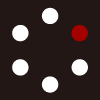
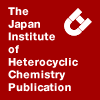
HETEROCYCLES
An International Journal for Reviews and Communications in Heterocyclic ChemistryWeb Edition ISSN: 1881-0942
Published online by The Japan Institute of Heterocyclic Chemistry
e-Journal
Full Text HTML
Received, 8th July, 2014, Accepted, 26th August, 2014, Published online, 3rd September, 2014.
DOI: 10.3987/COM-14-S(K)79
■ Simple, Selective, and Practical Synthesis of 2-Substituted 4(3H)-Quinazolinones by Yb(OTf)3-Catalyzed Condensation of 2-Aminobenzamide with Carboxamides
Tsutomu Yoshimura, Di Yuanjun, Yu Kimura, Hisatsugu Yamada, Akio Toshimitsu, and Teruyuki Kondo*
Advanced Biomedical Engineering Research Unit, Kyoto University, Katsura, Nishikyo-ku, Kyoto 615-8510, Japan
Abstract
A simple, selective, and practical synthetic method of 4(3H)-quinazolinones is realized by Yb(OTf)3-catalyzed condensation of 2-aminobenzamide with carboxamides. As the reaction proceeds, NH3 and H2O were formed as byproducts; however, Yb(OTf)3 can operate as an efficient Lewis acid catalyst without deactivation.4(3H)-Quinazolinones belong to one of the most important classes of fused heterocyclic compounds with a wide range of biological activities;1 e.g., protein tyrosine kinase inhibitor,2 cholecystokinin inhibitor,3 antimalarial,4 antibacterial,5 antifungal,6 antiviral,7 anti-HIV,8 anticancer,9 antiinflammatory,10 antiallergy,11 anticonvulsant,12 antihypertensive,13 and antidiabetic.14 In addition, the 4(3H)-quinazolinone nucleus is also the key component of chromophoric,15 thermochromic,16 and fluorescent materials.17
Typically, 4(3H)-quinazolinones were prepared on the basis of the Niementowski synthesis18 by condensation of anthranilic acid with carbonyl compounds and amines, proceeding via an 2-amidobenz- amide intermediate.19 Nevertheless, this method suffers from multi-step and tedious procedures, costly reagents, and often low yields. To overcome these problems, several new methods for synthesis of 4(3H)-quinazolinones have recently been developed, using acids and/or oxidants such as PFPAT,20 NaHSO3,21 DDQ/DMF,22 I2,23 TBAB,24 and using metal catalysts such as CuI,25 CuBr,26 PAPAT-CuCl2,27 FeCl3·6H2O,28 and Ga(OTf)329 [OTf = trifluoromethanesulfonate] as well as solid catalysts.30 Wang and coworkers have reported a pioneering study on Yb(OTf)3-catalyzed one-pot synthesis of 4(3H)-quinazolinones by condensation of anthranilic acid, anilines, and orthoesters (or formic acid, HCO2H) without solvents; however, only 3-substituted 4(3H)-quinazolinones were obtained by this reaction.31 In addition, Liu,32a Bakavoli,32b and Wang32c have independently developed the microwave-assisted Niementowski reaction, and palladium-catalyzed carbonylative synthesis of 4(3H)-quinazolinones has also been reported.33
We have developed a novel method for synthesis of symmetrically substituted ureas by ruthenium-catalyzed condensation reaction of 2 equivalents of aromatic amines with formamide.34 We have attempted to use this ruthenium-catalyzed reaction to construct N-heterocyclic compounds,35 and the reaction of 2-aminobenzamide 1a with formamide 2a was examined, which has two possibilities to give quinazoline-2,4(1H,3H)-dione (the upper reaction in Scheme 1) and to give 4(3H)-quinazolinone (3a, the lower reaction in Scheme 1). After many trials, we have found that this reaction offers a simple, selective, and practical method for catalytic synthesis of 4(3H)-quinazolinones.
First, the reaction of 2-aminobenzamide 1a with formamide 2a was carried out in the presence of a catalytic amount of RuCl2(PPh3)3 in mesitylene under reflux (bath temp. 165 oC) for 6 h to give 4(3H)-quinazolinone 3a in 24% yield (Table 1). The catalytic activities of several zero- and divalent ruthenium complexes were examined, and [(η6-C6H6)RuCl2]2 and RuCl3·3H2O bearing chloro-ligands showed the high catalytic activity, while the concomitant use of basic phosphine ligands, such as P(cyclo-C6H11)3 and PnBu3, drastically decreased the catalytic activity of RuCl3·3H2O.
