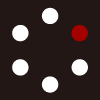
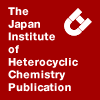
HETEROCYCLES
An International Journal for Reviews and Communications in Heterocyclic ChemistryWeb Edition ISSN: 1881-0942
Published online by The Japan Institute of Heterocyclic Chemistry
e-Journal
Full Text HTML
Received, 23rd January, 2014, Accepted, 7th February, 2014, Published online, 14th February, 2014.
DOI: 10.3987/COM-14-12948
■ Synthesis and Antimalarial Activity of Some Neocryptolepine Analogues Carrying a Multifunctional Linear and Branched Carbon-Side Chains
Elkhabiry Shaban, Kathryn J. Wicht, Ning Wang, Zhen-Wu Mei, Ikuya Hayashi, Ahmed Abdel Aleem El Gokha, Marcel Kaiser, Ibrahim El Tantawy El Sayed, Timothy J. Egan,* and Tsutomu Inokuchi*
Division of Chemsitry and Biochemistry, Graduate School of Natural Science and Technology, Okayama University, 3-1-1 Tsushima-naka, Okayama 700-8530, Japan
Abstract
The synthesis and in vitro antimalarial activity of several neocryptolepine analogues carrying either a linear or branched dibasic side chain at C11 are described. Many of these neocryptolepine analogues have low nanomolar antimalarial activity against the chloroquine-sensitive P. falciparum strain (NF54). The data also demonstrated that a branched structural motif is not superior for antimalarial activity over a linear side chain, but their thioureido derivatives showed lower cytotoxicity than the linear one. Ureido and thioureido derivatives also showed stronger β-haematin inhibition than the corresponding free amines.The emergence and spread of chloroquine-resistant Plasmodium falciparum parasites has been a major global health problem and contributes significantly to the continued high prevalence of malaria.1,2 New, safe, and effective drugs active against multidrug resistant P. falciparum strains are thus urgently needed.1,3 Medicinal plants have long been used for treating parasitic diseases, including malaria, and constitute an important source of new molecules for lead optimization programs, as exemplified by the success of artemisinin and its derivatives.4–9 Neocryptolepine, 1 (Figure 1), is an indolo[2,3-b]quinoline alkaloid isolated as a minor alkaloid alongside its major regio-isomer cryptolepine 2 from the roots of Cryptolepis sanguinolenta, a shrub used in traditional medicine for the treatment of malaria in Central and West Africa.10,11
In addition, neocryptolepine 1 also exerts a broad range of potential biological applications12–21 and appeared to have lower cytotoxicity compared to cryptolepine 2.22,23 However, several neocryptolepine analogues have been described to possess DNA intercalating activity and are reported as anticancer drugs.15,21 Based on our recent findings, it was speculated that substitution of neocryptolepine could be favorable for more potent and selective antimalarial activities and several series of substituted neocryptolepines have been synthesized.12–20 These promising results prompted us to investigate structure-activity relationships (SAR) with respect to the structural requirements of side-chains at C-11 of the neocryptolepine scaffold for improved antiplasmodial activity and selectivity relative to the lead compound, neocryptolepine 1. In this paper, we have explored a set of neocryptolepine analogues having diversified side-chains by varying the structure and length of the linker between the two nitrogen atoms as well as the substitution pattern and basicity of the distal amino group.
The synthetic strategy for neocryptolepine analogues 8a–g was based on nucleophilic aromatic substitution (SNAr) reactions of the key intermediate 11-chloro-substituted neocryptolepines 7 obtained via Scheme 1. This method was used for synthesis of neocryptolepines with substitutions on the B ring (C-11 position). Thus, a series of neocryptolepines with different side chains at C-11 were prepared starting from methyl 1H-indole-3-carboxylate (3) and N-methylaniline derivatives 4. The intermediate methyl 2-(phenylamino)-1H-indole-3-carboxylates 5 were obtained via chlorination with N-chlorosuccinimide in the presence of 1,4-dimethylpiperazine followed by addition of the aniline derivative as a trichloroacetate salt. Cyclization of 5 was achieved by heating in boiling diphenyl ether to afford 5,6-dihydro-11H-indolo[2,3-b]quinolin-11-ones 6, which were chlorinated with POCl3 to give 11-chloroneocryptolepines 7, the key intermediates for diversification. Subsequent amination of 7 with various 1,2-diaminoethanes and 1,3-diaminopropanes by heating yielded the target compounds 8a–g as depicted in Scheme 1.
