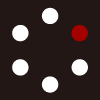
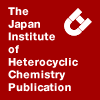
HETEROCYCLES
An International Journal for Reviews and Communications in Heterocyclic ChemistryWeb Edition ISSN: 1881-0942
Published online by The Japan Institute of Heterocyclic Chemistry
e-Journal
Full Text HTML
Received, 21st March, 2014, Accepted, 14th April, 2014, Published online, 18th April, 2014.
DOI: 10.3987/COM-14-S(K)19
■ ABC → ABCE/D Based Approaches to the Pentacyclic Ring System of the Vinca Alkaloids Using Intramolecular Hetero-[2+2]cycloaddition and Gold(I)-Catalyzed 6-endo-dig Cyclisation Protocols
Lorenzo V. White, Martin G. Banwell,* and Anthony C. Willis
Research School of Chemistry, Australian National University, Canberra, ACT 0200, Australia
Abstract
The angularly substituted tetrahydrocarbazole 13, which is readily obtained from cyclohexane-1,4-dione monoethylene ketal (6) using Fischer indole chemistry, has been converted into the isothiocyanate 16. Photolysis of this last compound affords, via an intramolecular hetero-[2+2]cycloaddition reaction, the pentacyclic β-thiolactam 17 that incorporates the ABCE ring substructure of natural products 1–3. Attempts to effect a two-carbon homologation of the four-membered ring within compound 17, and thereby establish the D-ring, failed. The azide 20, also obtained from compound 13, forms the cyclic imine 21 on thermolysis in refluxing toluene and the readily derived enamide 23 engages in a Au(I)-catalysed 6-endo-dig cyclisation reaction to give compound 24 embodying the ABCDE ring system of the title alkaloids.INTRODUCTION
Members of the Vinca class of alkaloid have been the subject of extensive synthetic studies.1 These are ongoing and have been prompted by the fascinating molecular architectures of such natural products and, in certain instances, their attendant and significant biological properties.1,2 For example, the pentacyclic vindoline (1) is the principal alkaloid found in the plant Catharanthus roseus and a biogenetic precursor to the co-occurring but much less abundant indole-indoline-containing systems vinblastine (2) and vincristine (3), compounds that are currently being used in the clinical treatment of various human cancers because of their potent antimitotic properties.1,2 It is this situation, combined with the prospect that they might be effective in other therapeutic settings,1 that has prompted extensive (and notably successful) studies directed toward the total synthesis of compounds such as 1–31 as well as various derivatives3,4 (including ones incorporating “deep-seated” modifications) that continue to display significant biological activities.
Some time ago we noted5 that the cyclohexa-3,5-diene-1,2-diol 4, which is readily obtained in enantiomerically pure form through the whole-cell biotransformation of m-ethyltoluene, bears a strong structural resemblance to the highly functionalised C-ring of the alkaloids 1–3. Accordingly, we sought to identify protocols that might allow for the elaboration of functionalised cyclohexanes such as metabolite 4 into the ABCDE framework associated with these natural products.6 As a result, we now describe the outcomes of a model study that provides usefully functionalised forms of the ABC-ring system and to which the DE substructure (or a potential precursor to it) can be appended. The sequence involves the starting C-ring-containing compound being fused with the A- and B-rings using Fischer indole chemistry and the resulting ABC-substructure being annulated using either hetero-[2+2]cycloaddition or Au(I)-mediated cyclisation reactions. The study reported here has certain parallels with ones in which we have been seeking to develop methods for the synthesis of the closely related Aspidosperma alkaloids.7,8
RESULTS AND DISCUSSION
The assembly of the pivotal ABC-ring-containing system is shown in Scheme 1 and uses phenylhydrazine (5) and cyclohexane-1,4-dione monoethylene ketal (6) as substrates for the initial Fischer indole synthesis with the latter destined to become incorporated as the C-ring of the target framework. From this point, chemical manipulations of product 79 (94%) were focused on the introduction of relevant functionality onto the C-ring including that considered appropriate for creating the five-membered E-ring. To such ends, compound 7 was treated with n-butyllithium, copper(I) iodide then allyl bromide and so providing the previously reported10 C3-allylated isoindole 8 (88%) that was subjected to reductive methylation using formaldehyde in the presence of sodium cyanoborohydride.
The N-methylindoline 9 (87%) so-formed was exposed to aqueous hydrochloric acid in THF thereby cleaving the associated ketal moiety and thus affording cyclohexane 10 (92%), the structure of which was confirmed by single-crystal X-ray analysis (see Experimental section for details). Reaction of latter compound with potassium hydride then Comins’ reagent11 (a process believed to proceed under conditions of thermodynamic control) resulted in the essentially completely regioselective formation of enol triflate 11 (89%), the allyl side-chain of which could be oxidatively cleaved through successive treatment with a potassium osmate/potassium ferricyanide/DABCO12 mixture then sodium metaperiodate. The aldehyde 12 (85%) so-formed was treated with tert-butylamine (so as to effect temporary protection of the carbonyl moiety) and then with the Gilman-type reagent generated through reaction of ethylmagnesium bromide with copper(I) cyanide. By such means, and after aqueous work-up, the pivotal and ethyl-substituted cyclohexene 13 (83%) was obtained.
At this point, two quite distinct strategies were pursued in efforts to convert, via DE-ring annulation, compound 13 into the pentacyclic ring system associated with the Vinca alkaloids. The first of these sought to exploit intramolecular and hetero-[2+2]cycloaddition chemistry for this purpose and with the intention of engaging any product azetidine in a two carbon-homologation reaction so as to form the six-membered D-ring. To such ends, and as shown in Scheme 2, the oxime derived from aldehyde 13 was reduced with zinc in acetic acid13 under conditions of ultrasonication to give the 1°-amine 14 in 90% yield and the structure of which was confirmed by single-crystal X-ray analysis of the readily derived oxalate salt. Reaction of this amine with Boc2O in the presence of DMAP14 gave the corresponding isocyanate 15 in 89% yield while treatment of the same substrate with carbon disulfide and Boc2O in the presence of triethylamine and DMAP15 gave the stable and thus fully characterisable isothiocyanate 16 in 82% yield. A variety of conditions was explored in an effort to engage compounds 15 and 16 in an intramolecular hetero-[2+2]cycloaddition reaction.16,17 Simply heating solutions of these compounds at a range of different temperatures up to 211 °C (using nitrobenzene as solvent) failed, as did treating them with variety of catalysts, including ones that it was thought might promote “open-shell” cycloaddition reactions.18 Ultimately, irradiation of a benzene solution of the isothiocyante 16 for 1 h at 18 °C resulted (Scheme 2) in the formation of the β-thiolactam 17 (14%). Analogous treatment of isocyanate 15 failed to produce the equivalent β-lactam 18. The spectral data recorded on compound 17 were in complete accord with the assigned structure. In particular, the 13C NMR spectrum displayed a resonance at δC 219.1 that is assigned to the newly installed β-thiolactam carbonyl carbon while the infra-red spectrum contained a strong and characteristic C=S stretching band at 1412 cm–1.
Despite considerable experimentation, including an investigation of flow photochemical techniques,19 the yield of the β-thiolactam 17 could never be raised above the ca. 14% level. The formation of elemental sulfur was a persistent feature of all those reactions leading to compound 17 and independent irradiation of this material resulted in its relatively rapid decomposition (with accompanying formation of sulfur). This situation coupled with an inability to identify an effective two-carbon homologation protocol that would generate the required six-membered D-ring from the thio-β-lactam lead to the abandonment of the hetero-[2+2]cycloaddition approach just described.