Accordingly, we consider that ruthenium chlorides may work as a Lewis acid catalyst in the present reaction, and the catalytic activities of the representative Lewis acids were examined. As can be readily seen from Table 2, anhydrous AlCl3, FeCl3, and Yb(OTf)3 as well as NiBr2 hydrate showed the excellent catalytic activitiy to give 4(3H)-quinazolinone 3a in quantitative yield. However, YbCl3·6H2O and Yb(OAc)3·4H2O showed the moderate catalytic activity to give 3a in 66% and 35% yield, respectively. Naturally abundant FeCl3 and water-stable Yb(OTf)336 were the strong candidate of the catalyst for the present reaction; however, the catalytic activity of FeCl3 decreased, drastically, below 140 oC, while Yb(OTf)3 showed high catalytic activity above 100 oC.
Then, catalytic activities of several metal triflates were examined, and the results were summarized in Table 3. Among metal triflates examined, Yb(OTf)3 showed the highest catalytic activity, and 0.050 mmol (1.25 mol%) Yb(OTf)3 was at least required for the success of the present reaction. Mesitylene is the most suitable solvent for the present reaction, while the yield of 3a decreased, drastically, in diglyme (3a, 11%) and N-methylpiperidine (3a, 11%), due to their strong coordination ability through oxygen and nitrogen atoms to coordinatively unsaturated and catalytically active cationic ytterbium center (vide infra).
Formamide 2a is the most effective C1 source in the construction of 4(3H)-quinazolinone 3a, while methyl formate 2b or paraformaldehyde, (CH2O)n 2c, instead of formamide 2a, gave 3a in 39% and 12% yield, respectively, even under reflux in mesitylene (bath temp. 165 oC). Besides formamide 2a, carboxamides, such as acetamide 2d, 2-methylpropanamide 2e, pivalamide 2f, and benzamide 2g, can be used in the present reaction to give 2-methyl-, 2-isopropyl-, 2-(tert-butyl)-, and 2-phenyl-4(3H)-quinozolinone (3b -e) in good to high yields (Scheme 2).37
Condensation of several 2-aminobenzamides (1b-e) with formamide (2a) proceeded, smoothly, by Yb(OTf)3 catalyst to give the corresponding 4(3H)-quinazolinones (3f-i) in moderate to high isolated yields (Table 4).40 In addition, the related 2-aminonicotinamide (1f) also reacted with 2a under the present catalytic reaction conditions to give pyrido[2,3-d]pyrimidin-4(3H)-one (3j) in an isolated yield of 55%.
To investigate the mechanism, quinazoline- 2,4(1H,3H)-dione was treated with a catalytic amount of Yb(OTf)3 in mesitylene at 120 oC for 6 h under an argon atmosphere in the presence or absence of formamide 2a. In both reactions, no 3a was obtained at all (Scheme 3). Thus, the mechanism through the reduction of a carbonyl group in quinazolin-2,4(1H,3H)- dione was ruled out, completely.
Considering the results obtained above, the most plausible mechanism is illustrated in Scheme 4. We believe that the dissociation of a triflate anion (OTf-) from Yb(OTf)3 first occurred to generate catalytically active Yb(OTf)2+, which immediately coordinates to a carbonyl oxygen in carboxamide. Subsequent nucleophilic attack of an amino group in 2-aminobenzamide to the activated carbonyl carbon in carboxamide proceeded to give an amidine intermediate and Yb(OTf)2(OH), followed by dissociation of OH- to regenerate Yb(OTf)2+. Yb(OTf)2+ again coordinates to a carbonyl oxygen in benzamide to promote the intramolecular nucleophilic attack of an amino group to the activated carbonyl carbon. Isomerization of the intermediate, followed by elimination of NH3 give 4(3H)-quinazolinone and Yb(OTf)2+.
In conclusion, we developed one-pot and environmentally benign synthesis of 2-substituted 4(3H)-quinazolinones by Yb(OTf)3-catalyzed condensation of 2-aminobenzamides with carboxamides. Surprisingly, Yb(OTf)3 can operate as an efficient Lewis acid catalyst without deactivation even in the presence of byproducts, NH3 and H2O. Accordingly, application of Yb(OTf)3 catalyst to construct other valuable nitrogen-heterocycles is highly expected, and the study is in progress in our laboratory.
