29), 99 (44), 95 (40), 71 (67), 67 (37), 60 (16), 55 (53); HRMS (ESI) calcd for C15H31N2O5S (M+H) 351.1954, found 351.1950.
(4R*,5R,RS)-Methyl N-(tert-butanesulfinyl)-5-amino-4-nitrotridecanoate (9ca). Mixture of diastereoisomers (1:1); pale yellow oil; Rf 0.40 (hexane/EtOAc: 1/1); IR ν (film) 3242, 2953, 2925, 2925, 2855, 1736, 1547, 1056, 733 cm-1; 1H NMR (300 MHz, CDCl3) δ 4.97-4.78 (2H, m, 2 × CH), 4.23 (1H, d, J = 8.7 Hz, NH), 4.14 (1H, d, J = 8.3 Hz, NH), 3.71 (3H, s, Me), 3.71 (3H, s, Me), 3.64-3.46 (2H, m, 2 × CH), 2.61-2.15 (8H, m, 4 × CH2), 1.61-1.44 (8H, m, 4 × CH2), 1.40-1.14 [38H, m, 10 × CH2, 2 × Me3C], 0.89 (6H, t, J = 6.6 Hz, 2 × Me); 13C NMR (75 MHz, CDCl3) δ 172.4 (C), 90.8, 89.7, 59.4, 58.6 (CH), 56.7, 56.6 (C), 52.0, 51.9 (Me), 33.8, 31.8, 30.4, 29.7, 29.3, 29.1, 29.0, 26.1, 25.9, 25.8 (CH2), 22.8, 22.7 (Me), 22.6 (CH2), 14.05 (Me); LRMS (EI) m/z 317 (M+-C2H3O3, 3%), 270 (17), 256 (16), 238 (19), 224 (16), 208 (21), 196 (17), 187 (19), 140 (17), 110 (34), 97 (33), 96 (57), 95 (24), 85 (16), 83 (44), 82 (100), 81 (33), 80 (17), 79 (17), 71 (20), 69 (42), 68 (30), 67 (42), 64 (46), 59 (35), 57 (55), 56 (44), 55 (82), 54 (67), 53 (25); HRMS (ESI) calcd for C18H37N2O5S (M+H) 393.2423, found 393.2418.
(4R*,5R,RS)-Ethyl N-(tert-butanesulfinyl)-5-amino-4-nitrotridecanoate (9cb). Mixture of diastereoisomers (1:1); colourless oil; Rf 0.41 (hexane/EtOAc: 1/1); IR ν (film) 2957, 2925, 2856, 1734, 1547, 1365, 1181, 1053 cm-1; 1H NMR (300 MHz, CDCl3) δ 4.97-4.78 (2H, m, CH), 4.27-4.02 (6H, m, 2 × NH, 2 × CH2), 3.61-3.46 (2H, m, 2 × CH), 2.59-2.18 (10H, m, 5 × CH2), 1.64-1.41 (6H, m, 3 × CH2), 1.38-1.13 [44H, 10 × CH2, 2 × Me, 2 × Me3C], 0.88 (6H, t, J = 6.7 Hz, 2 × Me); 13C NMR (75 MHz, CDCl3) δ 171.9 (C), 90.8, 89.6 (CH), 60.9, 60.8 (CH2), 59.3, 58.5 (CH), 56.6, 56.5 (C), 33.7, 31.7, 29.8, 29.3, 29.2, 29.1, 29.0, 28.9, 26.1, 25.8, 25.7, 25.0 (CH2), 23.0, 22.7, 22.6 (Me), 22.5 (CH2), 14.1, 14.0 (Me); LRMS (EI) m/z 335 (M+-C4H8-Me, 1%), 187 (18), 170 (11), 143 (20), 140 (20), 129 (50), 113 (19), 110 (24), 100 (49), 97 (30), 96 (43), 95 (31), 90 (24), 83 (35), 82 (42), 81 (42), 71 (22), 70 (14), 69 (55), 68 (17), 67 (39), 64 (36), 63 (18), 57 (63), 56 (37), 55 (100), 54 (27), 53 (16); HRMS (ESI) calcd for C19H39N2O5S (M+H) 407.2580, found 407.2573.
Preparation of β-nitroamine derivatives 9aa and 9ab from nitromethane 5, acrylates 4 and chiral imine 8a. General procedure.