The second strategy examined in an effort to annulate the D- and E-rings to the ABC-framework of aldehyde 13 is shown in Scheme 3 and involved, as the first step, its reduction to the corresponding alcohol that was then converted into the mesylate 19. As a result of its instability, this last compound was immediately treated with sodium azide in DMF and so producing the anticipated (and stable) compound 20 in 88% yield (from 13). With compound 20 to hand efforts were made to engage the associated azide and alkenic residues in cyclisation processes that would lead to the construction of the required E-ring. In the event, and in anticipation of effecting an intramolecular [3+2]cycloaddition reaction between these two functionalities,7b,c azide 20 was heated at reflux in toluene for 4 h (Scheme 3). However, rather than the expected triazene being isolated, imine 21 was obtained as the major product of reaction20 and as a single diastereoisomer of undefined configuration at the ethyl-bearing centre. This was accompanied by small amounts of the isomeric but rather unstable enamine that was readily converted into imine 21 and its epimer on sustained contact with silica gel. When compound 21 was treated with acryloyl chloride in the presence of triethylamine then the crystalline amide 22 was obtained in 90% yield and its structure confirmed by single-crystal X-ray analysis. While various attempts to effect the cyclisation of this compound and thus form the D-ring of the Vinca alkaloids were unsuccessful, when the similarly derived propiolamide 23 (82% from 20) was treated with Echavarran’s gold(I) catalyst21 in the presence of p-TsOH•H2O then a 6-endo-dig cyclisation22 took place and thus providing, after aqueous work-up, the rather unstable pentacyclic compound 24. This was obtained in ca. 33% yield. The assignment of the structure of compound 24 follows from the derived spectral data. Most notably, the 400 MHz 1H NMR spectrum of this material displayed an AX spin system (δH = 6.54 and 5.99, J = 10.2 Hz) arising from the mutually coupled protons of the newly formed cyclic double bond. The signals due to the sp-hybridised carbons of precursor 23 (observed at δC 78.4/77.2 and 69.5/69.0 – pairing due to the presence of amide rotamers) were no longer evident in the 13C NMR spectrum of product 24 which displayed a total of twenty resonances including one at δC 93.3 that is attributed to the hydroxy-substituted and sp3-hybridised carbon formed as a result of water adding to the N-acyliminium ion generated during the cyclisation event. The infra-red spectrum of compound 24 displayed prominent absorption bands at 3352, 1657 and 1601 cm–1 that are attributed to the associated hydroxyl, lactam carbonyl and C=C moieties, respectively.
Extensive efforts, including those involving the use of other gold catalysts, the use of stoichiometric amounts of the original one and/or derivatives of the substrate in which the triple bond was capped by a methyl or a trimethylsilyl group, failed to improve the yield of this type of cyclisation reaction. The use of both a gold(I) species and an acid was clearly pivotal for the success of the conversion 23 → 24 since in the absence of one or other of these reagents no cyclisation product was observed.
Despite the modest yields associated with the last step of the reaction sequence shown in Scheme 3, the chemistry reported therein is now being applied in an effort to effect the conversion 4 → 1 while the scope and limitations of the Au(I)-catalysed cyclisation of N-(cyclohex-1-en-1-yl)propiolamides (as exemplified by the conversion of compound 23 into isomer 24) and related compounds is also being investigated. Results will be reported in due course.
In closing, it is worth noting that since Trost and Quancard23 have demonstrated the conversion 7 → 8 can be carried out enantioselectively (66% ee)24 the protocols reported here could be used to make compounds 17 and 24, as well as various of their precursors, in chiral, non-racemic form.25
EXPERIMENTAL
General Experimental Procedures. Unless otherwise specified, proton (1H) and carbon (13C) NMR spectra were recorded at room temperature in base-filtered CDCl3 on a Varian spectrometer operating at 400 MHz for proton and 100 MHz for carbon nuclei or on a Bruker Avance 800 machine operating at 800 MHz for proton and 200 MHz for carbon nuclei. 1H NMR data are reported as follows: chemical shift (δ) [multiplicity, coupling constant(s) J (Hz), relative integral] where multiplicity is defined as: s = singlet; d = doublet; t = triplet; q = quartet; m = multiplet or combinations of the above. The signal due to residual CHCl3 appearing at δH 7.26 and the central resonance of the CDCl3 “triplet” appearing at δC 77.0 were used to reference 1H and 13C NMR spectra, respectively. Infrared spectra (νmax) were recorded on a Perkin–Elmer 1800 Series FTIR Spectrometer. Samples were analysed as thin films on KBr plates. Low-resolution ESI mass spectra were recorded on a single quadrupole liquid chromatograph-mass spectrometer, while high-resolution measurements were conducted on a time-of-flight instrument. Low- and high-resolution EI mass spectra were recorded on a magnetic-sector machine. Melting points were measured on an Optimelt™ automated melting point system and are uncorrected. Analytical thin layer chromatography (TLC) was performed on aluminum-backed 0.2 mm thick silica gel 60 F254 plates as supplied by Merck. Eluted plates were visualised using a 254 nm UV lamp and/or by treatment with a suitable dip followed by heating. These dips included phosphomolybdic acid : ceric sulfate : sulfuric acid (conc.) : water (37.5 g : 7.5 g : 37.5 g : 720 mL) or potassium permanganate : potassium carbonate : 5% sodium hydroxide aqueous solution : water (3 g : 20 g: 5 mL : 300 mL). Flash chromatographic separations were carried out following protocols defined by Still et al.26 with silica gel 60 (40–63 µm) as the stationary phase and using the AR- or HPLC-grade solvents indicated. Starting materials and reagents were generally available from the Sigma–Aldrich, Merck, TCI, Strem or Lancaster Chemical Companies and were used as supplied. Drying agents and other inorganic salts were purchased from the AJAX, BDH or Unilab Chemical Companies. Tetrahydrofuran (THF), MeOH and CH2Cl2 were dried using a Glass Contour solvent purification system that is based upon a technology originally described by Grubbs et al.27 Where necessary, reactions were performed under a nitrogen atmosphere.