ACKNOWLEDGEMENTS
T.K. acknowledges financial support from the Asahi Glass Foundation and the Yazaki Memorial Foundation for Science and Technology. This research was conducted in part at Katsura-Int’tech Center, Graduate School of Engineering, Kyoto University.
References
1. For a review, see: (a) D. W. Fry, A. J. Kraker, A. McMichael, L. A. Ambroso, J. M. Nelson, W. R. Leopold, R. W. Connors, and A. J. Bridges, Science, 1994, 265, 1093; CrossRef (b) I. Hermecz, J. Kȍkȍsi, B. Podányi, and G. Szástz, Heterocycles, 1994, 37, 903; CrossRef (c) J. P. Michael, Nat. Prod. Rep., 2005, 22, 627; CrossRef (d) S. B. Mhaske and P. Argade, Tetrahedron, 2006, 62, 9787. CrossRef
2. G. Wagner and I. Wunderlich, Pharmazie, 1978, 33, 15.
3. (a) M. J. Yu, J. R. McCowan, N. R. Mason, J. B. Deeter, and L. G. Mendelsohn, J. Med. Chem., 1992, 35, 2534; (b) J. K. Padia, M. Field, J. Hinton, K. Meecham, J. Pablo, R. Pinnock, B. D. Roth, L. Singh, N. Suman-Chauhan, B. K. Trivedi, and L. Webdale, J. Med. Chem., 1998, 41, 1042; CrossRef (c) S. S. Ibrahim, A. M. Abdel-Halim, Y. Gabr, S. El-Edfawy, and R. M. Abdel-Rahman, J. Chem. Res. Synop., 1997, 154. CrossRef
4. Y. Takaya, H. Tasaka, T. Chiba, K. Uwai, M. A. Tanitsu, H. S. Kim, Y. Wataya, M. Miura, M. Takeshita, and Y. Oshima, J. Med. Chem., 1999, 42, 3163. CrossRef
5. P. P. Kung, M. D. Casper, K. L. Cook, L. Wilson-Lingard, L. M. Risen, A. Vickers, R. Ranken, L. B. Blyn, R. Wyatt, P. D. Cook, and J. Ecker, J. Med. Chem., 1999, 42, 4705. CrossRef
6. (a) H. Panwar, S. Singh, A. Kumar, S. Singh, N. Singh, and H. Singh, Der Pharma Chemica, 2011, 3, 399; (b) X. Wang, P. Li, Z. Li, J. Yin, M. He, W. Xue, Z. Chen, and B. Song, J. Agric. Food Chem., 2013, 61, 9575; (c) W. Pendergast, J. V. Johnson, S. H. Dickerson, I. K. Dey, D. S. Duch, R. Ferone, W. R. Hall, J. Humphery, J. Kelly, and D. C. Wilson, J. Med. Chem., 1993, 36, 2279; CrossRef (d) M. M. Ghorab, M. A. Abdel-Gawad, and M. S. A. El-Gaby, Farmaco, 2000, 55, 249 (CA, 2000, 133, 237956). CrossRef
7. X. Gao, X. Cai, K. Yan, B. Song, L. Gao, and Z. Chen, Molecules, 2007, 12, 2621. CrossRef
8. M. A. Khili, R. Soliman, A. M. Furghuli, and A. A. Bekhit, Arch. Pharm., 1994, 327, 27. CrossRef
9. (a) H.-Z. Li, H.-Y. He, Y.-Y. Han, X. Gu, L. He, Q.-R. Qi, Y.-L. Zhao, and L. Yang, Molecules, 2010, 15, 9473; CrossRef (b) A. Boumendjel, H. Baubichon-Cortay, D. Trompier, T. Perrotton, and A. D. Pietro, Med. Res. Rev., 2005, 25, 453. CrossRef
10. (a) V. Alagarsamy, S. V. Raja, and K. Dhanabal, Bioorg. Med. Chem., 2007, 15, 235; CrossRef (b) V. Alagarsamy, K. Dhanabai, P. Parthiban, G. Anjana, G. Deepa, B. Murugesan, S. Rajkumar, and A. J. Beevi, J. Pharm. Pharmacol., 2007, 59, 669. CrossRef
11. R. A. LeMahieu, M. Carson, W. C. Nason, D. R. Parrish, A. F. Weton, H. W. Baruth, and B. Yaremko, J. Med. Chem., 1983, 26, 420. CrossRef
12. V. Jatav, P. Mishra, S. Kashaw, and J. P. Stables, Eur. J. Med. Chem., 2008, 43, 135. CrossRef
13. (a) V. Alagarsamy, Pathak, and S. Urvishbhai, Bioorg. Med. Chem., 2007, 15, 3457; CrossRef (b) J.-W. Cherns P.-L. Tao, K.-C. Wang, A. A. Gutcait, S.-W. Liu, M.-H. Yen, S.-L. Chien, and J.-K. Rong, J. Med. Chem., 1998, 41, 3128.