The synthesized compounds 8a–g were evaluated for their in vitro antimalarial activity against the chloroquine-sensitive P. falciparum strain (NF54). The corresponding IC50 values together with their cytotoxicity determined using mammalian L6 cells are presented in Table 1. In this study, we have chosen the side chain portion using a propyl spacer (three carbons) for the aminoalkylamino substituent at the C11, because incorporation of such shorter side chain variant proved to be of important element for antimalarial activity.24,25 The side chains at C11 of 8 were introduced by the reaction of 7 with branched 1,2-diaminoethane or 1,3-diaminopropanes, and their in vitro antimalarial activity was compared with that of 7 with no branched aminoalkylamino-substituent at C-11. IC50 values for compounds 8a–g range from 11.8 to 232.5 nM, which represent a significant improvement in antiplasmodial activity over the neocryptolepine 1 (1580 nM), but not as good as the well-known antimalarial drugs, artemisinin (4.3 nM) and chloroquine (9.4 nM). Inspection of the data in Table 1 allows the following conclusions to be drawn. Firstly, compounds containing a linear 3-aminopropylamino group with a three carbon spacer, e.g., 8a, 8c, generally present better antiplasmodial activity than those with the corresponding branched chains, e.g., 8b, 8d. The type of pendant group residing on the spacer also has influence on antiplasmodial activity, for example, replacing the geminal dimethyl group in 8d with hydroxy group as in 8e substantially improved the antiplasmodial activity. Also the number of pendant methyl groups on the spacer has an influence as it appears when the results of side-chain with two carbon atom spacer and branched with one methyl group, e.g., 8f, and two geminal dimethyl groups, e.g., 8g, are compared. The results showed that such variation has a slight effect on activity but a remarkable effect on cytotoxicity and subsequently improved selectivity index (SI) against the parasite. For example, 8g has a SI of over 100 compared with 8e, which has a SI below 20. It should also be noted that the antiplasmodial activity as well as the selectivity indices increased by adding a chloro substituent at C-2 on the A-ring in combination with a 11-aminoalkylamino side-chain on the neocryptolepine core, as in compounds 8c and 8d, when compared with the corresponding nonchlorinated analogues 8a and 8b.
Based on the above results, we have further studied the influence of substituent modification around the terminal nitrogen atom in compounds 8 on antimalarial activity. In the previous paper, we demonstrated that the addition of a rigid ureido functionality at the terminus of the 3-aminopropylamino-substituent at C11 of the neocryptolepine core improved both the antimalarial activity and selectivity index.17 The ureido derivative of 8c showed an IC50 of 2.2 nM and a SI of 1400, which are 5.3 and 61 times higher than those of 8c, respectively. Accordingly, a series of ureido and thioureido derivatives 9, 10 were prepared in high yields by modification of free terminal amines of 8 with either phenylisocyanate or isothiocyanate in dry CH2Cl2 at room temperature, as shown below.
The antiplasmodial activities of compounds 9 and 10 were measured against P. falciparum (CQS: NF54 strain). All compounds within this series showed antimalarial activity with high selectivity indices and revealed promising drug discovery leads (Table 2). More importantly, ureido and thioureido derivatives 9b and 10b with branched three carbon spacers in combination with chlorine atom at C2 of the neocryptolepine core were the most active in this series (IC50 of 19.1 nM and 19.9 nM, respectively). It should be noted that compounds with a thioureido functionality are more potent than the corresponding ureido analogues. Comparing 9b with 10b, the antiplasmodial activities were similar, but 10b bearing a thioureido group showed a three times higher SI.