Specific Experimental Procedures and Product Characterisation
Compound 9. A magnetically stirred solution of compound 810 (2.50 g, 9.2 mmol) in MeCN/AcOH (250 mL of a 10:1 v/v mixture) maintained at 18 °C was treated with H2CO (125 mL of a 37% aqueous solution). NaBH3CN (2.92 g, 46.5 mmol) was then added in equal portions over 0.16 h and the ensuing mixture stirred at 18 °C for 0.5 h before being quenched with Na2CO3 (100 mL of a saturated aqueous solution). The separated aqueous phase was extracted with EtOAc (3 × 100 mL) and the combined organic phases dried (Na2SO4) then filtered and concentrated under reduced pressure at 40 °C. The light-yellow oil thus obtained was subjected to flash chromatography (silica, 1:5 v/v EtOAc/hexane elution) and concentration of the appropriate fractions (Rf = 0.65 in 1:3 v/v EtOAc/hexane) afforded compound 9 (2.30 g, 87%) as a golden-coloured oil (Found: M+•, 285.1729. C18H23NO2 requires M+•, 285.1729). 1H NMR (400 MHz, CDCl3) δ 7.10 (dt, J = 7.8 and 1.2 Hz, 1H), 7.03 (dd, J = 7.4 and 1.2 Hz, 1H), 6.72 (dt, J = 7.4 and 1.2 Hz, 1H), 6.56 (d, J = 7.8 Hz, 1H), 5.76 (m, 1H), 5.08 (d, J = 16.8 Hz, 1H), 5.00 (d, J = 10.2 Hz, 1H), 4.03–3.95 (complex m, 2H), 3.93–3.87 (complex m, 2H), 3.02 (app t, J = 3.3 Hz, 1H), 2.82 (dd, J = 14.6 and 9.1 Hz, 1H), 2.70 (s, 3H), 2.62 (dd, J = 14.6 and 5.6 Hz, 1H), 2.04–1.81 (complex m, 4H), 1.71 (dd, J = 14.6 and 2.3 Hz, 1H), 1.58–1.53 (complex m, 1H); 13C NMR (100 MHz, CDCl3) δ 151.7, 136.8, 135.9, 127.3, 121.7, 118.2, 117.0, 108.3, 108.2, 67.9, 64.4, 63.8, 47.0, 42.4, 39.3, 33.4, 29.1, 20.8; νmax 2953, 2878, 1605, 1481, 1373, 1302, 1274, 1158, 1130, 1113, 1073, 979, 918, 741 cm–1; Mass spectrum (EI, 70 eV) m/z 285 (M+•, 38%), 244 (100), 200 (40), 182 (30), 157 (19), 144 (41), 99 (68).
Compound 10. A magnetically stirred solution of compound 9 (10.10 g, 35.4 mmol) in THF (375 mL) containing HCl (375 mL of 10% aqueous solution) was stirred at 18 °C for 4 h then quenched with Na2CO3 (250 mL of a saturated aqueous solution) and the separated aqueous phase was extracted with CH2Cl2 (3 × 150 mL). The combined organic phases were dried (Na2SO4), filtered and concentrated under reduced pressure. The brown solid thus obtained was subjected to flash chromatography (silica, 1:3 v/v EtOAc/hexane elution). Concentration of the appropriate fractions (Rf = 0.4) afforded a white solid, recrystallisation (EtOAc) of which afforded compound 10 (7.86 g, 92%) as a white, crystalline solid, mp 82–83 °C (Found: M+•, 241.1469. C16H19NO requires M+•, 241.1467). 1H NMR (400 MHz, CDCl3) δ 7.08 (dt, J = 7.7 and 1.3 Hz, 1H), 6.93 (dd, J = 7.4 and 1.3 Hz, 1H), 6.65 (dt, J = 7.4 and 1.0 Hz, 1H), 6.42 (dd, J = 7.7 and 1.0 Hz, 1H), 5.66 (m, 1H), 5.12 (dd, J = 2.6 and 0.6 Hz, 1H), 5.08 (dd, J = 6.1 and 0.6 Hz, 1H), 3.48 (app. t, J = 3.8 Hz, 1H), 2.78 (s, 3H), 2.55 (d, J = 6.0 Hz, 2H), 2.53–2.48 (complex m, 1H), 2.41–2.32 (complex m, 2H), 2.23–2.14 (complex m, 2H), 2.06–1.98 (complex m, 1H); 13C NMR (100 MHz, CDCl3) δ 211.2, 151.5, 133.8, 133.4, 128.4, 122.4, 118.9, 117.7, 106.4, 67.2, 48.9, 48.6, 45.0, 34.5, 32.6, 23.5; νmax 2964, 2932, 2861, 1709, 1603, 1447, 1464, 1421, 1302, 1227, 1214, 1155, 1001, 935, 749, 460 cm–1. Mass spectrum (EI, 70 eV) m/z 241 (M+•, 27%), 200 (100), 144 (69).
Compound 11. KH (1.70 g of a 30% dispersion in mineral oil, 12.7 mmol) contained in a two-necked round-bottomed flask and maintained under a nitrogen atmosphere was washed with hexane (2 × 5 mL) before being dried under vacuum then suspended in anhydrous THF (100 mL). While being stirred magnetically at ca. 18 °C the suspension was treated, dropwise, with a solution of ketone 10 (2.05 g, 8.5 mmol) in THF (30 mL) (CAUTION: evolution of hydrogen gas). After 1 h a solution of Comins’ reagent (5.00 g, 12.7 mmol) in THF (30 mL) was added dropwise over 0.17 h. The reaction mixture thus obtained was stirred for a further 1 h and then quenched with NH4Cl (150 mL of a 1:1 v/v mixture of a saturated aqueous solution and water). The separated aqueous layer was washed with EtOAc (3 × 100 mL) and the combined organic washings then dried (Na2SO4) filtered and concentrated under reduced pressure. The brown oil thus obtained was subjected to flash chromatography (silica, 1:19 v/v EtOAc/hexane elution) and concentration of the appropriate fractions (Rf = 0.8 in 1:5 v/v EtOAc/hexane) afforded compound 11 (2.82 g, 89%) as a clear, colourless oil (Found: M+•, 373.0963. C17H18F3NO3S requires M+•, 373.0960). 1H NMR (400 MHz, CDCl3) δ 7.13 (dt, J = 7.8 and 1.2 Hz, 1H), 7.04 (dd, J = 7.3 and 0.8 Hz, 1H), 6.73 (dt, J = 7.3 and 0.8 Hz, 1H), 6.51 (broadened d, J = 7.8 Hz, 1H), 5.76 (m, 1H), 5.55 (d, J = 2.1 Hz, 1H), 5.14 (s, 1H), 5.11 (dd, J = 6.5 and 1.6 Hz, 1H), 3.34 (app. t, J = 3.8 Hz, 1H), 2.71 (s, 3H), 2.67–2.53 (complex m, 3H), 2.22–2.11 (complex m, 2H), 2.03–1.94 (complex m, 1H); 13C NMR (100 MHz, CDCl3) δ 151.6, 148.8, 133.5, 132.5, 128.6, 122.5, 122.3, 118.5, 118.5 (partially obscured q, J = 320 Hz), 118.4, 108.1, 67.3, 48.3, 42.7, 32.9, 23.1, 21.6; νmax (KBr) 2956, 1605, 1486, 1416, 1245, 1211, 1142, 1024, 913, 867, 745, 606 cm–1; Mass spectrum (EI, 70 eV) m/z 373 (M+•, 38%), 332 (100), 250 (22), 199 (84), 171 (96), 144 (27), 143 (41).
