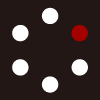
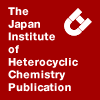
HETEROCYCLES
An International Journal for Reviews and Communications in Heterocyclic ChemistryWeb Edition ISSN: 1881-0942
Published online by The Japan Institute of Heterocyclic Chemistry
e-Journal
Full Text HTML
Received, 26th June, 2014, Accepted, 29th July, 2014, Published online, 4th August, 2014.
DOI: 10.3987/COM-14-S(K)42
■ Total Synthesis of Marine Sesquiterpenoid Sinularianin B and 8-epi-Sinularianin B
Koichiro Ota and Hiroaki Miyaoka*
School of Pharmacy, Tokyo University of Pharmacy and Life Sciences, 1432-1 Horinouchi, Hachioji, Tokyo 192-0392, Japan
Abstract
The enantioselective total synthesis of marine sesquiterpenoid sinularianin B, isolated from the Formosan soft coral Sinularia sp., has been accomplished in 16 steps based on a highly concise synthetic strategy. The synthesis features two highly stereoselective sulfone-mediated reactions, including a key tandem intermolecular−intramolecular alkylation developed in our laboratory, and a palladium-catalyzed desulfonylation of an allyl sulfone through π-allyl palladium complex formation.INTRODUCTION
Sinularianin B (1) is a structurally unique valerenane sesquiterpenoid first isolated in 2006 from extracts of the Formosan soft coral Sinularia sp. by Sheu and co-workers (Figure 1).1 The structure of this unique tricyclic sesquiterpenoid was determined by detailed NMR spectroscopic analysis to comprise a highly strained trans-5,6-fused ring appended to γ-spirolactone. Although the overall relative configuration of sinularianin B was assigned on the basis of spectral analysis, the absolute configuration had not been determined. Furthermore, sesquiterpenoids possessing a valerenane skeleton have been isolated mostly from terrestrial plants, e.g. Valeriana officinalis,2 although one report has described the isolation of valerenane sesquiterpenoid from soft coral.3 Recently, we were first to report on the asymmetric total synthesis of sinularianin B (1) using our developed method involving sulfone-mediated highly stereoselective tandem intermolecular−intramolecular alkylation. 4 In this paper, we wish to report on the detailed synthesis of sinularianin B and 8-epi-sinularianin B.
Our initial retrosynthetic analysis for the synthesis of sinularianin B (1) is outlined in Figure 2. We envisioned that the trans-5,6-fused ring moiety should be constructed in the latter stages due to potential isomerization of the double bond between C-5 and C-6 under both acidic and basic conditions. Therefore, we set triene A for further elaboration toward 1. Triene A was expected to be generated from aldehyde B through stepwise double alkylation at C-8. Aldehyde B could be generated from sulfonylcyclopentane C by stereoselective palladium-mediated hydrogenolysis and oxidation. Key intermediate sulfonylcyclopentane C would be constructed by our developed tandem intermolecular−intramolecular alkylation of epoxide D and methallyl phenyl sulfone.5 Optically active epoxide D could be derived from known allylic alcohol 2.6
RESULTS AND DISCUSSION
The first stage involved construction of the sulfonylcyclopentane possessing a tertiary hydroxy group at C-1 (sinularianin B numbering). The asymmetric synthesis of sulfonylcyclopentane 5 from epoxyiodide 4 and methallyl phenyl sulfone-mediated tandem intermolecular−intramolecular alkylation is shown in Scheme 1. According to the known three-step procedure, 4-hydroxybutan-2-one was elaborated to allylic alcohol 2,6 which was then converted to epoxyalcohol 3 in 97% yield using Sharpless asymmetric epoxidation under standard conditions.7 The optical purity of epoxyalcohol 3 was determined as >95% ee, which was estimated from the 1H NMR spectra in the presence of chiral shift reagent “Chirabite-AR”.8 To demonstrate the practical utility of Chirabite-AR, at first, synthetic racemic epoxyalcohol rac-3 was tested. Prior to the comparison between synthetic (−)-epoxyalcohol 3 and rac-3 with Chirabite-AR, we examined the effect of differing amounts of Chirabite-AR regarding