Some studies revealed that the effectiveness of CQ as an antimalarial drug may be ascribed to its capability of docking with the fastest growing face of the haemozoin crystal. The quinoline ring of CQ interacts with ferriprotoporphyrin IX (Fe(III)PPIX) by π-π stacking, and in addition the 4-amino group of CQ interacts with haematin by a weak hydrogen bonding interaction. The resulting haematin-CQ complex directly exerts a toxic effect on the parasite.26 We tested the β-haematin inhibition of the 11-(ω-aminoalkylamino)indolo[2,3-b]-quinolones and their ureido and thioureido derivatives. Compounds 8b, 8d, 8f and 8g with a branched ω-aminoalkylamino substituent showed weak β-haematin inhibition. The compound 8e with a hydroxylated pendant showed slightly increased β-haematin inhibition, which may be attributed to the improved hydrophilicity of the polar hydroxy group. Compounds 9 and 10 with ureido and thioureido functionalities showed greater β-haematin inhibition. In particular 9b showed the strongest β-haematin inhibition with IC50 value of 10.07 μM. The reason for the improved inhibition may be due to the increased π-π stacking ability of the derivatives containing ureido and thioureido groups with haem, resulting from the delocalized electrons in the planar urea/thiourea-phenyl systems.
In conclusion, we have prepared a novel series of neocryptolepine derivatives by systematically varying the structure and length of the linker between the two nitrogen atoms on the neocryptolepine core. All the synthesized compounds showed more potent antiplasmodial activities against CQS parasites (NF54 strain) in vitro when compared with neocryptolepine. A comparative study showed that compounds containing linear-side chains with three carbon atoms spacers between the two distal nitrogen generally present better antiplasmodial activities than those with branched carbon atoms spacers. Further variations in substituents and substitution patterns may be necessary to obtain nontoxic compounds showing better activity and selectivity.
EXPERIMENTAL
The 1H NMR, 13C NMR spectra were measured on the Varian INOVA-600 or Varian INOVA-400 spectrometer, using CDCl3 or DMSO-d6 as solvent and tetramethylsilane (TMS) as the internal standard.
General procedure for the synthesis of 11-aminoneocryptolpines 8a–8g: 11-Chloroindoloquinolines 7 (0.3 mmol) and an excess of the appropriate aminoalkylamine (3.0 mmol) were heated together at 120 °C for 4 h. TLC monitoring was used to ensure the completion of reaction. The resulting brown crude oil was purified by flash chromatography using AcOEt-2N ammonia in MeOH (9:1v/v) as an eluent to yield pure yellowish solids product.
N-(3-Amino-2,2-dimethylpropyl)-5-methyl-5H-indolo[2,3-b]quinolin-11-amine (8b): Yield 280 mg (84%), yellow solids; mp 112–114 °C; 1H NMR (300MHz, CDCl3) δ 8.39 (br s, 1H), 8.11 (d, J = 8.3 Hz, 1H), 7.99 (d, J = 7.8 Hz, 1H), 7.76 (d, J = 8.0 Hz, 1H), 7.61 (dt, J = 17.0, 8.5 Hz, 2H), 7.39–7.33 (m, 1H), 7.29–7.24 (m, 1H), 7.19–7.16 (m, 1H), 4.20 (s, 3H), 3.83 (s, 2H), 2.83 (s, 2H), 0.86 (s, 6H); 13C NMR (151MHz, CDCl3 ) δ 157.56, 152.53, 149.42, 138.20, 130.25 (d), 125.02, 124.20, 123.58, 122.22, 120.55, 118.59, 117.32, 116.20, 114.73, 104.53, 61.79, 53.35, 36.03, 32.95 (d), 23.92 (d) (2C). HRMS (ESI) calcd for C21H24N4 [M-H]-. Exact mass: 332.4421, found 331.4462.