A mixture of NaOH (6 mg, 0.15 mmol), nitromethane (55 mg, 0.049 mL, 0.9 mmol) and the chiral imine 8a (71 mg, 0.3 mmol) was stirred at 40 ºC for 24 h. After that, the excess of nitromethane (5) is removed under vacuum (15 Torr). To the resulting residue the corresponding acrylate 4 (1.2 mmol) was added and the reaction mixture was stirred at 40 ºC for additional 24 h. Then, the resulting mixture was hydrolyzed with H2O (10 mL) and extracted with EtOAc (3 × 10 mL). The organic layer was washed with brine (2 × 10 mL), dried over anhydrous MgSO4 and evaporated (15 Torr). The residue was purified by column chromatography (silica gel, hexane/EtOAc) to yield pure compounds 9aa and 9ab. Yields are given in Scheme 4. Physical and spectroscopic data are given above.
Preparation of 6-substituted-5-nitropiperidin-2-ones 10. General procedure.
To a solution of the corresponding β-nitroamino derivative 9 (0.5 mmol) in THF (0.5 mL) was added dropwise a 6M solution of HCl (0.25 mL, 1.5 mmol) at 0 ºC. The reaction mixture was stirred at this temperature for 4 h and after that basified with saturated aqueous solution of NaHCO3. Then stirring was continued at room temperature for 20 min. Then, the resulting mixture was extracted with EtOAc (3 × 10 mL). The organic layer was washed with brine (2 × 10 mL), dried over anhydrous MgSO4 and evaporated (15 Torr). The residue was purified by column chromatography (silica gel, hexane/EtOAc) to yield pure compounds 10. Yields are given in Table 2. Physical and spectroscopic data follow.
(5S,6R)-5-Nitro-6-(2-phenylethyl)piperidin-2-one trans-(10a). White solid; mp 110-112 ºC (hexane/CH2Cl2); [α]20D +71 (c 0.79, CH2Cl2); Rf 0.25 (hexane/EtOAc: 1/2); IR ν (KBr) 3182, 3025, 2918, 2858, 1656, 1555, 1348, 1349, 802, 747, 698 cm-1; 1H NMR (300 MHz, CDCl3) δ 7.41-7.08 (5H, m, ArH), 6.30 (1H, s, NH), 4.71-4.47 (1H, m, CH), 4.25-3.97 (1H, m, CH), 2.91-2.75 (1H, m, CHH), 2.76-2.63 (1H, m, CHH), 2.63-2.41 (3H, m, CH2, CHH), 2.41-2.25 (1H, m, CHH), 2.00-1.82 (2H, m, CH2); 13C NMR (75 MHz, CDCl3) δ 169.7, 139.4 (C), 128.9, 128.2, 126.7, 82.7, 54.0 (CH), 36.3, 31.2, 27.7, 23.8 (CH2); LRMS (EI) m/z 248 (M+, 0.5%), 202 (22), 201 (100), 143 (13), 105 (13), 97 (59), 92 (16), 91 (96), 69 (11), 65 (11), 55 (14); HRMS (ESI) calcd for C13H17N2O3 (M+H) 249.1239, found 249.1227.
(5R,6R)-5-Nitro-6-(2-phenylethyl)piperidin-2-one cis-(10a). White solid; mp 108-113 ºC (hexane/CH2Cl2); [α]20D +85 (c 1.04, CH2Cl2); Rf 0.12 (hexane/EtOAc: 1/2); IR ν (KBr) 3187, 3070, 2941, 2922, 1655, 1540, 1405, 747, 704 cm-1; 1H NMR (300 MHz, CDCl3) δ 7.40-7.14 (5H, m, ArH), 6.84 (1H, s, NH), 4.87 (1H, dd, J = 9.7, 4.5 Hz, CH), 3.86-3.68 (1H, m, CH), 2.94-2.60 (3H, m, CH2, CHH), 2.60-2.38 (2H, m, CH2), 2.30-2.14 (1H, m, CHH), 1.89 (2H, dd, J = 14.8, 7.6 Hz, CH2); 13C NMR (75 MHz, CDCl3) δ 170.7, 139.5 (C), 128.7, 128.3, 126.6, 80.8, 53.0 (CH), 33.2, 31.4, 27.2, 22.9 (CH2); LRMS (EI) m/z 248 (M+, 1%), 202 (24), 201 (100), 143 (16), 105 (11), 97 (63), 92 (14), 91 (93), 69 (14), 55 (17); HRMS (ESI) calcd for C13H17N2O3 (M+H) 249.1239, found 249.1229.
