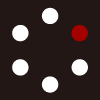
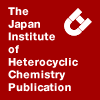
HETEROCYCLES
An International Journal for Reviews and Communications in Heterocyclic ChemistryWeb Edition ISSN: 1881-0942
Published online by The Japan Institute of Heterocyclic Chemistry
e-Journal
Full Text HTML
Received, 29th July, 2014, Accepted, 14th August, 2014, Published online, 20th August, 2014.
DOI: 10.3987/COM-14-S(K)95
■ A Concise Approach to Tetracyclic Spiroamine Scaffold of Erythrinan Alkaloids via an Oxidative Dearomatization-Spirocyclization Sequence
Emi Saito, Akihiko Nakamura, and Masahisa Nakada*
Department of Chemistry and Biochemistry, School of Advanced Science and Engineering, Waseda University, 3-4-1 Okubo, Shinjuku-ku, Tokyo 169-8555, Japan
Abstract
This paper describes a novel synthetic approach to 11,12-dimethoxy-8,9-dihydro-1H-indolo[7a,1-a]isoquinoline-2,6-dione, which is a key synthetic intermediate to some erythrinan alkaloids. This concise approach features an oxidative dearomatization-spirocyclization sequence mediated by phenyliodine (III) bis(trifluoroacetate) (PIFA) that efficiently forms the tetracyclic spiroamine scaffold. An unusual solvent effect in the oxidative dearomatization-spirocyclization sequence is also described.Some erythrinan alkaloids feature the unique tetracyclic spiroamine scaffold shown in Figure 1.1 Dienoid-type erythrinan alkaloids are a major group of the Erythrina family and have drawn significant attention from the synthetic community owing to their unique structures and intriguing bioactivities.1 Erythraline, erysodine, and erysotrine (Figure 1) show in vitro cytotoxic activity against Hep-G2 cells with an IC50 = 17.6, 11.8, and 15.8 µg/mL, and against HEp-2 cells with an IC50 = 15.90, 19.90, and 21.60 µg/mL, respectively.2 A number of syntheses of erythrinan alkaloids have been reported;1 however, these unique and complex structures continue to offer a challenge for new and efficient syntheses.
Herein, we report a concise approach to 11,12-dimethoxy-8,9-dihydro-1H-indolo[7a,1-a]isoquinoline-2,6-dione, a key intermediate in the synthesis of dienoid-type erythrinan alkaloids via a dearomatization-spirocyclization sequence mediated by phenyliodine (III) bis(trifluoroacetate) (PIFA).
Our retrosynthetic analysis for erysotrine is shown in Scheme 1. Erysotrine will be derived from 11,12-dimethoxy-8,9-dihydro-1H-indolo[7a,1-a]isoquinoline-2,6-dione (1), which has been reported3 as a late-stage synthetic intermediate to erysotrine. Compound 1 will be obtained by acidic hydrolysis of alkenyl ether 2, which will be prepared by an intramolecular aldol condensation of compound 3. Spirocycle 3 was envisioned to be formed by an oxidative dearomatization-spirocyclization sequence4,5 from o-hydroxyaniline derivative 4, which could be prepared from compound 6 after acetylation. Compound 6 was expected to be obtained by coupling commercially available homoveratrylamine 7 with aryl bromide 8.6 Hence, the synthesis of compound 1 was commenced with the assembly of compounds 7 and 8.
Aryl bromide 8 was prepared from p-methoxyphenol 9 as shown in Scheme 2. Monobromination of 9 afforded o-bromo-p-methoxyphenol 10 (88%) that was subsequently treated with MOMCl to afford compound 8 (94%). Several different reaction conditions were attempted for the coupling reaction between primary amine 7 and aryl bromide 8; ultimately, Buchwald–Hartwig amination6 afforded compound 6 in 91% yield. Acetylation of aniline 6 gave compound 5 (96%), and subsequent removal of the MOM group under acidic conditions afforded o-hydroxyaniline derivative 4 (75%).