N-(3-Amino-2,2-dimethylpropyl)-2-chloro-5-methyl-5H-indolo[2,3-b]quinolin-11-amine (8d): Yield 248 mg (95%), yellow solids; mp 177–179 °C; 1H NMR (400 MHz, CDCl3) δ 8.07 (d, J = 1.9 Hz, 1H), 7.97 (d, J = 8 Hz, 1H), 7.76 (d, J = 7.9 Hz, 1H), 7.54 (dd, J = 5.0, 4.1 Hz, 1H), 7.49 (t, J = 7.4 Hz, 1H), 7.41 (t, J = 7.6 Hz, 1H), 7.18 (t, J = 7.5 Hz, 1H), 4.18 (s, 3H), 3.78 (s, 2H), 2.84 (s, 2H), 0.86 (s, 6H); 13C NMR (101 MHz, CDCl3) δ 148.44, 136.82, 130.40 (2C), 126.31, 125.70 (2C), 124.69, 124.02 (2C), 122.47 (2C), 119.21, 117.54, 116.36, 62.08, 53.49, 36.01, 33.41, 24.09 (2C). HRMS (ESI) calcd for C21H23ClN4 [M-H]-. Exact mass: 366.1611, found 365.1624.
1-Amino-3-(2-chloro-5-methyl-5H-indolo[2,3-b]quinolin-11-ylamino)propan-2-ol (8e): Yield 290 mg (82%), yellow solids; mp 150–152 °C; 1H NMR (400 MHz, DMSO-d6) δ 8.56 (d, J = 2.1 Hz, 1H), 7.95 (d, J = 7.8 Hz, 1H), 7.85 (d, J = 9.2 Hz, 1H), 7.78 (dd, J = 9.1, 2.1 Hz, 1H), 7.50 (d, J = 7.8 Hz, 1H), 7.28 (t, J = 7.5 Hz, 1H), 7.07 (d, J = 14.2 Hz, 1H), 4.13 (s, 3H), 3.91–3.87 (m, 1H), 3.79 (dd, J = 12.8, 6.5 Hz, 2H), 3.69–3.66 (m, 2H); 13C NMR (101MHz, DMSO-d6) δ 156.17, 152.45, 147.55, 136.10, 130.17, 124.92 (2C), 123.91, 123.40, 122.21, 118.19, 117.01, 116.77, 116.67, 105.46, 70.97, 52.32, 45.41, 32.34. HRMS (ESI) calcd for C19H19ClN4O [M-H]-. Exact mass: 354.1247, found 353.1275.
N-(2-Aminopropyl)-2-chloro-5-methyl-5H-indolo[2,3-b]quinolin-11-amine (8f): Yield 297 mg (87%), yellow solids; mp 95–79 °C; 1H NMR (600 MHz, CDCl3) δ 8.10 (d, J = 5.7 Hz, 2H), 7.76 (d, J = 7.9 Hz, 1H), 7.61–7.59 (m, 1H), 7.54 (d, J = 9.1 Hz, 1H), 7.44 (t, J = 7.6 Hz, 1H), 7.22 (t, J = 7.5 Hz, 1H), 6.48 (br, 1H), 4.24–4.18 (m, 3H), 3.79 (d, J = 11.8 Hz, 1H), 3.40 (t, J = 6.0 Hz, 1H), 3.12 (dd, J = 4.4, 1.7 Hz, 1H), 1.10 (d, J = 6.3 Hz, 6H); 13C NMR (151MHz, CDCl3) δ 156.16, 152.66, 147.82 (d), 136.95 (d), 130.52, 130.41 (d), 127.01, 126.22 (d), 124.45, 121.75 (d), 119.41 (d), 117.57, 116.40 (d), 107.63, 55.20 (d), 47.90 (d), 33.17 (d), 22.95 (d). HRMS (ESI) calcd for C19H19ClN4 [M-H]-. Exact mass: 338.1298, found 337.1232.
N-(2-Amino-2-methylpropyl)-2-chloro-5-methyl-5H-indolo[2,3-b]quinolin-11-amine (8g): Yield 320 mg (90%), yellow solids; mp 108–110 °C; 1H NMR (400 MHz, CDCl3) δ 8.15 (dd, J = 12.5, 4.9 Hz, 2H), 7.76 (d, J = 8.0 Hz, 1H), 7.62 (dt, J = 19.8, 5.3 Hz, 2H), 7.44 (t, J = 7.6 Hz, 1H), 7.21 (t, J = 7.5 Hz, 1H), 6.73 (br, 1H), 4.22 (d, J = 0.4 Hz, 3H), 3.58 (d, J = 4.5 Hz, 2H), 1.11 (s, 6H); 13C NMR (101 MHz, CDCl3) δ 156.47, 152.91, 148.22, 137.17, 130.43 (d), 126.12 (2C), 124.58 (2C), 121.64 (2C) 119.40 (d), 117.67, 116.83 (d), 107.63, 59.14, 51.50, 33.16, 29.50 (2C). HRMS (ESI) calcd for C20H21ClN4 [M-H]-. Exact mass: 352.1455, found 351.1422.