14. M. S. Malamas and J. Millen, J. Med. Chem., 1991, 34, 1492. CrossRef
15. H. A. Bhatti and S. Seshadri, Color. Technol., 2004, 120, 10.
16. R. G. Patel, M. P. Patel, and R. G. Patel, Dyes and Pigments, 2005, 66, 7. CrossRef
17. S. P. Anthony, Chem. Asian J., 2012, 7, 374. CrossRef
18. S. Niementowski, Ber., 1894, 27, 1394. CrossRef
19. For a review, see: (a) D. J. Connolly, D. Cusack, T. P. O'Sullivan, and P. J. Guiry, Tetrahedron, 2005, 61, 10153; CrossRef (b) L. He, H. Li, J. Chen, and X.-F. Wu, RSC Adv., 2014, 4, 12065.
20. J. J. Naleway, C. M. J. Fox, D. Robinhold, E. Terpetsching, N. A. Olsen, and R. P. Haugland, Tetrahedron Lett., 1994, 35, 8569. CrossRef
21. S. E. Lopez, M. E. Rosales, N. Urdaneta, M. V. Godoy, and J. E. Charris, J. Chem. Res. Synop., 2000, 6, 258. CrossRef
22. (a) B. A. Bhat and D. P. Sahu, Synth. Commun., 2004, 34, 2169; CrossRef (b) M. Dabiri, P. Salehi, M. Bahramnejad, and M. Alizadeh, Monatsh. Chem., 2010, 141, 877. CrossRef
23. (a) M. Bakavoli, A. Shiri, Z. Ebrahimpour, and M. Rahimizadeh, Chin. Chem. Lett., 2008, 19, 1403; CrossRef (b) S.-L. Wang, K. Yang, C.-S. Yao, and X.-S. Wang, Synth. Commun., 2012, 42, 341. CrossRef
24. A. Davoodnia, S. Allameh, A. R. Fakhari, and N. Tavakoli-Hoseini, Chin. Chem. Lett., 2010, 21, 550. CrossRef
25. (a) J. Zhou, L. Fu, M. Lv, J. Liu, D. Pei, and K. Ding, Synthesis, 2008, 3974; CrossRef (b) X. Huang, H. Yang, H. Fu, R. Qiao, and Y. Zhao, Synthesis, 2009, 2679. CrossRef
26. W. Xu and H. Fu, J. Org. Chem., 2011, 76, 3846. CrossRef
27. N. Montazeri, K. Pourshamsian, S. Yosefiyan, and S. S. Momeni, J. Chem. Sci., 2012, 124, 883. CrossRef
28. G.-W. Wang, C.-B. Miao, and H. Kang, Bull. Chem. Soc. Jpn., 2006, 79, 1426. CrossRef
29. J. Chen, D. Wu, F. He, M. Liu, H. Wu, J. Ding, and W. Su, Tetrahedron Lett., 2008, 49, 3814. CrossRef
30. (a) M. Dabiri, P. Salehi, A. A. Mohammadi, M. Baghbanzadeh, and G. Kozehgiry, J. Chem. Res., 2004, 570; (b) M. M. Heravi, S. Sadjadi, S. Sadjadi, H. A. Oskooie, and F. F. Bamoharram, Ultrason. Sonochem., 2009, 16, 708; CrossRef (c) T.-H. Niloofar and D. Abolghasem, Chin. J. Chem., 2011, 29, 1685. CrossRef
31. L. Wang, J. Xia, F. Qin, C. Qian, and J. Sun, Synthesis, 2003, 1241. CrossRef
32. (a) J.-F. Liu, J. Lee, A. M. Dalton, G. Bi, L. Yu, C. M. Baldino, E. McElory, and M. Brown, Tetrahedron Lett., 2005, 46, 1241; CrossRef (b) M. Bakavoli, O. Sabzevari, and M. Rahimizadeh, Chin. Chem. Lett., 2007, 18, 1466; CrossRef (c) F. Li, Y. Feng, Q. Meng, W. Li, Z. Li, Q. Wang, and F. Tao, ARKIVOC, 2007, i, 40. CrossRef