rac-3, in an effort to determine sufficient signal separations between (+)- and (−)-3. Consequently, a mixture of rac-3 with 75 mol% of Chirabite-AR was measured sequentially by 400 MHz 1H NMR at 25 °C in CDCl3. The methine proton at C-9 signal separations were observed between 3.32 to 3.14 ppm, and good enantiomeric discrimination was achieved for (+)- and (−)-3. NMR analysis of (−)-epoxyalcohol 3 under the same conditions indicated that separated signals exhibited >39/1 ratio in numerical integration value. Therefore, the optical purity of (−)-3 was determined as >95 % ee. Epoxyiodide 4, required for the key tandem intermolecular−intramolecular alkylation necessary for the construction of sulfonylcyclopentane 5, was obtained by protection of the primary hydroxy group in chiral epoxyalcohol 3 with benzyl bromide, sodium hydride, and TBAI, selective deprotection with DDQ, and iodination of the primary hydroxy group with I2, Ph3P and imidazole in 91% yield for the three steps and in multigram quantities. The sulfonylcarbanion prepared from methallyl phenyl sulfone (1.30 equiv.) and nBuLi (1.25 equiv.) was treated with epoxyiodide 4 at −45 °C. Following confirmation of the disappearance of the starting material by TLC, nBuLi (2.00 equiv.) and Me3Al (1.50 equiv.) were added sequentially at −78 °C, and allowing the reaction mixture to warm to −55 °C cleanly promoted 5-endo cyclization to give sulfonylcyclopentane 5 as the sole product in 99% yield.
The relative configuration of sulfonylcyclopentane 5 was determined by NOESY analysis (Figure 3). The trans-configuration of the isopropenyl and benzyloxymethyl groups in sulfonylcyclopentane 5 was determined by NOE correlation between the β-methylene proton at C-3 and the sp2-methine proton at the ortho position of the phenylsulfonyl group, the α-methylene proton at C-3 and the methine proton at C-9, the methylene protons at C-8 and the methyl protons at C-10, and the methine proton at C-9 and the methyl protons at C-11.
The complete diastereoselectivity associated with the tandem intermolecular−intramolecular alkylation was attributed to the stability of the transition state in the intramolecular alkylation from the sulfonylcarbanion intermediate. The carbanion of methallyl phenyl sulfone reacted with epoxyiodide 4 to form epoxysulfone 6, which is rapidly converted into corresponding sulfonylcarbanion 6a. Furthermore, activation of the epoxide that followed the addition of Me3Al as a Lewis acid, shifted the equilibrium between sulfonylcarbanions 6b and 6c. In the equilibrium state, intramolecular cyclization mostly proceeded from sulfonylcarbanion 6c, as a result of transition state destabilization due to steric hindrance between the phenylsulfonyl group and the benzyloxymethyl group in sulfonylcarbanion 6b. Consequently, sulfonylcyclopentane 5 was generated by acidic workup of alkoxyaluminum anionic species 6d.
We next focused on removal of the phenylsulfonyl group in sulfonylcyclopentane 5. We conceived a hydrogenolysis approach via a π-allyl palladium complex (Table 1). Initially, we applied NaBH49 or LiBHEt310 as a standard hydride source for the palladium-mediated hydrogenolysis, however, the desired cyclopentane 7 was not generated, and isomerization proceeded to the corresponding internal olefin 9 (entries 1 and 2). A solution to this problem was found by using in situ-generated ammonium formate as the hydride source,11 where treatment of sulfonylcyclopentane 5 with triethylamine (8.0 equiv.), formic acid (8.0 equiv.), and PdCl2(dppp) (0.005 equiv.) in THF at rt facilitated regioselective hydrogenolysis and provided cyclopentane 7 in 16% yield and 4-epimer 8 in 18% yield with low diastereoselectivity (entry 3). After some optimization, optimal reaction conditions included a reagent combination comprising Pd2(dba)3·CHCl3 (0.08 equiv.), nBu3P (0.20 equiv.), triethylamine (8.0 equiv.), and formic acid (8.0 equiv.) in 1,4-dioxane (0.05 M) under reflux, and these conditions generated cyclopentane 7 in 91% yield with high stereoselectivity at C-4 (>20 : 1 d.r.) (entry 8).