General procedure for the synthesis of compounds 9a–9f: 2-Substituted 5-methyl-5H-indolo[2,3-b]quinolin-11-amine (8a–g, 50 mg) was completely dissolved in dry CH2CL2 (1 mL), and then a solution of phenylisocyanate or phenylisothiocyanate (1.1 equiv) and dry CH2CL2 (1 mL) were added drop by drop under stirring at room temperature for 2–4 h. Tlc monitoring was used to ensure the completion of reaction. The reaction mixture was evaporated to dryness under reduced pressure. The crude product was purified by flash chromatography using AcOEt-2N ammonia in MeOH (9:1 v/v) as an eluent to yield pure products as yellowish solids.
1-(2,2-Dimethyl-3-(5-methyl-5H-indolo[2,3-b]quinolin-11-ylamino)propyl)-3-phenylurea (9a): Yield 412 mg (91%), yellow solids; mp 154–156 °C; 1H NMR (600 MHz, CDCl3) δ 9.25 (s, 1H), 8.42 (d, J = 5.7 Hz, 1H), 7.74–7.70 (m, 2H), 7.64 (d, J = 5.6 Hz, 1H), 7.47 (s, 2H), 7.40–7.35 (m, 2H), 7.24–7.22 (m, 2H), 7.14 (d, J = 2.8 Hz, 1H), 6.97 (d, J = 2.9 Hz, 2H), 6.77 (s, 1H), 6.64 (s, 1H), 4.03 (d, J = 2.8 Hz, 3H), 3.60 (s, 2H), 3.25 (s, 2H), 2.29 (br, 1H), 0.63 (d, J = 2.2 Hz, 6H); 13C NMR (151MHz, CDCl3) δ 158.01, 157.18, 151.56, 149.62, 140.05, 137.95, 130.96, 129.53 (2C), 126.04, 124.40, 124.25, 123.19, 122.67, 121.99, 120.00 (2C), 119.73, 116.93, 116.59, 115.04, 106.85, 55.71, 48.02, 38.61, 33.30, 24.07 (2C). HRMS (ESI) calcd for C28H29N5O [M-H]-. Exact mass: 451.2372, found 450.2364.
1-(3-(2-Chloro-5-methyl-5H-indolo[2,3-b]quinolin-11-ylamino)-2,2-dimethylpropyl)-3-phenylurea (9b): Yield 410 mg (84%), yellow solids; mp 232–234 °C; 1H NMR (400 MHz, CDCl3) δ 8.37 (s, 1H), 8.13 (br, 1H), 7.73–7.70 (m, 2H), 7.60–7.57 (m, 1H), 7.45 (dd, J = 8.0, 4.7 Hz, 3H), 7.39 (t, J = 7.4 Hz, 1H), 7.14–7.06 (m, 2H), 7.31 (t, J = 7.2 Hz, 2H), 6.53 (br, 1H), 5.91 (br, 1H), 4.12 (d, J = 5.5 Hz, 3H), 3.57 (d, J = 6.2 Hz, 2H), 3.31 (d, J = 6.0 Hz, 2H), 0.73 (s, 2H), 0.73 (s, 6H); 13C NMR (101 MHz, CDCl3) δ 157.75, 156.80,148.24, 139.72, 136.45, 130.92, 129.70 (2C), 127.36, 126.62, 124.04 (2C), 123.94, 123.75, 122.68, 120.69 (2C), 120.01, 117.92, 116.98, 116.46, 106.85, 55.97, 48.31, 38.44, 33.49, 24.18 (2C). HRMS (ESI) calcd for C28H28ClN5O [M-H]-. Exact mass: 485.1982, found 484.1918.