33. (a) C. Larksarp and H. Alper, J. Org. Chem., 2000, 65, 2773; CrossRef (b) L. He, H. Li, H. Neumann, M. Beller, and X.-F. Wu, Angew. Chem. Int. Ed., 2014, 53, 1420; CrossRef (c) X. Jiang, T. Tang, J.-M. Wang, Z. Chen, Y.-M. Zhu, and S.-J. Ji, J. Org. Chem., 2014, 79, 5082. CrossRef
34. T. Kondo, S. Kotachi, Y. Tsuji, Y. Watanabe, and T. Mitsudo, Organometallics, 1997, 16, 2562. CrossRef
35. (a) M. Akazome, T. Kondo, and Y. Watanabe, J. Org. Chem., 1993, 58, 310; CrossRef (b) T. Kondo, T. Kanda, D. Takagi, K. Wada, Y. Kimura, and A. Toshimitsu, Heterocycles, 2012, 86, 1015. CrossRef
36. For a review, see: (a) S. Kobayashi, Synlett, 1994, 689; (b) T. Kitanosonoa and S. Kobayashi, Adv. Synth. Catal., 2013, 355, 3095. CrossRef
37. A mixture of 2-aminobenzamide (1, 4.0 mmol), carboxamide (2, 6.0 mmol), Yb(OTf)3 (0.20 mmol, 5.0 mol%), and mesitylene (5.0 mL) was placed in a 20-mL Pyrex flask equipped with a magnetic stirring bar and a reflux condenser under a flow of argon. The reaction was carried out at 120-165 oC (bath temp.) for 6 h with stirring. Then, the reaction mixture was cooled to room temperature, and analyzed by GLC, GC-MS (EI), and LC-MS (ESI). After evaporation of mesitylene under vacuum, the products (3) were isolated by recrystallization from MeOH/hexane and/or medium pressure column chromatography on silica gel (eluent: EtOAc/hexane = 50/50 ~ EtOAc 100%. For 3j, eluent: MeOH/CHCl3 = 50/50). 1H NMR spectra were recorded at 400 MHz, and 13C NMR spectra were recorded at 100 MHz in DMSO-d6. The analytical and spectral data of 3a-e,38 3f,39 3g,40 3h,41 and 3j,42 were consistent with those reported previously. The product, 3i, was characterized below43.
38. D. J. Connolly, P. M. Lacey, M. McCarthy, C. P. Saunders, A.-M. Carroll, R. Goddard, and P. J. Guiry, J. Org. Chem., 2004, 69, 6572. CrossRef
39. S. K. Kundu, M. P. D. Mahindaratne, M. V. Quintero, A. Bao, and G. R. Negrete, ARKIVOC, 2008, ii, 33.
40. A. A. Layeva, E. V. Nosova, G. N. Lipunova, T. V. Trashakhova, and V. N. Charushin, Russ. Chem. Bull., Int. Ed., 2007, 56, 1821.
41. L. Ȍrfi, F. Wáczek, J. Pató, I. Varga, B. Hegymegi-Barakonyi, R. A. Houghten, and G. Kéri, Curr. Med. Chem., 2004, 11, 2549.
42. A. Srinivasan, P. E. Fagerness, and A. D. Broom, J. Org. Chem., 1978, 43, 828. CrossRef
43. 3i: a white solid. IR (KBr) cm-1: 3127, 1698; 1H NMR (DMSO-d6, 400 MHz) δ: 12.16 (1H, br s), 8.00 (1H, s), 7.61 (1H, d, J = 8.98 Hz), 7.49 (1H, d, J = 2.86 Hz), 7.42 (1H, dd, J = 8.98 and 2.86 Hz), 3.86 (3H, s); 13C NMR (DMSO-d6, 100 MHz) δ: 160.4, 157.7, 143.2, 142.8, 128.7, 123.7, 123.4, 105.8, 55.6; MS (ESI): 177.0726 (M+H)+.