Compound 12. A vigorously stirred suspension of triflate 11 (3.00 g, 8.0 mmol) in tert-butanol/water (100 mL of a 1:1 v/v mixture) maintained at 18 °C was treated with K2CO3 (3.33 g, 24.1 mmol), K3Fe(CN)6 (7.92 g, 24.1 mmol), DABCO (450 mg, 4.01 mmol) and K2OsO4•2H2O (147 mg, 0.4 mmol). The resulting slurry was stirred for a further 1 h then treated with Na2SO3 (100 mL of a saturated aqueous solution) and after 0.25 h diluted with EtOAc (50 mL). The separated aqueous phase was extracted with EtOAc (3 × 75 mL) and the combined organic phases then dried (Na2SO4), filtered and concentrated under reduced pressure. The yellow oil thus obtained was dissolved acetone/water (100 mL of a 1:1 v/v mixture) and the resulting solution stirred at 18 °C then treated with NaIO4 (3.43 g, 16.0 mmol) and stirred for 0.5 h before being filtered (to remove the ensuing white precipitate). The filtrate was extracted with EtOAc (3 × 50 mL) and the combined organic phases then dried (Na2SO4), filtered and concentrated under reduced pressure. The residue thus obtained was subjected to flash chromatography (silica, 1:3 v/v EtOAc/hexane elution) and concentration of the relevant fractions (Rf = 0.6 in 1:3 v/v EtOAc/hexane) afforded triflate 12 (2.56 g, 85%) as a clear, colourless oil (Found: M+•, 375.0751. C16H16F3NO4S requires M+•, 375.0752). 1H NMR (400 MHz, CDCl3) δ 9.74 (app. t, J = 2.6 Hz, 1H), 7.16 (dt, J = 7.8 and 1.2 Hz, 1H), 7.07 (d, J = 7.4 Hz, 1H), 6.75 (dt, J = 7.4 and 1.2 Hz, 1H), 6.53 (d, J = 7.8 Hz, 1H), 5.73 (s, 1H), 3.42 (m, 1H), 2.85 (ABq, J = 15.8 Hz, 2H), 2.73 (s, 3H), 2.61 (m, 1H), 2.26 (m, 1H), 2.15 (m, 1H), 1.98 (m, 1H); 13C NMR (100 MHz, CDCl3) δ 200.2, 151.2, 149.3, 130.8, 129.2, 122.6, 120.7, 118.8, 118.4 (partially obscured q, J = 319 Hz) 108.4, 67.9, 51.0, 46.8, 32.7, 23.3, 21.3; νmax (KBr) 2867, 1722, 1605, 1486, 1416, 1246, 1211, 1141, 1028, 895, 752, 609 cm–1; Mass spectrum (EI, 70 eV) m/z 375 (M+•, 81%), 332 (85), 199 (92), 171 (100), 170 (48), 143 (46).
Compound 13. A magnetically stirred solution of triflate 12 (2.28 g, 6.07 mmol) in THF (50 mL) maintained at 18 °C under a nitrogen atmosphere was treated with dried 4 Å molecular sieves (2.30 g) then tert-butylamine (670 µL, 466 mg, 6.38 mmol) and the resulting mixture stirred for 1 h. In a separate flask a magnetically stirred suspension of CuCN (2.72 g, 30.4 mmol) in THF (50 mL) maintained under a nitrogen atmosphere was cooled to –78° C then treated with EtMgBr (10.1 mL of a 3 M solution in Et2O, 30.3 mmol). The ensuing mixture was warmed to 0 °C and stirred at this temperature for 0.17 h (turns black). The original mixture containing triflate 12 and tert-butylamine was filtered, under a nitrogen atmosphere, to remove the molecular sieves that were washed with THF (3 × 10 mL). The combined filtrates were added to the abovementioned black reaction mixture derived from CuCN and EtMgBr and the resulting mixture was stirred for 2 h at 18 °C and then treated with NH4Cl (100 mL of a saturated aqueous solution). The separated aqueous layer was extracted with EtOAc (3 × 75 mL) and the combined organic phases dried (Na2SO4), filtered then concentrated under reduced pressure. The brown oil thus obtained was subjected to flash chromatography (silica, 1:5 v/v EtOAc/hexane elution) and concentration of the relevant fractions (Rf = 0.5) afforded alkene 13 (1.29 g, 83%) as a clear, colourless oil that solidified below 0 °C (Found: M+•, 255.1625. C17H21NO requires M+•, 255.1623). 1H NMR (400 MHz, CDCl3) δ 9.71 (app. t, J = 3.5 Hz, 1H), 7.10 (dt, J = 7.9 and 1.3 Hz, 1H), 7.05 (dd, J = 7.4 and 1.3 Hz, 1H), 6.70 (t, J = 7.4 Hz, 1H), 6.47 (d, J = 7.9 Hz, 1H), 5.34 (s, 1H), 3.42 (m, 1H), 2.73 (s, 3H), 2.70 (broadened s, 2H), 2.18–2.07 (complex m, 1H), 2.02–1.72 (complex m, 5H), 0.97 (t, J = 7.5 Hz, 3H); 13C NMR (100 MHz, CDCl3) δ 202.7, 151.2, 140.5, 134.2, 128.1, 122.3, 118.0, 107.5, 77.2, 69.1, 52.5, 46.3, 32.5, 30.4, 23.7, 21.3, 12.0; νmax 2961, 2931, 1719, 1604, 1485, 1298, 1275, 1022, 739 cm–1; Mass spectrum (EI, 70 eV) m/z 255 (M+•, 15%), 212 (100).
Compound 14. A magnetically stirred solution of aldehyde 13 (110 mg, 0.43 mmol) in MeOH/water (21 mL of a 6:1 v/v mixture) maintained at 18 °C under a nitrogen atmosphere was treated with sodium acetate (71 mg, 0.87 mmol) and NH2OH•HCl (45 mg, 0.65 mmol). The ensuing mixture was stirred at 18 °C for 1.5 h then concentrated under reduced pressure. The resulting white paste was dissolved in acetic acid (25 mL) and the solution thus formed treated with activated Zn dust (564 mg, 8.63 mg.atom) and the resulting suspension subjected to sonication for 2 h after which time it was concentrated under pressure until approximately 5 mL of material remained. This residue was treated with Na2CO3 (50 mL of a saturated aqueous solution) then extracted with CH2Cl2 (3 × 50 mL). The combined organic phases were dried (Na2SO4) and filtered before being concentrated under reduced pressure. The light-yellow oil thus obtained was subjected to flash chromatography (silica, 1:10 v/v ammonia saturated MeOH/CH2Cl2 elution) and concentration of the appropriate fractions (Rf = 0.3) gave compound 14 (99 mg, 90%) as clear, colourless oil (Found: M+•, 256.1931. C17H24N2 requires M+•, 256.1939). 1H NMR (400 MHz, CDCl3) δ 7.05 (t, J = 7.9 Hz, 1H), 6.99 (d, J = 7.6 Hz, 1H), 6.65 (t, J = 7.6 Hz, 1H), 6.43 (d, J = 7.9 Hz, 1H), 5.15 (s, 1H), 3.35 (broadened s, 1H), 2.78–2.67 (complex m, 2H), 2.72 (s, 3H), 2.17–2.06 (complex m, 1H), 2.00–1.85 (complex m, 5H), 1.80–1.68 (complex m, 2H), 1.59 (broad s, 2H), 0.94 (t, J = 7.5 Hz, 3H); 13C NMR (100 MHz, CDCl3) δ 151.6, 139.1, 136.2, 127.4, 124.3, 122.3, 117.6, 107.2, 68.8, 46.9, 43.4, 38.5, 32.8, 30.5, 23.3, 22.2, 12.1; νmax 3356, 2960, 2927, 1603, 1486, 1462, 1297, 1273, 1121, 1022, 737 cm–1; Mass spectrum (EI, 70 eV) m/z 256 (M+•, 49%), 213 (37), 212 (100), 196 (22), 183 (22), 182 (24).
A solution of a sample of amine 14 in MeOH was treated with one molar equivalent of oxalic acid in MeOH and the resulting solution allowed to stand in an uncapped vial at 18 °C until such time as crystals appeared (mp 140–141 °C). One of these was submitted for single-crystal X-ray analysis, details of which are provided below.