With compound 4 in hand, the oxidative dearomatization-spirocyclization sequence was examined (Table 1). The oxidative dearomatization of 4 was initially carried out using PhI(OCOCF3)2 (PIFA) in dichloromethane (CH2Cl2) (entry 1), but unfortunately this reaction resulted in a complex mixture. An unusual solvent effect was observed when a mixture of 2,2,2-trifluoroethanol (CF3CH2OH)7 and CH2Cl2 was employed as the solvent. When the oxidative dearomatization of 4 was carried out in CF3CH2OH and CH2Cl2 (1/1, v/v) at 0 °C for 30 min, the desired product 3 was afforded as a single isomer in 54% yield (entry 2). The highly regioselective spirocyclization is well explained by the steric strain between the methoxy group on the dimethoxybenzene moiety and the dearomatized o-hydroxyaniline moiety in the transition state which leads to another isomeric product. Changing the solvent to CF3CH2OH (entry 3) increased the yield to 80%.
Formation of trifluoroethoxy adducts 3a and 3b (Figure 2) was observed for entries 2 and 3, indicating that the first step of the reaction was oxidative dearomatization by PIFA, followed by spirocyclization. Thus, compounds 3a and 3b likely arise from trapping of the cationic intermediate generated from PIFA oxidation of 4 by CF3CH2OH.
We also examined the reaction of phosphonate 4a (Figure 2), as alternative for the formation of the B-ring after the spirocyclization. Unfortunately, the reaction of compound 4a with PIFA afforded a complex mixture. Hence, compound 3 was used for further transformations.
Next, formation of the B-ring via an intramolecular aldol reaction of compound 3 was examined. Various bases were screened and treatment of 3 with 2 M NaOH in 1,4-dioxane and heating to reflux produced the desired intramolecular aldol reaction and subsequent dehydration to afford compound 2 in one-pot (52%) (Scheme 3). Finally, conjugated alkenyl ether 2 was hydrolyzed under acidic conditions (1 M HCl in THF at 60 °C) to afford ketone 1 in 91% yield, which was identical to the reported compound3 in all respects. Conversion of compound 1 to erythrinan alkaloids including erysotramidine and erysotrine has been reported, therefore completing our formal total syntheses of these compounds.
In summary, a new approach to 11,12-dimethoxy-8,9-dihydro-1H-indolo[7a,1-a]isoquinoline-2,6-dione, which is a key synthetic intermediate to erythrinan alkaloids, has been developed. This concise approach features a PIFA-mediated oxidative dearomatization-spirocyclization sequence that is solvent dependent. This sequence allows efficient access to the tetracyclic spiroamine scaffold of erythrinan alkaloids, and could provide access to other types of erythrinan alkaloids. Enantioselective synthesis of the spirocyclic intermediates could be solved using a chiral phenyliodine (III) reagent. Hence, we are now investigating asymmetric catalysis for the oxidative dearomatization-spirocyclization sequence.
EXPERIMENTAL
1H and 13C NMR spectra were recorded on a JEOL AL-400 spectrometer. 1H and 13C chemical shifts are reported in ppm downfield from tetramethylsila
ne (TMS, δ scale) with the solvent resonances as internal standards. The following abbreviations were used to explain the multiplicities: s, singlet; d, doublet; t, triplet; q, quartet; m, multiplet; band, several overlapping signals; br, broad. IR spectra were recorded on a JASCO FT/IR-8300. Melting points (mp) are uncorrected, recorded on a Yamato capillary melting point apparatus. Mass spectra and elemental analyses were provided at the Materials Characterization Central Laboratory, Waseda University. All reactions were carried out under an argon atmosphere with dry, freshly distilled solvents under anhydrous conditions, unless otherwise noted. All reactions were monitored by thin-layer chromatography carried out on 0.25 mm E. Merck silica gel plates (60F-254) using UV light as visualizing agent and phosphomolybdic acid and heat as developing agents. E. Kanto Chemical Silica Gel 60N (spherical, neutral, 63-210 μm or 40-50 μm partial size) was used for flash chromatography. THF was distilled from sodium/benzophenone ketyl. 1,4-Dioxane was dried with CaCl2 and distilled from sodium and LiAlH4. Toluene was distilled from sodium. CH2Cl2 was distilled from CaH2. MeOH was distilled with a small amount of magnesium and I2. 2,2,2-Trifluoroethanol (Wako) was purchased from Wako and used as received. All reagents were purchased from Aldrich, TCI, Wako, or Kanto Chemical Co. Ltd.