(5S,6R)-6-Isobutyl-5-nitropiperidin-2-one trans-(10b). White solid; mp 127-129 ºC (hexane/CH2Cl2); [α]20D +49 (c 0.78, CH2Cl2); Rf 0.21 (hexane/EtOAc: 1/3); IR ν (KBr) 3193, 3074, 2957, 2927, 2868, 1657, 1555, 1406, 1375, 1353, 779 cm-1; 1H NMR (300 MHz, CDCl3) δ 6.48 (1H, s, NH), 4.51 (1H, dt, J = 6.8, 4.8 Hz, CH), 4.13 (1H, ddd, J = 9.8, 6.2, 2.0 Hz, CH), 2.63-2.50 (3H, m, CH2, CHH), 2.50-2.41 (1H, m, CHH), 2.39-2.28 (1H, m, CHH), 1.83-1.63 (1H, m, CH), 1.54 (1H, ddd, J = 14.5, 9.3, 5.3 Hz, CHH), 1.37 (1H, m, J = 13.7, 9.2, 4.4 Hz, CHH), 0.98 (3H, d, J = 6.6 Hz, Me), 0.95 (3H, d, J = 6.6 Hz, Me); 13C NMR (75 MHz, CDCl3) δ 170.0 (C), 83.3, 52.5 (CH), 43.8, 27.6 (CH2), 24.2 (CH), 23.6 (CH2), 23.1, 21.3 (Me); LRMS (EI) m/z 157 (M+-C3H7, 1%), 153 (44), 138 (16), 112 (13), 111 (100), 97 (96), 83 (15), 82 (15), 69 (13), 55 (16); HRMS (ESI) calcd for C9H17N2O3 (M+H) 201.1239, found 201.1229.
(5S,6R)-5-Nitro-6-octylpiperidin-2-one trans-(10c). White solid; mp 96-99 ºC (hexane/CH2Cl2); [α]20D +44 (c 0.93, CH2Cl2); Rf 0.38 (hexane/EtOAc: 1/3); IR ν (KBr) 3203, 2919, 2848, 1656, 1556, 1405, 1352, 793, 626 cm-1; 1H NMR (300 MHz, CDCl3) δ 6.49 (1H, s, NH), 4.56 (1H, dt, J = 11.1, 5.5 Hz, CH), 4.06 (1H, td, J = 7.6, 1.8 Hz, CH), 2.63-2.40 (3H, m, CH2, CHH), 2.39-2.28 (1H, m, CHH), 1.61-1.56 (2H, m, CH2), 1.48-1.44 (1H, m, CHH), 1.37-1.27 (11H, m, CHH, 5 × CH2), 0.88 (3H, t, J = 6.9 Hz, Me); 13C NMR (75 MHz, CDCl3) δ 170.0, 82.8, 54.5 (CH), 34.5, 31.7, 29.2, 29.1, 29.05, 27.6, 24.8, 23.8, 22.6 (CH2), 14.0 (Me); LRMS (EI) m/z 210 (M+-NO2, 7%), 143 (13), 124 (37), 111 (100), 97 (52), 83 (13), 82 (13), 56 (22); HRMS (ESI) calcd for C13H25N2O3 (M+H) 257.1865, found 257.1853.
(5R,6R)-5-Nitro-6-octylpiperidin-2-one cis-(10c). White solid; mp 99-103 ºC (hexane/CH2Cl2); [α]20D +84 (c 0.44, CH2Cl2); Rf 0.23 (hexane/EtOAc: 1/3); IR ν (KBr) 3198, 2918, 2850, 1660, 1537, 1411, 1346, 807 cm-1; 1H NMR (300 MHz, CDCl3) δ 6.35 (1H, s, NH), 4.98-4.80 (1H, m, CH), 3.88-3.70 (1H, m, CH), 2.85-2.61 (1H, m, CHH), 2.57-2.40 (2H, m, CH2), 2.28 (1H, ddd, J = 15.6, 9.7, 7.4 Hz, CHH), 1.64-1.48 (2H, m, CH2), 1.46-1.14 (12H, m, CHH, 6 × CH2), 0.88 (3H, t, J = 6.9 Hz, Me); 13C NMR (75 MHz, CDCl3) δ 170.6, 80.7, 54.1 (CH), 31.7, 31.6, 29.2, 29.1, 29.05, 27.2, 25.5, 23.0, 22.6 (CH2), 14.05 (Me); LRMS (EI) m/z 210 (M+-NO2, 23%), 208 (15), 143 (51), 112 (13), 98 (15), 97 (100), 96 (14), 69 (17), 56 (17), 55 (34); HRMS (ESI) calcd for C13H25N2O3 (M+H) 257.1865, found 257.1857.