Compound 15. A magnetically stirred solution of Boc2O (26 mg, 0.12 mmol) in MeCN (8 mL) maintained at 18 °C under a nitrogen atmosphere was treated with DMAP (11 mg, 0.09 mmol). Stirring was continued for 0.08 h then a solution of amine 14 (20 mg, 0.08 mmol) in MeCN (2 mL) was added in one portion. The resulting mixture was stirred at 18 °C for 0.16 h then treated with NH4Cl (25 mL of a saturated aqueous solution). The separated aqueous phase was extracted with CH2Cl2 (3 × 25 mL) and the combined organic phases then dried (Na2SO4), filtered and concentrated under reduced pressure. The light-yellow oil thus obtained was subjected to flash chromatography (silica, 1:10 v/v EtOAc/hexane elution) and concentration of the appropriate fractions (Rf = 0.6) afforded isocyanate 15 (17 mg, 89%) as a clear, colourless oil (Found: M+•, 282.1729. C18H22N2O requires M+•, 282.1732). 1H NMR (400 MHz, CDCl3) δ 7.07 (t, J = 8.0 Hz, 1H), 6.96 (d, J = 7.7 Hz, 1H), 6.67 (t, J = 7.7 Hz, 1H), 6.44 (d, J = 8.0 Hz, 1H), 5.13 (s, 1H), 3.38–3.24 (complex m, 3H), 2.71 (s, 3H), 2.16–2.01 (complex m, 3H), 2.00–1.87 (complex m, 3H), 1.83–1.66 (complex m, 2H), 0.95 (t, J = 7.3 Hz, 3H); 13C NMR (100 MHz, CDCl3) δ 151.4, 140.3, 134.9, 127.8, 123.1, 122.2, 121.9, 117.8, 107.3, 68.5, 46.6, 40.8, 39.5, 32.7, 30.5, 23.4, 22.2, 12.0; νmax (KBr) 2962, 2930, 2264, 1804, 1604, 1486, 1459, 1372, 1297, 1273, 1071, 1037, 740 cm–1; Mass spectrum (EI, 70 eV) m/z 282 (M+•, 31%), 213 (30), 212 (100), 183 (22).
Compound 16. A magnetically stirred solution of amine 14 (59 mg, 0.23 mmol) in EtOH (4 mL) maintained at 18 °C under a nitrogen atmosphere was treated with triethylamine (35 µL, 25 mg, 0.25 mmol) and CS2 (140 µL, 175 mg, 2.30 mmol). The resulting mixture was stirred at 18 °C for 0.5 h then cooled to 0° C and treated, dropwise, with a solution of Boc2O (55 mg, 0.25 mmol) in EtOH (1 mL). A solution of DMAP (5 mg, 0.05 mmol) in EtOH (1 mL) was added immediately thereafter. The ensuing mixture was maintained at 0° C for 0.08 h then warmed to 18 °C and stirred at this temperature for 0.5 h. At this point the reaction mixture was concentrated under reduced pressure and the white paste thus obtained subjected to flash chromatography (silica, 5:95 v/v EtOAc/hexane elution). Concentration of the appropriate fractions (Rf = 0.6 in 1:10 v/v EtOAc/hexane) afforded isothiocyanate 16 (56 mg, 82%) as a clear, colourless oil that solidified on standing at 5 °C (Found: M+•, 298.1503. C18H22N2S requires M+•, 298.1504). 1H NMR (400 MHz, CDCl3) δ 7.08 (dt, J = 7.7 and 1.3 Hz, 1H), 6.95 (dd, J = 7.3 and 1.3 Hz, 1H), 6.68 (dt, J = 7.3 and 1.0 Hz, 1H), 6.44 (broadened d, J = 7.7 Hz, 1H), 5.14 (s, 1H), 3.52 (m, 2H), 3.35 (dd, J = 5.3 and 3.3 Hz, 1H), 2.73 (s, 3H), 2.16–2.07 (complex m, 3H), 2.01–1.90 (complex m, 3H), 1.81 (dt, J = 16.7 and 5.0 Hz, 1H), 1.75–1.68 (complex m, 1H), 3.08 (t, J = 7.5, 3H); 13C NMR (100 MHz, CDCl3) δ 151.3, 140.8, 134.3, 129.8, 128.0, 122.7, 122.1, 117.8, 107.4, 68.3, 46.6, 41.6, 39.1, 32.6, 30.5, 23.5, 22.2, 12.0; νmax 2928, 2185, 2102, 1958, 1603, 1485, 1297, 1021, 741 cm–1; Mass spectrum (EI, 70 eV) m/z 298 (M+•, 47%), 213 (32), 212 (100).
Compound 17. A magnetically stirred solution of isothiocyanate 16 (50 mg, 0.17 mmol) in benzene (10 mL) contained in a Pyrex™ vessel at 18 °C under a nitrogen atmosphere was irradiated with a high pressure mercury vapour lamp for 1 h then concentrated under reduced pressure and the resulting yellow paste subjected to flash chromatography (silica, 1:10 v/v EtOAc/hexane elution). Concentration of the appropriate fractions (Rf = 0.4) afforded β-thiolactam 17 (7 mg, 14%) as a clear, pale-yellow oil (Found: M+•, 298.1505. C18H22N2S requires M+•, 298.1504). 1H NMR (800 MHz, CDCl3) δ 7.16 (dt, J = 7.6 and 1.2 Hz, 1H), 7.11 (dd, J = 7.4 and 1.2 Hz, 1H), 6.78 (dt, J = 7.4 and 1.0 Hz, 1H), 6.54 (broadened d, J = 7.6 Hz, 1H), 3.94 (m, 1H), 3.66 (s, 1H), 3.43 (ddd, J = 14.6, 9.5 and 4.9 Hz, 1H), 3.10 (app. t, J = 3.0 Hz, 1H), 2.85 (ddd, J = 16.5, 9.5 and 7.1 Hz, 1H), 2.69 (s, 3H), 2.29 (m, 1H), 1.93 (m, 1H), 1.79 (dt, J = 13.8 and 3.3 Hz, 1H), 1.70–1.59 (complex m, 3H), 1.55–1.52 (complex m, 1H), 0.88 (t, J = 7.4 Hz, 3H); 13C NMR (100 MHz, CDCl3) δ 219.1, 153.0, 133.4, 128.5, 122.0, 118.6, 107.9, 72.6, 71.3, 57.0, 49.5, 45.9, 44.4, 33.2, 28.3, 25.2, 20.1, 8.4; νmax (KBr) 2925, 1605, 1487, 1412, 1297, 1273, 1232, 1023, 908, 752 cm–1; Mass spectrum (EI, 70 eV) m/z 298 (M+•, 31%), 213 (25), 212 (100).