2-Bromo-4-methoxyphenol (10). Bromine (2.18 mL, 42.3 mmol) was added dropwise to a stirred solution of 4-methoxyphenol 9 (5.00 g, 40.3 mmol) in dry CH2Cl2 (67.1 mL). The resulting mixture was stirred at room temperature for 20 h (evolved HBr was trapped with H2O). The reaction mixture was quenched with saturated aqueous Na2SO3 solution (70 mL). The organic layer was washed with brine, dried over Na2SO4 and filtered. The solvent was removed under reduced pressure, and the residue was purified by silica gel flash column chromatography with EtOAc/hexanes (1:50 to 1:4) to give 10 (7.23 g, 35.6 mmol, 88% yield) as a white needle. Rf = 0.37 (silica gel, EtOAc/hexanes 1:4); mp 42 °C (CH2Cl2); IR (neat) 3286, 1590, 1498, 1421, 1267, 1199, 1031, 860, 745 cm-1; 1H NMR (400 MHz, CDCl3) δ 7.01 (d, J = 2.7 Hz, 1H), 6.94 (d, J = 9.2 Hz, 1H), 6.80 (dd, J = 9.2, 2.7 Hz, 1H), 5.11 (br s, 1H), 3.75 (s, 3H); 13C NMR (100 MHz, CDCl3) δ 160.0, 146.6, 116.9, 116.4, 115.5, 110.0, 56.1; HRMS calcd for C7H7BrO2 [M-]: 200.9551, found 200.9557.
2-Bromo-4-methoxy-1-methoxymethoxybenzene (8). To a stirred solution of 10 (3.00 g, 14.8 mmol) in CH2Cl2 (73.9 mL) was added N,N-diisopropylethylamine (3.06 mL, 17.7 mmol) and chloromethyl methyl ether (1.35 mL, 17.7 mmol). The resulting mixture was stirred at room temperature for 16 h, and then quenched with saturated aqueous NH4Cl solution (75 mL). After extracting the aqueous layer with CH2Cl2 (3 × 50 mL), the combined organic layer was dried over Na2SO4 and filtered. The solvent was removed under reduced pressure, and the residue was purified by silica gel flash column chromatography with EtOAc/hexanes (1:50 to 1:30) to give 8 (3.44 g, 13.9 mmol, 94% yield) as a colorless oil. Rf = 0.45 (silica gel, EtOAc/hexanes 1:4); IR (neat) 1489, 1216, 1197, 1081, 1031, 969, 920, 861, 729 cm-1; 1H NMR (400 MHz, CDCl3) δ 7.11 (d, J = 3.2 Hz, 1H), 7.08 (d, J = 9.2 Hz, 1H), 6.80 (dd, J = 9.2, 3.2Hz, 1H), 5.16 (s, 2H), 3.76 (s, 3H), 3.53 (s, 3H); 13C NMR (100 MHz, CDCl3) δ 155.2, 148.1, 118,6, 118.0, 114.1, 113.7, 96.2, 56.5, 55.9; HRMS calcd for C9H11BrO3Na [M + Na]+: 268.9789, found 268.9784.