1-(2,2-Dimethyl-3-(5-methyl-5H-indolo[2,3-b]quinolin-11-ylamino)propyl)-3phenylthiourea (10a): Yield 42 mg (90%), yellow solids; mp 172–174 °C; 1H NMR (600 MHz, CDCl3) δ 8.54 (d, J = 7.7 Hz, 1H), 7.86 (d, J = 7.0 Hz, 1H), 7.76 (dd, J = 15.1, 7.2 Hz, 2H), 7.66 (d, J = 8.1 Hz, 1H), 7.46 (t, J = 6.7 Hz, 1H), 7.39 (dd, J = 17.1, 8.2 Hz, 3H), 7.33 (d, J = 6.4 Hz, 2H), 7.24 (dd, J = 18.7, 11.6 Hz, 1H), 6.89 (s, 1H), 4.24 (s, 3H), 3.79 (d, J = 4.2 Hz, 2H), 3.73 (d, J = 4.2 Hz, 2H), 1.96 (br, 1H), 0.72 (s, 6H); 13C NMR (151 MHz, CDCl3) δ 182.53, 153.42, 151.07, 145.53, 138.59, 137.49, 132.00, 129.50 (2C), 126.44, 126.24, 125.25 (2C), 125.01, 123.39, 123.16, 122.73, 121.14, 117.18, 115.42, 115.19, 103.55, 54.75, 51.84, 39.27 (d), 34.68, 24.30 (d) (2C). HRMS (ESI) calcd for C28H29N5S [M-H]-. Exact mass: 467.2144, found 466.2170.
1-(3-(2-Chloro-5-methyl-5H-indolo[2,3-b]quinolin-11-ylamino)-2,2-dimethylpropyl)-3-phenylthiourea (10b): Yield 401 mg (79%), yellow solids; mp 155–157 °C; 1H NMR (600 MHz, CDCl3) δ 8.56 (br, 1H), 8.50 (d, J = 3.8 Hz, 1H), 7.87–7.85 (m, 1H), 7.73–7.71 (m, 1H), 7.60 (dd, J = 8.7, 6.3 Hz, 1H), 7.51 (dd, J = 9.0, 6.2 Hz, 1H), 7.42–7.37 (m, 3H), 7.31 (ddd, J = 10.8, 9.8, 4.6 Hz, 3H), 7.19 (dd, J = 13.6, 6.8 Hz, 1H), 6.61 (br, 1H), 4.15 (d, J = 6.2 Hz, 3H), 3.76 (d, J = 5.9 Hz, 2H), 3.65 (t, J = 5.8 Hz, 2H), 1.99 (br, 1H), 0.66 (d, J = 6.0 Hz, 6H); 13C NMR (151MHz, CDCl3) δ 182.15, 157.42, 152.91, 147.77, 136.64, 136.55, 130.86, 130.67 (2C), 128.15, 127.22, 126.51, 126.00 (2C), 124.11 (2C), 122.96, 119.60, 117.89, 117.53, 116.46, 108.04, 54.79, 52.92, 39.13, 33.42, 24.15 (2C). HRMS (ESI) calcd for C28H28ClN5S [M-H]-. Exact mass: 501.1754, found 500.1777.