Compound 20. A magnetically stirred solution of aldehyde 13 (590 mg, 2.31 mmol) in MeOH (50 mL) maintained at 18 °C under a nitrogen atmosphere was treated, in one portion, with NaBH4 (96 mg, 2.54 mmol). After 0.25 h the reaction mixture was diluted with water (50 mL) and extracted with EtOAc (3 × 50 mL). The combined organic phases were dried (Na2SO4), filtered and concentrated under reduced pressure to give a clear, colourless oil that was dissolved in CH2Cl2 (50 mL). The solution thus obtained was cooled to 0 °C and, after the establishment of a nitrogen atmosphere, was treated with triethylamine (390 µL, 283 mg, 2.7 mmol) and methanesulfonyl chloride (200 µL, 296 mg, 2.58 mmol). The ensuing mixture was stirred at 0 °C for 0.25 h then quenched with water (25 mL) containing NaHCO3 (5 drops of a saturated aqueous solution). The separated aqueous phase was extracted with CH2Cl2 (3 × 50 mL) and the combined organic phases dried (Na2SO4), filtered and concentrated under reduced pressure. The yellow oil thus obtained was dissolved in DMF (25 mL) and while being maintained with magnetic stirring at 18 °C under a nitrogen atmosphere was treated with sodium azide (450 mg, 6.92 mmol). After 24 h the reaction mixture was diluted with water (25 mL) and extracted with EtOAc (3 × 50 mL). The combined organic phases were then dried (Na2SO4) and filtered before being concentrated under reduced pressure. The light-yellow oil thus obtained was subjected to flash chromatography (silica, 1:20 v/v EtOAc/hexane elution) and concentration of the relevant fractions (Rf = 0.5 in 1:10 v/v EtOAc/hexane) afforded azide 20 (580 mg, 88%) as a clear, colourless oil (Found: M+•, 282.1845. C17H22N4 requires M+•, 282.1844). 1H NMR (400 MHz, CDCl3) δ 7.09 (dt, J = 7.7 and 1.1 Hz, 1H), 6.99 (dd, J = 7.4 and 1.2 Hz, 1H), 6.69 (t, J = 7.4 Hz, 1H), 6.46 (broadened d, J = 7.7 Hz, 1H), 5.15 (s, 1H), 3.38 (m, 1H), 3.30 (m, 2H), 2.73 (s, 3H), 2.18–2.10 (complex m, 1H), 2.04–1.92 (complex m, 5H), 1.94–1.70 (complex m, 2H), 0.97 (t, J = 7.3 Hz, 3H); 13C NMR (100 MHz, CDCl3) δ 151.5, 140.1, 135.0, 127.8, 123.3, 122.2, 117.7, 107.3, 68.5, 47.9, 46.6, 37.8, 32.7, 30.5, 23.4, 22.1, 12.0; νmax 2925, 2094, 1604, 1486, 1459, 1374, 1297, 1273, 1120, 1021, 738 cm–1; Mass spectrum (EI, 70 eV) m/z 282 (M+•, 43%), 213 (28), 212 (100).
Compound 21. A magnetically stirred solution of azide 20 (570 mg, 2.02 mmol) in toluene (60 mL) maintained under a nitrogen atmosphere was heated at reflux for 5 h then cooled to 18 °C and concentrated under reduced pressure. The yellow oil thus obtained was dissolved in EtOAc (20 mL) and the solution so formed passed through a short plug of TLC-grade silica gel that was washed with EtOAc (250 mL). The combined filtrates were concentrated under reduced pressure to give imine 21 and associated isomers (513 mg, quantitative) as a light-yellow oil (Found: M+•, 254.1783. C17H22N2 requires M+•, 254.1783). 1H NMR (400 MHz, CDCl3) δ (major isomer) 7.13 (t, J = 7.8 Hz, 1H), 6.75 (d, J = 6.9 Hz, 1H), 6.64 (t, J = 6.9 Hz, 1H), 6.47 (d, J = 7.8 Hz, 1H), 3.95 (dd, J = 15.1 and 8.6 Hz, 1H), 3.79–3.70 (complex m, 1H), 3.45 (m, 1H), 2.81 (s, 3H), 2.11–1.90 (complex m, 7H), 1.44–1.31 (complex m, 2H), 0.97 (t, J = 7.2 Hz, 3H); 13C NMR (100 MHz, CDCl3) δ (major isomer) 180.3, 149.9, 132.7, 128.4, 122.0, 117.5, 107.3, 74.9, 62.1, 57.5, 41.0, 40.8, 31.8, 27.1, 25.0, 23.8, 11.7; νmax 2931, 2861, 1638, 1603, 1480, 1446, 1372, 1215, 1123, 1019, 741 cm–1; Mass spectrum (EI, 70 eV) m/z 254 (M+•, 61%), 159 (25), 158 (100), 144 (28).
Compound 22. A magnetically stirred solution of imine 21 and its associated isomers (176 mg, 0.69 mmol) and triethylamine (100 µL, 72.6 mg, 0.69 mmol) in CH2Cl2 (25 mL) was cooled to 0 °C while being maintained atmosphere of nitrogen. Acryloyl chloride (62 µL, 68 mg, 0.75 mmol) was then added dropwise and the ensuing mixture stirred at 0 °C for 0.5 h before being treated with NaHCO3 (25 mL of a saturated aqueous solution). The separated aqueous phase was extracted with CH2Cl2 (3 × 20 mL) and the combined organic phases were then dried (Na2SO4), filtered and concentrated under reduced pressure. The yellow residue thus obtained was subjected to flash chromatography (silica, 1:1 v/v EtOAc/hexane elution) and concentration of the relevant fractions (Rf = 0.4) gave a white solid, recrystallisation (1:5 v/v EtOAc/hexane) of which afforded compound 22 (194 mg, 91%) as a white, crystalline solid, mp 144–148 °C (Found: (M+•, 308.1893. C20H24N2O requires M+•, 308.1889). 1H NMR (400 MHz, CDCl3) δ 7.08 (t, J = 7.5 Hz, 1H), 6.60–6.30 (complex m, 4H), 6.21 (dd, J = 17.2 and 9.7 Hz, 1H), 5.54 (d, J = 10.4 Hz, 1H), 4.07 (m, 1H), 3.55 (m, 2H), 2.79 (s, 3H), 2.33–2.20 (complex m, 2H), 2.09–1.80 (complex m, 5H), 1.70 (m, 1H), 0.94 (t, J = 7.6 Hz, 3H); 13C NMR (100 MHz, CDCl3) δ 165.5, 150.9, 134.2, 132.7, 129.6, 128.2, 128.0, 127.0, 122.3, 118.0, 107.2, 69.0, 50.3, 43.8, 35.5, 32.9, 25.4, 23.5, 22.5, 10.9; νmax (KBr) 2934, 1650, 1614, 1485, 1412, 1365, 1275, 1024, 956, 792, 742 cm–1; Mass spectrum (EI, 70 eV) m/z 308 (M+•, 100 %), 293 (23), 280 (48), 279 (45), 265 (21), 253 (40), 239 (30), 224 (25), 210 (41), 202 (32), 158 (52), 144 (48).