N-(3,4-Dimethoxyphenethyl)-5-methoxy-2-methoxymethoxybenzeneamine (6). To a stirred solution of 8 (1.00 g, 4.05 mmol) in toluene (16.2 mL) was added homoveratrylamine 7 (0.73 mL, 4.45 mmol), sodium tert-butoxide (1.17 g, 12.1 mmol), Pd2(dba)3 (185 mg, 0.20 mmol), and (S)-BINAP (302 mg, 0.49 mmol) successively. The resulting mixture was heated to 90 °C. After 3 h, the mixture was cooled to room temperature, diluted with Et2O and filtered through a pad of Celite. The filtrate was concentrated, and the residue was purified by silica gel flash column chromatography with EtOAc/hexanes (1:8 to 1:4) to give 6 (1.28 g, 3.68 mmol, 91% yield) as a yellow solid. Rf = 0.14 (silica gel, EtOAc/hexanes 1:4); mp 42-44 °C (CH2Cl2); IR (neat) 3431, 1614, 1510, 1260, 1236, 1210, 1150, 1133, 1000, 817 cm-1; 1H NMR (400 MHz, CDCl3) δ 6.89 (d, J = 8.7 Hz, 1H), 6.84-6,72 (m, 3H), 6.26 (d, J = 2.7 Hz, 1H), 6.13 (dd, J = 8.7, 2.7 Hz, 1H), 5.04 (s, 2H), 4.33 (br s, 1H), 3.86 (s, 3H × 2), 3.76 (s, 3H), 3.42 (s, 3H), 3.37 (t, J = 6.9 Hz, 2H), 2.89 (t, J = 6.9 Hz, 2H); 13C NMR (100 MHz, CDCl3) δ 156.0, 149.0, 147.7, 140.0, 139.1, 132.1, 120.8, 115.4, 112.1, 111.5, 99.3, 98.3, 96.1, 56.1, 56.0, 55.9, 55.5, 45.0, 35.1; HRMS calcd for C19H25NO5Na [M + Na]+: 370.1630, found 370.1625.
N-(3,4-Dimethoxyphenethyl)-N-(5-methoxy-2-(methoxymethoxy)phenyl)acetamide (5). To a stirred solution of 6 (1.00 g, 2.88 mmol) in CH2Cl2 (14.4 mL) was added Et3N (0.56 mL, 4.03 mmol). The reaction mixture was cooled to 0 °C and AcCl (0.25 mL, 3.45 mmol) was added slowly. After stirring at room temperature for 6 h, the mixture was concentrated. To the residue was added water (10 mL), and the aqueous layer was extracted with EtOAc (3 × 15 mL). The combined organic layer was washed with 0.5 M HCl, saturated aqueous NaHCO3 solution, brine, dried over Na2SO4, and filtered. The solvent was removed under reduced pressure, and the residue was purified by silica gel flash column chromatography with EtOAc/hexanes (1:2, 1:1, and 2:1) to give 5 (1.08 g, 2.77 mmol, 96% yield) as a pale yellow oil. Rf = 0.09 (silica gel, EtOAc/hexanes 1:2); IR (neat) 1655, 1503, 1260,1234, 1216, 1196, 1153, 1028, 984, 806 cm-1; 1H NMR (400 MHz, CDCl3) δ 7.15 (d, J = 9.2 Hz, 1H), 6.84 (dd, J = 9.2, 3.2 Hz, 1H), 6.79-6.69 (m, 3H), 6.52 (d, J = 3.2 Hz, 1H), 5.13 (d, J = 6.9 Hz, 1H), 5.10 (d, J = 6.9 Hz, 1H), 4.09 (ddd, J = 13, 10, 5.5 Hz, 1H), 3.84 (s, 3H × 2), 3.74 (s, 3H), 3.58 (ddd, J = 13, 10, 6.0 Hz, 1H), 3.45 (s, 3H), 2.89 (ddd, J = 13, 10, 6.0 Hz, 1H), 2.77 (ddd, J = 13, 10, 5.5 Hz, 1H), 1.85 (s, 3H); 13C NMR (100 MHz, CDCl3) δ 171.1, 154.6, 148.9, 147.5, 147.3, 133.2, 131.8, 120.8, 116.6, 115.4, 114.3, 112.2, 111.3, 95.2, 56.3, 56.0, 55.9, 55.8, 50.2, 33.7, 22.3; HRMS calcd for C21H27NO6Na [M + Na]+: 412.1736, found 412.1731.