The relative configurations of cyclopentane 7 were determined by NOE correlation between the methine proton at C-4 and the methyl protons at C-10, the methylene protons at C-8 and the methyl protons at C-10, and the methine proton at C-9 and the methyl protons at C-11 (Figure 5). Similarly, the relative configurations of 4-epimer 8 were determined by NOE correlation between the methine proton at C-4 and the methine proton at C-9, the methylene protons at C-8 and the methyl protons at C-10, and the methylene protons at C-8 and the methyl protons at C-11.
In order to account for the observed improvement in the reaction outcome, a possible mechanism of the regio- and stereoselective hydrogenolysis has been outlined in Figure 6. In palladium-catalyzed hydrogenolysis, it is well-established that the initial step in π-allyl palladium complex formation involves inversion of stereochemistry. The resulting π-allyl palladium complex 10a rapidly rearranges to the σ-allyl palladium formate 10b, and subsequently the single bond rotation, which was caused by the non-bonding interactions between the oxygen atom and the cationic palladium species, generated 10c. Subsequent migration of the hydride from the σ-allyl palladium formate on the more substituted end of the allylic system afforded cyclopentane 7.
As shown in Scheme 2, removal of the benzyl group in cyclopentane 7 with Na/liq.NH3 did not affect the terminal olefin and proceeded smoothly to give the primary hydroxy group. Subsequent treatment of the derived diol with IBX12 in DMSO/THF oxidized the primary alcohol to corresponding aldehyde 11 in 96% yield for the two steps. Next, according to the synthetic plan, we introduced two different allylic groups at C-8. Thus, zinc-mediated Barbier−Grignard type allylation13 of aldehyde 11 with 2-(bromomethyl)allyl acetate in saturated NH4Cl aqueous/THF furnished corresponding diol 12 as diastereomeric mixtures. Oxidation of diol 12 with IBX in DMSO/THF afforded the β,γ-unsaturated ketone in 87% yield for the two steps. To the resulting ketone was introduced an allyl group using allyl magnesium bromide, giving triene 13 in 85% yield as a single diastereomer. Although it was not possible to determine the configuration of the tertiary hydroxy group at C-8 in triene 13 at this stage, the presence of an opposite configuration to that of the natural sinularianin B was determined by NOESY analysis at a later step. Oxidation of the allylic hydroxy group in stereochemically-undefined triene 13 with manganese dioxide14 led to α-methylene-γ-lactone 14 in quantitative yield. Isomerization with rhodium chloride trihydrate,15 and ring-closing metathesis (RCM) of the resulting α,β-unsaturated lactone gave tricyclic compound 15 in 30% yield for the two steps.
Since the 1H- and 13C NMR data of synthetic 15 and the natural product did not match,1 the aforementioned determination of the relative configuration at C-8 was performed at this stage as shown in Figure 7. The relative configurations of 15 were determined by NOE correlation between the methine proton at C-4 and the methyl protons at C-10, the methine proton at C-4 and the olefin proton at C-12, the β-methylene proton at C-7 and the olefin proton at C-12, the α-methylene proton at C-7 and the methine proton at C-9, and the methyl protons at C-10 and the olefin proton at C-12. Therefore, synthetic compound 15 was determined to be 8-epi-sinularianin B.