Compound 23. A magnetically stirred solution of 3-(trimethylsilyl)propynoic acid (112 mg, 0.79 mmol) and DMF (5 µL, 4.7 mg, 0.06 mmol) in CH2Cl2 (10 mL) maintained at 18 °C under a nitrogen atmosphere was treated with oxalyl chloride (68.5 µL, 101.3 mg, 0.80 mmol) After 0.5 h half (5 mL) of the solution thus formed was added to a magnetically stirred solution of imine 21 and its associated isomers (100 mg, 0.33 mmol) and Hünig’s base (210 µL) in CH2Cl2 (20 mL) maintained at 0 °C under a nitrogen atmosphere. After a further 1 h the remaining half (5 mL) of the original solution was added to the second solution and stirring of the resulting mixture continued at 0 °C for a further 1 h. The reaction mixture was then quenched by the slow addition of NaHCO3 (40 mL of a saturated aqueous solution). The separated aqueous phase was extracted with CH2Cl2 (3 × 25 mL) and the combined organic phases were dried (Na2SO4), filtered and then concentrated under reduced pressure. The thick brown oil thus obtained was dissolved in THF/water (20 mL of a 4:1 v/v mixture) and the resulting and magnetically stirred solution was treated with Na2CO3 (20 drops of a saturated aqueous solution). After 1 h the reaction mixture was diluted with EtOAc (20 mL) and the separated aqueous phase extracted with EtOAc (3 × 25 mL). The combined organic phases were dried (Na2SO4), filtered and then concentrated under reduced pressure to give a brown oil that was subjected to flash chromatography (silica, 10:30:1 v/v/v EtOAc/hexane/CH2Cl2 elution). Concentration of the appropriate fractions (Rf = 0.35 in 1:3 v/v EtOAc/hexane) afforded propiolamide 23 (98 mg, 82% from 20) as a clear, light-yellow oil (Found: M+•, 306.1731. C20H22N2O requires M+•, 306.1732). 1H NMR (400 MHz, CDCl3) (mixture of rotamers) δ 6.93 (m, 1H), 6.60 (m, 1H), 6.45 (m, 1H), 6.29 (m, 1H), 3.99–3.85 (complex m, 1H), 3.68 (m, 0.3H), 3.38–3.26 (complex m, 1.7H), 3.03 (s, 0.3H), 2.68 (s, 0.7H), 2.63 (s, 3H), 2.17–1.40 (complex m, 8H), 0.88–0.82 (complex m, 3H); 13C NMR (100 MHz, CDCl3) δ (mixture of rotamers) 152.7, 150.6, 150.5, 150.2, 134.4, 134.1, 131.8, 129.8, 129.3, 129.1, 128.2, 128.1, 122.2, 121.9, 118.0, 117.8, 107.2, 78.4, 77.2, 69.5, 69.0, 50.7, 50.4, 46.7, 44.1, 35.9, 35.6, 32.6, 32.3, 26.9, 26.1, 25.0, 23.6, 22.1, 21.7, 11.1, 10.8; νmax 3214, 2930, 2103, 1626, 1481, 1374, 1289, 1156, 1115, 741 cm–1; Mass spectrum (EI, 70 eV) m/z 306 (M+•, 72%), 278 (60), 277 (60), 250 (50), 158 (100), 144 (63), 139 (60), 124 (88).
Compound 24. A magnetically stirred solution of propiolamide 23 (20 mg, 0.07 mmol) in CH2Cl2 (10 mL) maintained under a nitrogen atmosphere at 18 °C was treated with p-TsOH•H2O (12 mg, 0.06 mmol) and the resulting solution stirred for 0.16 h then (MeCN)[(2-biphenyl)di-tert-butylphosphine]gold(I) hexafluoroantimonate (6 mg, 0.008 mmol, 11 mole %) added to it. After 18 h the reaction mixture was treated with NaHCO3 (25 mL of a saturated aqueous solution) and the separated aqueous phase extracted with CH2Cl2 (3 × 25 mL). The combined organic phases were then dried (Na2SO4), filtered and concentrated under reduced pressure. The resulting light-yellow oil was subjected to flash chromatography (silica, 1:2 v/v acetone/hexane) and concentration of the appropriate fractions (Rf = 0.2) afforded a solid that upon crystallisation (1:1 v/v CH2Cl2/hexane) gave compound 24 (7.0 mg, 33%) as fine, white needles (no mp, decomposition above 50 °C) [Found: (M+H)+, 325.1917. C20H24N2O2 requires (M+H)+, 325.1916]. 1H NMR (400 MHz, CDCl3) δ 7.62 (d, J = 7.5 Hz, 1H), 7.13 (t, J = 7.8 Hz, 1H), 6.67 (t, J = 7.5 Hz, 1H), 6.54 (d, J = 10.2 Hz, 1H), 6.42 (d, J = 7.8 Hz, 1H), 5.99 (d, J = 10.2 Hz, 1H), 3.86 (dd, J = 12.3 and 9.7 Hz, 1H), 3.50 (m, 1H), 3.22 (m, 1H), 2.77 (s, 3H), 2.65 (s, 1H), 2.38 (m, 1H), 2.09 (m, 1H), 1.76–1.57 (complex m, 6H), 0.82 (t, J = 8.0 Hz, 3H); 13C NMR (100 MHz, CDCl3) δ 162.2, 150.5, 146.5, 131.2, 128.1, 126.1, 122.5, 117.9, 106.7, 93.3, 68.1, 58.2, 44.0, 41.5, 32.2, 31.5, 27.6, 21.7, 19.0, 7.5; νmax 3352, 2934, 1657, 1601, 1480, 1446, 1373, 1331, 1299, 1234, 1156, 1067, 1025, 912, 859, 816, 754, 737 cm–1; Mass spectrum (ESI, +ve) m/z 347 (63%), 325 [(M+H)+,100], 307 (92).
Crystallographic Studies
Compound 10: C16H19NO, Mr = 241.33, T = 200 K, triclinic, space group Pī, Z = 2, a = 7.2598(3), b = 8.6985(3), c = 11.4581(5) Å; α = 100.373(2)°, β = 103.087(3)°, γ = 103.087(3)°; V = 665.75(5) Å3, Dx = 1.204 g cm–3, 3051 unique data (2θmax = 55°), R = 0.041 [for 2437 reflections with I > 2.0σ(I)]; Rw = 0.105 (all data), S = 0.98.
Oxalate Salt of Compound 14 [C17H23N2]+[C2HO4]– ·CH3OH, M = 376.45, T = 200 K, orthorhombic, space group Pbca, Z = 8, a = 11.1600(5), b = 13.6169(5), c = 27.9681(13) Å; V = 4250.2(3) Å3, Dx = 1.177 g cm–3, 3737 unique data (2θmax = 50°), R = 0.144 [for 1554 reflections with I > 2.0σ(I)]; Rw = 0.438 (all data), S = 1.00.
Compound 22: C20H24N2O, Mr = 308.42, T = 200 K, monoclinic, space group P21/c, Z = 4, a = 8.7799(1), b = 14.6530(2), c = 13.2854(2) Å; β = 100.9286(9)°; V = 1678.19(4) Å3, Dx = 1.221 g cm–3, 4907 unique data (2θmax = 60.2°), R = 0.044 [for 3971 reflections with I > 2.0σ(I)]; Rw = 0.114 (all data), S = 0.98.
Structure Determination. Images were measured on a Nonius Kappa CCD diffractometer (MoKα, graphite monochromator, λ = 0.71073 Å) and data extracted using the DENZO package.28 Structure solution was by direct methods (SIR92).29 The structures of compounds 10, the oxalate salt of 14 and 22 were refined using the CRYSTALS program package.30 Atomic coordinates, bond lengths and angles, and displacement parameters have been deposited at the Cambridge Crystallographic Data Centre [CCDC nos. 990090 (10), 990091 (oxalate salt of 14), and 990092 (22)]. These data can be obtained free-of-charge via www.ccdc.cam.ac.uk/data_request/cif, by emailing data_request@ccdc.cam.ac.uk, or by contacting The Cambridge Crystallographic Data Centre, 12 Union Road, Cambridge CB2 1EZ, UK; fax: +44 1223 336033.
ACKNOWLEDGEMENTS
We thank the Australian Research Council and the Institute of Advanced Studies for financial support. LVW is the grateful recipient of an APA Scholarship provided by the Australian Government.
SUPPORTING INFORMATION
Copies of the 1H and 13C NMR spectra for compounds 9–17 and 20–24, together with the ORTEPs arising from the single-crystal X-ray analyses of compound 10, the oxalate salt of compound 14, and compound 22 can be obtained, as a pdf, through the HETERORESOURCE website of the journal.