N-(3,4-Dimethoxyphenethyl)-N-(2-hydroxy-5-methoxyphenyl)acetamide (4). To a stirred solution of 5 (1.06 g, 2.72 mmol) in MeOH (13.6 mL) was added conc. HCl (2 drops) and heated to reflux. After 17 h, the mixture was cooled to room temperature, and the crude product was precipitated. The precipitate was filtered, washed with Et2O to give 4 (470 mg, 1.36 mmol) as a white solid. The solid was sufficiently pure and used for the next step without further purification. The filtrate was concentrated and purified by silica gel flash column chromatography with EtOAc/hexanes (1:2 to 1:1) to give another crop of compound 4 (237 mg, 0.69 mmol, 75% total yield) as a white solid. Rf = 0.24 (silica gel, EtOAc/hexanes 2:1); mp 169-170 °C (EtOAc/hexanes); IR (neat) 3071, 1636, 1503, 1443, 1317, 1237, 1157, 1025, 863, 816 cm-1; 1H NMR (400 MHz, CDCl3) δ 6.91 (d, J = 9.2 Hz, 1H), 6.84 (dd, J = 9.2, 2.7 Hz, 1H), 6.78 (d, J = 8.7 Hz, 1H), 6.73 (d, J = 2.3 Hz, 1H), 6.72 (d, J = 8.7 Hz, 1H), 6.51 (d, J = 2.7 Hz, 1H), 3.96-3.88 (m, 2H), 3.85 (s, 3H), 3.83 (s, 3H), 3.74 (s, 3H), 2.95-2.78 (m, 2H), 1.85 (s, 3H); 13C NMR (100 MHz, CDCl3) δ 172.3, 153.6, 149.1, 147.7, 146.5, 131.1, 129.6, 127.8, 118.1, 115.6, 114.0, 112.0, 111.4, 56.0, 55.95, 55.91, 49.6, 33.4, 22.5; HRMS calcd for C19H23NO5Na [M + Na]+: 368.1474, found 368.1479.
2'-Acetyl-3,6',7'-trimethoxy-3',4'-dihydro-2'H-spiro[cyclohexane-1,1'-isoquinoline]-2,4-dien-6-one (3). To a stirred solution of 4 (75.1 mg, 0.22 mmol) in 2,2,2-trifluoroethanol (4.3 mL) was added phenyliodine (III) bis(trifluoroacetate) (PIFA) (103.0 mg, 0.24 mmol) at 0 °C. The reaction mixture was stirred at 0 °C for 30 min. and powdered NaHCO3 was added to the reaction mixture. Stirring was continued at 0 °C for another 1 h and then filtered through a pad of Celite. The filtrate was concentrated and the residue was purified by silica gel flash column chromatography with EtOAc/hexanes (2:1, 4:1, and 8:1) to give 3 (60.0 mg, 0.17 mmol, 80% yield) as a yellow solid. Rf = 0.06 (silica gel, EtOAc/hexanes 2:1); mp 225-226 °C (EtOH); IR (neat) 1672, 1637, 1514, 1403, 1246, 1213, 1009, 860, 796, 560 cm-1; 1H NMR (400 MHz, CDCl3) δ 6.87 (dd, J = 10, 3.2 Hz, 1H), 6.64 (s, 1H), 6.61 (s, 1H), 6.15 (d, J = 10 Hz, 1H), 5.12 (d, J = 3.2 Hz, 1H), 4.06 (dt, J = 12, 3.7 Hz, 1H), 3.84 (s, 3H), 3.74 (s, 3H), 3.61 (s, 3H), 3.47 (dt, J = 12, 2.7 Hz, 1H), 3.25 (ddd, J = 15, 12, 3.7 Hz, 1H), 2.75 (dt, J = 15, 2.7 Hz, 1H), 2.18 (s, 3H); 13C NMR (100 MHz, CDCl3) δ 195.9, 168.6, 152.0, 148.6, 148.1, 139.2, 127.5, 127.2, 125.2, 112.0, 108.9, 107.6, 64.9, 56.2, 56.0, 55.1, 43.3, 29.8, 22.7; HRMS calcd for C19H21NO5Na [M + Na]+: 366.1317, found 366.1312.