Given the above results, a second synthetic attempt was performed whereby the order of introducing the side chain was altered. Zinc-mediated Barbier−Grignard type allylation13 of aldehyde 11 with 3-bromoprop-1-ene in saturated NH4Cl aqueous/THF furnished the corresponding diol as diastereomeric mixtures (Scheme 3). Subsequent treatment of the derived diol with IBX12 in DMSO/THF oxidized the secondary alcohol to β,γ-unsaturated ketone 16 in 92% yield for the two steps. Although metal-mediated allylation of β,γ-unsaturated ketone 16 with various hydroxy group protected allylic bromides (R = Bn, MPM, THP, TBS) was negligible, β,γ-unsaturated ketone 16 underwent decomposition.
On the basis of this unsatisfactory result, an alternative strategy had to be implemented (Figure 8). We envisaged that the γ-spirolactone moiety of sinularianin B (1) could be assembled from precursor E. Construction of the cyclohexene ring of E from the O-silylated cyanohydrin F would involve sequential RCM, conversion of the nitrile to the aldehyde, and deprotection. O-Silylated cyanohydrin F could be generated from aldehyde 11 by cyanohydrin formation and stereoselective alkylation with allyl halide at C-8.
As shown in Scheme 4, for the pivotal construction of the trans-5,6-fused ring, aldehyde 11 was treated with TBSCN in the presence of a catalytic amount of bis(triphenylphosphoranylidene)ammonium chloride (PNPCl).16 Following confirmation of the disappearance of 11 by TLC, TMSCN was added, which promoted silylation of the bulky tertiary hydroxy group at C-1 to afford α-siloxynitrile 18 as the diastereomeric mixture at C-8. Initially, allylation of crude α-siloxynitrile 18 with allyl bromide was negligible given the steric bulkiness of the nucleophile. However, following extensive optimization, it was found that generation of the carbanion of α-siloxynitrile 18 with LDA in THF at 0 °C followed by cooling to −78 °C and addition of allyl bromide premixed with HMPA gave α-siloxynitrile 18 as an inseparable diastereomeric mixture at C-8 and acceptable selectivity (5 : 1). After considerable optimization, RCM of a mixture comprising α-siloxynitrile 18 and C-8 epimer cleanly generated the trans-5,6-fused ring using Grubbs 2nd generation catalyst,17 with high catalyst loading (10 mol%). Reduction of the cyano group with DIBAH led to the α-siloxyaldehyde. The reaction proceeded in good yields, based on careful monitoring of the weak acidic workup conditions and a minimal excess of DIBAH. Interestingly, degradation of the C-8 epimer occurred concurrently with DIBAH reduction. Deprotection with TBAF/AcOH afforded α-siloxyaldehyde 20 as a sole product in 67% yield for the five steps from 11. The desired R configuration at C-8 of α-siloxyaldehyde 20 was determined by single-crystal X-ray diffraction (Figure 9).
The final critical step, involving assembly of sinularianin B (1), was executed via the α,β-unsaturated seven-membered lactone. α-Siloxyaldehyde 20 was coupled with 2-(diethoxyphosphoryl)propanoic acid using carbodiimide18 to give the phosphate as shown in Scheme 5. The resultant phosphate was utilized in an intramolecular Horner-Wadsworth-Emmons reaction19 to give α,β-unsaturated seven-membered lactone 21 in 78% yield for the two steps from 20. The last transformation was best achieved by one-pot desilylation/methanolysis/re-lactonization methodologies, and where it was imperative to remove the TBS group from lactone 21 with TBAF to generate γ-hydroxylactone. Following confirmation of the disappearance of lactone 21 by TLC, treatment with K2CO3 in MeOH at 40 °C for 2 days cleanly promoted methanolysis and rapid re-lactonization to give sinularianin B (1) as the sole product in 99% yield. The 1H and 13C NMR spectroscopic data of synthetic 1 matched those reported for sinularianin B. Comparison of the specific optical rotation data {[α]D −111 (c 1.55, CHCl3); lit.1 [α]D −27 (c 1.04, CHCl3)} enabled us to establish the absolute configuration of natural sinularianin B as being identical to that of synthetic product 1. Despite the discrepancy in magnitude, the match of all other data has confirmed that the constitution and stereochemistry of sinularianin B (1) assigned by previously determined spectroscopic analyses are correct.