Preparation of 6-substituted piperidine-2,5-diones 11. General procedure.
To a solution of the corresponding nitropiridinone 10 (0.2 mmol) in MeOH (0.5 mL) was added dropwise a 1M solution of NaOMe in MeOH (0.3 mL, 0.3 mmol) at room temperature. The reaction mixture was stirred at this temperature for 2 h and after that, it was cooled down to -50 ºC. To the resulting reaction mixture was added first a 1M solution of H2SO4 in MeOH (0.6 mL, 0.6 mmol) and after 10 min, H2O (1.0 mL). The reaction mixture was allowed to reach room temperature, basified with saturated aqueous solution of NaHCO3, extracted with EtOAc (3 × 10 mL), dried over anhydrous MgSO4 and evaporated (15 Torr). The resulting residue was suspended in H2O and then a 1M solution of H2SO4 in H2O (0.02 mL, 0.02 mmol) was added. The reaction mixture was stirred at 100 ºC for 3 h and after that it was cooled down to room temperature, basified with saturated aqueous solution of NaHCO3 and extracted with EtOAc (3 × 10 mL). The organic layer was washed with brine (2 × 10 mL), dried over anhydrous MgSO4 and evaporated (15 Torr). The resulting white solid was purified by column chromatography (silica gel, hexane/EtOAc) to yield pure compounds 11. Yields are given in Table 2. Physical and spectroscopic data follow.
(S)-6-(2-Phenylethyl)piperidine-2,5-dione (11a). White solid; mp 99-102 ºC (hexane/CH2Cl2); [α]20D +29 (c 1.02, CH2Cl2); Rf 0.24 (hexane/EtOAc: 1/3); IR ν (KBr) 3193, 2957, 2927, 1717, 1663, 1417, 1318, 749, 703 cm-1; 1H NMR (300 MHz, CDCl3) δ 7.33-7.25 (2H, m, ArH), 7.24-7.15 (3H, m, ArH), 6.76 (1H, s, NH), 3.90-3.74 (1H, m, CH), 2.79-2.69 (2H, m, CH2), 2.69-2.62 (4H, m, 2 × CH2), 2.25-2.12 (1H, m, CHH), 2.10-1.98 (1H, m, CHH); 13C NMR (75 MHz, CDCl3) δ 206.1, 172.2, 139.9 (C), 128.7, 128.4, 126.5, 60.1 (CH), 35.3, 34.2, 31.1, 29.0 (CH2); LRMS (EI) m/z 217 (M+, 100%), 134 (12), 132 (27), 117 (21), 113 (66), 98 (15), 91 (78), 84 (56); HRMS (ESI) calcd for C13H16NO2 (M+H) 218.1181, found 218.1172.
(S)-6-Isobutylpiperidine-2,5-dione (11b). White solid; mp 136-139 ºC (hexane/CH2Cl2); [α]20D +23 (c 0.23, CH2Cl2); Rf 0.21 (hexane/EtOAc: 1/3); IR ν (KBr) 3203, 2957, 2927, 2868, 1719, 1659, 1426, 1314 cm-1; 1H NMR (300 MHz, CDCl3) δ 6.37 (1H, s, NH), 3.95-3.76 (1H, m, CH), 2.70-2.66 (4H, m, 2 × CH2), 1.88-1.65 (2H, m, CH2), 1.65-1.45 (1H, m, CH), 0.97 (3H, d, J = 6.0 Hz, Me), 0.94 (3H, d, J = 5.9 Hz, Me); 13C NMR (75 MHz, CDCl3) δ 206.5, 172.0 (C), 59.2 (CH), 41.5, 35.0, 29.1 (CH2), 24.3 (CH), 23.1, 21.2 (Me); LRMS (EI) m/z 141 (M+-CO, 6%), 126 (6), 112 (9), 86 (26), 84 (100), 57 (9); HRMS (ESI) calcd for C9H16NO2 (M+H) 170.1181, found 170.1173.