2,11,12-Trimethoxy-8,9-dihydro-6H-indolo[7a,1-a]isoquinolin-6-one (2). To a stirred solution of 3 (100 mg, 0.29 mmol) in 1,4-dioxane (0.5 mL) was added 2M NaOH (1 mL) and heated to reflux. After 10 h, the reaction mixture was concentrated, and the residue was extracted with CH2Cl2 (3 × 1 mL), dried over Na2SO4 and filtered. The solvent was removed under reduced pressure, and the residue was purified by silica gel flash column chromatography with EtOAc/hexanes (1:2 to 1:1) to give 2 (49 mg, 0.15 mmol, 52%) as a pale yellow solid. Rf = 0.52 (silica gel, EtOAc); mp 117-120 °C (CH2Cl2/hexanes); IR (neat) 1670, 1514, 1454, 1256, 1221, 1104, 1076, 1011, 857, 800 cm-1; 1H NMR (400 MHz, CDCl3) δ 7.41 (s, 1H), 6.71 (d, J = 10 Hz, 1H), 6.55 (s, 1H), 6.09 (dd, J = 10, 2.3 Hz, 1H), 5.97 (s, 1H), 5.34 (d, J = 2.3 Hz, 1H), 4.35 (ddd, J = 13, 6.4, 1.8 Hz, 1H), 3.83 (s, 3H), 3.79 (s, 3H), 3.55 (s, 3H), 3.23 (ddd, J = 13, 12, 4.6 Hz, 1H), 3.04 (ddd, J = 16, 12, 6.4 Hz, 1H), 2.57 (ddd, J = 16, 4.6, 1.8 Hz, 1H); 13C NMR (100 MHz, CDCl3) δ 172.2, 161.7, 153.3, 148.9, 147.4, 129.6, 129.5, 124.6, 123.8, 121.9, 111.6, 108.2, 103.9, 69.8, 56.1, 55.9, 54.8, 36.4, 27.9; HRMS calcd for C19H19NO4Na [M + Na]+: 348.1212, found 348.1206.
11,12-Dimethoxy-8,9-dihydro-1H-indolo[7a,1-a]isoquinoline-2,6-dione (1). To a solution of 2 (40 mg, 0.12 mmol) in THF (0.6 mL) was added 1M HCl (0.6 mL). The reaction mixture was stirred at 60 °C for 7 h, and then quenched with water (1 mL). After extracting the aqueous layer with EtOAc (3 × 2 mL), the combined organic layer was washed with water, brine, dried over Na2SO4 and filtered. The solvent was removed under reduced pressure, and the residue was purified by silica gel flash column chromatography with EtOAc/hexanes (2:1) to give 1 (35 mg, 0.11 mmol, 91% yield) as a pale yellow needle. Rf = 0.13 (silica gel, EtOAc/hexanes 2:1); mp 192-194 °C (acetone/hexanes); IR (neat) 1673, 1511, 1208, 1108, 1071, 1034, 1013, 949, 859, 619 cm-1; 1H NMR (400 MHz, CDCl3) δ 7.75 (d, J = 10 Hz, 1H), 6.85 (s, 1H), 6.66 (s, 1H), 6.42 (d, J = 10 Hz, 1H), 6.37 (s, 1H), 4.22 (ddd, J = 13, 6.9, 5.0 Hz, 1H), 3.84 (s, 3H), 3.72 (s, 3H), 3.40 (ddd, J = 13, 8.7, 5.5 Hz, 1H), 3.27 (d, J = 16 Hz, 1H), 3.03 (ddd, J = 16, 8.7, 6.9 Hz, 1H), 2.83 (dt, J = 16, 5.5Hz, 1H), 2.80 (d, J = 16 Hz, 1H); 13C NMR (100 MHz, CDCl3) δ 195.3, 169.8, 154.9, 148.9, 147.4, 138.6, 132.0, 128.2, 126.1, 125.7, 112.6, 108.0, 67.8, 56.1, 56.0, 52.6, 37.0, 27.8; HRMS calcd for C18H17NO4Na [M + Na]+: 334.1056, found 334.1050.