References
1. A. Martinelli, R. Moreira, and P. V. L. Cravo, Mini-Rev. Med. Chem., 2008, 8, 201. CrossRef
2. D. A. Fidock, R. T. Eastman, S. A. Ward, and S. R. Meshnick, Trends Parasitol., 2008, 24, 537. CrossRef
3. R. G. Ridley, Nature, 2002, 415, 686. CrossRef
4. K. Kaur, M. Jain, T. Kaur, and R. Jain, Bioorg. Med. Chem., 2009, 17, 3229. CrossRef
5. V. Kumar, A. Mahajan, and K. Chibale, Bioorg. Med. Chem., 2009, 17, 2236. CrossRef
6. A. U. Krettli, J. O. Adebayo, and L. G. Krettli, Curr. Drug Targets, 2009, 10, 261. CrossRef
7. M. A. van Agtmael, T. A. Eggelte, and C. J. van Boxtel, Trends Pharmacol. Sci., 1999, 20, 199. CrossRef
8. P. G. Bray, S. A. Ward, and P. M. O’Neill, Drugs Dis. Post-Genom. Biol., 2005, 295, 3.
9. Y. J. Xu and L. Pieters, Mini-Rev. Med. Chem., 2013, 13, 1056. CrossRef
10. K. Cimanga, T. D. Bruyne, L. Pieters, M. Claeys, and A. Vlietinck, Tetrahedron Lett., 1996, 37, 1703. CrossRef
11. E. Kumar, J. R. Etukala, and S. Y. Ablordeppey, Mini-Rev. Med. Chem., 2008, 8, 538. CrossRef
12. I. El Sayed, P. V. Veken, L. Dhooghe, S. Hostyn, G. Van Baelen, G. Lemière, B. U. W. Maes, P. Cos, L. Maes, J. Joossens, A. Haemers, L. Pieters, and K. Augustyns, J. Med. Chem., 2009, 52, 2979. CrossRef
13. T. H. M. Jonckers, S. Van Miert, C. Kanyanga, C. Bailly, P. Colson, M. C. De Pauw-Gillet, H. van Den Heuvel, M. Claeys, F. Lemiere, E. L. Esmans, J. Rozenski, L. Quirijnen, L. Maes, R. Dommisse, G. L. F. Lemière, A. Vlietinck, and L. Pieters, J. Med. Chem., 2002, 45, 3497. CrossRef
14. J. Lavrado, R. Moreira, and A. Paulo, Curr. Med. Chem., 2010, 17, 2348. CrossRef
15. W. J. Lu, M. Świtalska, L. Wang, M. Yonezawa, I. E. El Sayed, J. Wietrzyk, and T. Inokuchi, Med. Chem. Res., 2013, 22, 4492. CrossRef
16. T. P. Prakash, S. P. Perunninakulath, and G. T. Santosh, Curr. Org. Chem., 2011, 15, 1036. CrossRef
17. Z. W. Mei, L. Wang, W. J. Lu, C. Q. Pang, T. Maeda, W. Peng, M. Kaiser, I. E. El Sayed, and T. Inokuchi, J. Med. Chem., 2013, 56, 1431. CrossRef
18. W. J. Lu, K. J. Wicht, L. Wang, K. Imai, Z. W. Mei, M. Kaiser, I. E. El Sayed, T. J. Egan, and T. Inokuchi, Eur. J. Med. Chem., 2013, 64, 498. CrossRef
19. I. El Sayed, F. Ramzy, S. William, M. El Bahanasawy, and M. M. Abdel-Staar, Med. Chem. Res., 2012, 21, 4219. CrossRef
20. S. El Bardicy, I. El-Sayed, F. Yousif, M. El Bahansawye, P. Van der Veken, A Haemers, K. Augustyns, and L. Pieters, Pharm. Biol., 2012, 50, 134. CrossRef
21. L. Wang, M. Świtalska, Z. W. Mei, W. J. Lu, Y. Takahara, X. W. Feng, I. E. Sayed, J. Wietrzyk, and T. Inokuchi, Bioorg. Med. Chem., 2012, 20, 4820. CrossRef
22. C. Bailly, W. Laine, B. Baldeyrou, M. C. De Pauw-Gillet, P.Colson, C. Houssier, K. Cimanga, S. Van Miert, A. J. Vlietinck, and L. Pieters, Anti-Cancer Drug Des., 2000, 15, 191.
23. L. Dassonneville, A. Lansiaux, A. Wattelet, N. Wattez, C. Mahieu, S. Van Miert, L. Pieters, and C. Bailly, Eur. J. Pharmacol., 2000, 409, 9. CrossRef
24. P. A. Stocks, K. J. Raynes, P. G. Bray, B. K. Park, P. M. O'Neill, and S. A. Ward, J. Med. Chem., 2002, 45, 4975. CrossRef
25. D. Y. De, F. M. Krogstad, F. B. Cogswell, and D. J. Krogstad, Am. J. Trop. Med. Hygien., 1996, 55, 579.
26. P. M. O'neill, P. G. Bray, S. R. Hawley, S. A. Ward, and B. K. Park, Pharmacol. Therapeut., 1998, 77, 29 CrossRef