(R)-6-Octylpiperidine-2,5-dione (11c). White solid; mp 83-85 ºC (hexane/CH2Cl2); [α]20D +47 (c 0.60, CH2Cl2); Rf 0.31 (hexane/EtOAc: 1/3); IR ν (KBr) 3222, 2917, 2849, 1717, 1669, 1420, 1328, 724 cm-1; 1H NMR (300 MHz, CDCl3) δ 6.26 (1H, s, NH), 3.89-3.74 (1H, m, CH), 2.76-2.61 (4H, m, 2 × CH2), 1.89-1.64 (2H, m, CH2), 1.30-1.23 (12H, m, 6 × CH2), 0.88 (3H, t, J = 6.9 Hz, Me); 13C NMR (75 MHz, CDCl3) δ 206.2, 172.0 (C), 60.9 (CH), 35.3, 32.9, 31.8, 29.3, 29.2, 29.1, 29.0, 25.0, 22.6 (CH2), 14.1, (Me); LRMS (EI) m/z 225 (M+, 1%), 142 (13), 84 (100), 56 (10); HRMS (ESI) calcd for C13H24NO2 (M+H) 226.1807, found 226.1798.
ACKNOWLEDGEMENTS
This work was generously supported by the Spanish Ministerio de Ciencia e Innovación (Grant Nos. CTQ2011-24165, and Consolider Ingenio 2010-CSD-2007-00006), the Generalitat Valenciana (Grant No. PROMETEO/2009/039 and FEDER) and the University of Alicante. M.J.G.M. thanks the University of Alicante for a predoctoral fellowship. We also thank MEDALCHEMY S.L. for a gift of chemicals.
References
1. M. Schneider, Alkaloids: Chemical and Biological Perspectives, Vol. 10, ed. by S. W. Pelletier, Pergamon: Oxford, 1996, pp. 55–299.
2. M. A. Wijdeven, F. L. van Delft, and F. P. J. T. Rutjes, Tetrahedron, 2010, 66, 5623. CrossRef
3. D. Enders, B. Nolte, and J. Runsink, Tetrahedron: Asymmetry, 2002, 13, 587. CrossRef
4. J. B. Koepeli, J. F. Mead, and J. Brockman, J. Am. Chem. Soc., 1947, 69, 1837. CrossRef
5. N. Desideri, A. Galli, J. Sestili, and M. L. Stein, Arch. Pharm., 1992, 325, 29. CrossRef
6. (a) G. Ratle, X. Monseur, B. C. Das, J. Yassi, Q. Khuong-Huu, and R. Goutarel, Bull. Soc. Chim. Fr., 1966, 2945; (b) Q. Khuong-Huu, G. Ratle, X. Monseur, and R. Goutarel, Bull. Soc. Chim. Belg., 1972, 81, 425; CrossRef (c) Q. Khuong-Huu, G. Ratle, X. Monseur, and R. Goutarel, Bull. Soc. Chim. Belg., 1972, 81, 443. CrossRef
7. (a) J. Löfstedt, H. Pettersson-Fasth, and J.-E. Bäckvall, Tetrahedron, 2000, 56, 2225; CrossRef (b) P. N. M. Botman, F. J. Dommerholt, R. de Gelder, Q. B. Broxterman, H. E. Schoemaker, F. P. J. T. Rutjes, and R. H. Blaauw, Org. Lett., 2004, 6, 4941; CrossRef (c) J.-C. Jung and M. A. Avery, Tetrahedron: Asymmetry, 2006, 17, 2479. CrossRef
8. C. J. Moody, A. P. Lightfoot, and P. T. Gallagher, J. Org. Chem., 1997, 62, 746. CrossRef
9. H. Sakagami, T. Kamikubo, and K. Ogasawara, J. Chem. Soc., Chem. Commun., 1996, 1433. CrossRef
10. (a) F. Foubelo and M. Yus, Tetrahedron: Asymmetry, 2004, 15, 3823; CrossRef (b) J. C. González-Gómez, F. Foubelo, and M. Yus, Synlett, 2008, 2777; (c) M. Medjahdi, J. C. González-Gómez, F. Foubelo, and M. Yus, Heterocycles, 2008, 76, 569; CrossRef (d) M. Medjahdi, J. C. González-Gómez, F. Foubelo, and M. Yus, J. Org. Chem., 2009, 74, 7859; CrossRef (e) H. K. Dema, F. Foubelo, and M. Yus, Heterocycles, 2010, 80, 125; CrossRef (f) J. C. González-Gómez, M. Medjahdi, F. Foubelo, and M. Yus, J. Org. Chem., 2010, 75, 6308; CrossRef (g) H. K. Dema, F. Foubelo, and M. Yus, Heterocycles, 2011, 82, 1411; CrossRef (h) M. Medjahdi, J. C. González-Gómez, F. Foubelo, and M. Yus, Eur. J. Org. Chem., 2011, 2230; CrossRef (i) H. K. Dema, F. Foubelo, and M. Yus, Jordan J. Chem., 2011, 6, 247; (j) J. C. González-Gómez, F. Foubelo, and M. Yus, Org. Synth., 2012, 89, 88; CrossRef (k) I. Bosque, J. C. González-Gómez, F. Foubelo, and M. Yus, J. Org. Chem., 2012, 77, 780 (correction: I. Bosque, J. C. González-Gómez, F. Foubelo, and M. Yus, J. Org. Chem., 2012, 77, 4190); CrossRef (l) I. Bosque, J. C. González-Gómez, A. Guijarro, F. Foubelo, and M. Yus, J. Org. Chem., 2012, 77, 10340; CrossRef (m) J. A. Sirvent, F. Foubelo, and M. Yus, Chem. Commun., 2012, 48, 2543; CrossRef (n) H. K. Dema, F. Foubelo, and M. Yus, Helv. Chim. Acta, 2012, 95, 1790; CrossRef (o) M. Medjahdi, J. C. González-Gómez, F. Foubelo, and M. Yus, Heterocycles, 2012, 86, 727; CrossRef (p) M. J. García-Muñoz, F. Zacconi, F. Foubelo, and M. Yus, Eur. J. Org. Chem., 2013, 1287; CrossRef (q) J. A. Sirvent, F. Foubelo, and M. Yus, Eur. J. Org. Chem., 2013, 2461; CrossRef (r) J. A. Sirvent, F. Foubelo, and M. Yus, Heterocycles, 2018, 88, 1163; CrossRef (s) J. A. Sirvent, F. Foubelo, and M. Yus, J. Org. Chem., 2014, 79, 1356; CrossRef (t) M. J. García-Muñoz, H. K. Dema, F. Foubelo, and M. Yus, Tetrahedron: Asymmetry, 2014, 25, 362; CrossRef (u) O. S. R. Barros, J. A. Sirvent, F. Foubelo, and M. Yus, Chem. Commun., 2014, 50, 6898. CrossRef
11. For reviews, see: (a) G.-Q. Lin, M.-H. Xu, Y.-W. Zhong, and X.-W. Sun, Acc. Chem. Res., 2008, 41, 831; (b) F. Ferreira, C. Botuha, F. Chemla, and A. Pérez-Luna, Chem. Soc. Rev., 2009, 38, 1162; CrossRef (c) M. A. T. Robak, M. A. Herbage, and J. A. Ellman, Chem. Rev., 2010, 110, 3600; CrossRef (d) F. Foubelo and M. Yus, Eur. J. Org. Chem., 2014, 485. CrossRef
12. T. P. Tang and J. A. Ellman, J. Org. Chem., 1999, 64, 12. CrossRef
13. (a) J. L. García Ruano, M. Topp, J. López-Cantarero, J. Alemán, M. J. Remuiñán, and M. B. Cid, Org. Lett., 2005, 7, 4407; CrossRef (b) J. L. García Ruano, J. López-Cantarero, T. De Haro, J. Alemán, and M. B. Cid, Tetrahedron, 2006, 62, 12197. CrossRef
14. J. L. García Ruano, T. de Haro, R. Singh, and M. B. Cid, J. Org. Chem., 2008, 73, 1150. CrossRef
15. L. B. Schenkel and J. A. Ellman, Org. Lett., 2004, 6, 3621. CrossRef
16. L. Nielsen, K. B. Lindsay, J. Faber, N. C. Nielsen, and T. Skrydstrup, J. Org. Chem., 2007, 72, 10035. CrossRef
17. R. Almansa, D. Guijarro, and M. Yus, Tetrahedron: Asymmetry, 2008, 19, 2484. CrossRef
18. J. Escalante and F. D. Díaz-Coutiño, Molecules, 2009, 14, 1595. CrossRef
19. B. M. Choudary, M. Lakshmi Kantam, B. Kavita, C. Venkat Reddya, and F. Figueras, Tetrahedron, 2000, 56, 9357.