ACKNOWLEDGEMENTS
This work was financially supported in part by the Grant-in-Aid for Scientific Research on Innovative Areas (2105) and for Scientific Research (B) (25293003) by MEXT, and a Waseda University Grant for Special Research Projects.
References
1. J. F. Grove, E. Reimann, and S. Roy, 'Progress in the Chemistry of Organic Natural Products: Synthesis Pathways to Erythrina Alkaloids and Erythrina Type Compounds,' Vol. 88, ed. by W. Herz, H. Falk, and G. W. Kirby, SpringerWienNewYork, 2007, pp. 1-212.
2. M. M. D. Mohammed, N. A. Ibrahim, N. E. Awad, A. A. Matloub, A. G. Mohamed-Ali, E. E. Barakat, A. E. Mohamed, and P. L. Cola, Nat. Prod. Res., 2012, 26, 1565. CrossRef
3. Y. Tsuda, S. Hosoi, N. Katagiri, C. Kaneko, and T. Sano, Chem. Pharm. Bull., 1993, 41, 2087. CrossRef
4. Reviews on hypervalent iodine chemistry: (a) P. J. Stang and V. V. Zhdankin, Chem. Rev., 1996, 96, 1123; CrossRef (b) T. Kitamura and Y. Fujiwara, Org. Prep. Proced. Int., 1997, 29, 409; CrossRef (c) A. Varvoglis, Tetrahedron, 1997, 53, 1179; (d) T. Wirth and U. H. Hirt, Synthesis, 1999, 1271; CrossRef (e) G. F. Koser, Aldrichimica Acta, 2001, 34, 89; (f) T. Wirth, Angew. Chem. Int. Ed., 2001, 40, 2812; CrossRef (g) V. V. Zhdankin and P. J. Stang, Chem. Rev., 2002, 102, 2523; CrossRef (h) P. J. Stang, J. Org. Chem., 2003, 68, 29973656; CrossRef (i) T. Wirth, Angew. Chem. Int. Ed., 2005, 44, 3656; CrossRef (j) R. M. Moriarty, J. Org. Chem., 2005, 70, 2893; CrossRef (k) V. V. Zhdankin and P. J. Stang, Chem. Rev., 2008, 108, 5299; CrossRef (l) E. A. Merritt and B. Olofsson, Angew. Chem. Int. Ed., 2009, 48, 9052; CrossRef (m) M. Uyanik and K. Ishihara, Chem. Commun., 2009, 2086. CrossRef
5. Oxidative dearomatization of p-hydroxyaniline derivative: (a) J. Liang, J. Chen, J. Liu, L. Li, and H. Zhang, Chem. Commun., 2010, 46, 3666; CrossRef (b) Z.-Q. Pan, J.-X. Liang, J.-B. Chen, X.-D. Yang, and H.-B. Zhang, Nat. Prod. Bioprospect., 2011, 1, 129. CrossRef
6. (a) A. S. Guram, R. A. Rennels, and S. L. Buchwald, Angew. Chem., Int. Ed. Engl., 1995, 34, 1348; CrossRef (b) J. Louie and J. F. Hartwig, Tetrahedron Lett., 1995, 36, 3609; CrossRef (c) J. P. Wolfe, S. Wagaw, and S. L. Buchwald, J. Am. Chem. Soc., 1996, 118, 7215. CrossRef
7. Y. Kita, T. Takada, M. Gyoten, H. Tohma, M. H. Zenk, and J. Eichhorn, J. Org. Chem., 1996, 61, 5857. CrossRef