References
1. For useful points of entry into the relevant literature see: (a) H. Ishikawa, D. A. Colby, S. Seto, P. Va, A. Tam, H. Kakei, T. J. Rayl, I. Hwang, and D. L. Boger, J. Am. Chem. Soc., 2009, 131, 4904; CrossRef (b) D. Kato, Y. Sasaki, and D. L. Boger, J. Am. Chem. Soc., 2010, 132, 3685; CrossRef (c) S. Yokoshima, H. Tokuyama, and T. Fukuyama, Chem. Rec., 2010, 10, 101; CrossRef (d) E. K. Leggans, T. J. Barker, K. K. Duncan, and D. L. Boger, Org. Lett., 2012, 14, 1428; CrossRef (e) T. C. Turner, K. Shibayama, and D. L. Boger, Org. Lett., 2013, 15, 1100; CrossRef (f) J. D. White and Y. Li, Heterocycles, 2014, 88, 899. CrossRef
2. For a general review of this class of alkaloids see: ‘Antitumor Bisindole Alkaloids from Catharanthus Roseus (L.).’ The Alkaloids, Vol. 37, ed. by A. Brossi and M. Suffness, Academic Press, New York, 1990, pp. 1–240. CrossRef
3. (a) T. Miyazaki, S. Yokoshima, S. Simizu, H. Osada, H. Tokuyama, and T. Fukuyama, Org. Lett., 2007, 9, 4737; CrossRef (b) A. Tam, H. Gotoh, W. M. Robertson, and D. L. Boger, Bioorg. Med. Chem. Lett., 2010, 20, 6408; CrossRef (c) K. D. Schleicher, Y. Sasaki, A. Tam, D. Kato, K. K. Duncan, and D. L. Boger, J. Med. Chem., 2013, 56, 483. CrossRef
4. P. Keglevich, L. Hazai, G. Kalaus, and C. Szántay, Molecules, 2012, 17, 5893. CrossRef
5. M. G. Banwell, A. J. Edwards, D. W. Lupton, and G. Whited, Aust. J. Chem., 2005, 58, 14. CrossRef
6. As another aspect of our work in the area, we have devised methods for the synthesis of compounds related to the indole-indoline cores of vinblastine and vincristine: M. J. Harvey, M. G. Banwell, and D. W. Lupton, Tetrahedron Lett., 2008, 49, 4780. CrossRef
7. (a) M. G. Banwell and J. A. Smith, J. Chem. Soc., Perkin Trans. 1, 2002, 2613; CrossRef (b) M. G. Banwell and D. W. Lupton, Org. Biomol. Chem., 2005, 3, 213; CrossRef (c) M. G. Banwell, D. W. Lupton, and A. C. Willis, Aust. J. Chem., 2005, 58, 722; CrossRef (d) S. H. Tan, M. G. Banwell, A. C. Willis, and T. A. Reekie, Org. Lett., 2012, 14, 5621. CrossRef
8. For useful points of entry into the literature on syntheses of Aspidosperma alkaloids see: (a) H. Ishikawa, G. I. Elliot, J. Velcicky, Y. Chi, and D. L. Boger, J. Am. Chem. Soc., 2006, 128, 10596; CrossRef (b) J. W. Medley and M. Movassaghi, Angew. Chem. Int. Ed., 2012, 51, 4572; CrossRef (c) Z. Li, S. Zhang, S. Wu, X. Shen, L. Zou, F. Wang, X. Li, F. Peng, H. Zhang, and Z. Shao, Angew. Chem. Int. Ed., 2013, 52, 4117 and references cited therein. CrossRef
9. (a) A. Urrutia and J. G. Rodríguez, Tetrahedron, 1999, 55, 11095; CrossRef (b) J. V. Bjerrum, T. Ulven, and A. D. Bond, Acta Crystallogr., Sect. E: Struct. Rep. Online, 2009, 65, o579. CrossRef
10. P. Liu, J. Wang, J. Zhang, and F. G. Qiu, Org. Lett., 2011, 13, 6426. CrossRef
11. D. L. Comins and A. Dehghani, Tetrahedron Lett., 1992, 33, 6299. CrossRef
12. J. Eames, H. J. Mitchell, A. Nelson, P. O’Brien, S. Warren, and P. Wyatt, J. Chem. Soc., Perkin Trans. 1, 1999, 1095. CrossRef
13. (a) Y. Wu and P. Ahlberg, J. Org. Chem., 1992, 57, 6324; CrossRef (b) H.-G. Brünker and W. Adam, J. Am. Chem. Soc., 1995, 117, 3976. CrossRef
14. H.-J. Knölker, T. Braxmeier, and G. Schlechtingen, Angew. Chem. Int. Ed., 1995, 34, 2497. CrossRef
15. H. Munch, J. S. Hansen, M. Pittelkow, J. B. Christensen, and U. Boas, Tetrahedron Lett., 2008, 49, 3117. CrossRef
16. For a review dealing with various aspects of the chemical reactivity of isocyanates see: A. D. Allen and T. T. Tidwell, Chem. Rev., 2013, 113, 7287. CrossRef
17. For a review dealing with various aspects of the chemical reactivity of isothiocyanates see: A. K. Mukerjee and R. Ashare, Chem. Rev., 1991, 91, 1. CrossRef
18. U. Haberl, E. Steckhan, S. Blechert, and O. Wiest, Chem. Eur. J., 1999, 5, 2859. CrossRef
19. J. P. Knowles, L. D. Elliot, and K. I. Booker-Milburn, Beilstein J. Org. Chem., 2012, 8, 2025. CrossRef
20. For examples of this type of transformation see: A. Hassner, A. S. Amarasekara, and D. Andisik, J. Org. Chem., 1988, 53, 27. CrossRef
21. E. Herrero-Gómez, C. Nieto-Oberhuber, S. López, J. Benet-Buchholz, and A. M. Echavarren, Angew. Chem. Int. Ed., 2006, 45, 5455. CrossRef
22. K. Gilmore and I. V. Alabugin, Chem. Rev., 2011, 111, 6513. CrossRef
23. B. M. Trost and J. Quancard, J. Am. Chem. Soc., 2006, 128, 6314. CrossRef
24. For a variant on Trost’s protocol see: J. Chen and M. J. Cook, Org. Lett., 2013, 15, 1088. CrossRef
25. Of course, given the chiral, non-racemic nature of compound 4 any application of the protocols defined in Schemes 1, 2 and 3 (or variants of these) to this C-ring building block would necessarily lead to enantiomerically pure products.
26. W. C. Still, M. Kahn, and A. Mitra, J. Org. Chem., 1978, 43, 2923. CrossRef
27. A. B. Pangborn, M. A. Giardello, R. H. Grubbs, R. K. Rosen, and F. J. Timmers, Organometallics, 1996, 15, 1518. CrossRef
28. DENZO–SMN. Z. Otwinowski and W. Minor, ‘Processing of X-ray diffraction data collected in oscillation mode.’ In Methods in Enzymology, Volume 276: Macromolecular Crystallography, Part A; ed. by C. W. Carter Jr. and R. M. Sweet, Academic Press, New York, 1997, pp. 307–326.
29. SIR92. A. Altomare, G. Cascarano, C. Giacovazzo, A. Guagliardi, M. C. Burla, G. Polidori, and M. Camalli, J. Appl. Crystallogr., 1994, 27, 435.
30. P. W. Betteridge, J. R. Carruthers, R. I. Cooper, K. Prout, and D. J. Watkin, J. Appl. Crystallogr., 2003, 36, 1487. CrossRef