In conclusion, a highly stereoselective total synthesis of sinularianin B (1) was accomplished by a concise strategy. The procedure comprises 16 steps (longest linear sequence) with 39.5% yield starting from known allylic alcohol 2. Furthermore, this work has unambiguously established the absolute configuration. Our synthetic strategy can be highlighted by the use of sulfone-mediated tandem intermolecular−intramolecular alkylation for construction of the cyclopentane core, palladium-catalyzed hydrogenolysis of allyl sulfone through π-allyl palladium complex formation, ring-closing metathesis to build the highly strained trans-5,6-fused ring, and an intramolecular Horner-Wadsworth-Emmons reaction for conversion of the seven-membered lactone into γ-spirolactone by an apparent acyl rearrangement.
EXPERIMENTAL
All reagents (Aldrich, Kanto, TCI and Wako) and solvents were of commercial quality and were used as received. Reactions were monitored by thin layer chromatography on glass plates coated with a fluorescent indicator with a 254 nm excitation wavelength (Merck Merck-5554-7). Flash column chromatography was performed using Kanto Chemical Silica Gel 60N (spherical, natural) 40−50 µm. Melting points (mp) were measured using the Yanaco melting point apparatus MP-S3 and are uncorrected. Optical rotations were measured with a JASCO P-1030 polarimeter. IR spectra were recorded with a JASCO FT-IR/620 spectrometer. UV spectra were recorded using a SHIMADZU UV-1200 spectrophotometer. Single crystal X-ray diffraction was recorded using a MacScience Co., Ltd DIP 2020 Image Plate. 1H- and 13C NMR spectra were recorded on a Bruker AVANCE III HD Nanobay or Bruker Biospin AV-600 spectrometer. Chemical shifts are given on the δ (ppm) scale using tetramethylsilane (TMS) as the internal standard (s, singlet; d, doublet; t, triplet; q, quartet; quint., quintet; m, multiplet; br, broad). High resolution ESIMS (HRESIMS) spectra were obtained using a Micromass LCT spectrometer. Elemental analysis data were obtained using an Elementar Vario EL.
((2R,3R)-3-(2-(4-Methoxybenzyloxy)ethyl)-3-methyloxiran-2-yl)methanol (3): To a cold (−20 °C) suspension of 4Å molecular sieves (8.44 g) in CH2Cl2 (38.0 mL) were added D-(−)-DIPT (1.50 mL, 7.13 mmol), Ti(OiPr)4 (1.10 mL, 3.73 mmol) and TBHP (5.55 M solution in CH2Cl2, 38.6 mL, 214 mmol). After stirring for 30 min at the same temperature, a solution of allylic alcohol 2 (16.9 g, 71.5 mmol) in CH2Cl2 (200 mL) was added over 10 h. After stirring at −20 °C for 2 h, NaOH (30% solution in brine, 3.25 mL) was added. The mixture was diluted with Et2O, warmed to room temperature, and stirred for 10 min. MgSO4 (2.90 g) and Celite (0.35 g) were then added, and after stirring for 15 min the mixture was passed through a pad of Celite and then concentrated in vacuo. The residue was purified with flash column chromatography on silica gel (hexane/AcOEt = 1:1) to give epoxyalcohol 3 (17.5 g, 97% yield) as a colorless oil. Rf = 0.20 (hexane/AcOEt 1:1); [α]D25 +0.29 (c 1.06 in CHCl3); 1H NMR (400 MHz, CDCl3): δ = 7.24 (d, J = 8.6 Hz, 2H), 6.87 (d, J = 8.6 Hz, 2H), 4.43 (d, J = 14.7 Hz, 1H), 4.40 (d, J = 14.7 Hz, 1H), 3.80 (m, 1H), 3.79 (s, 3H), 3.65 (m, 1H), 3.57−3.51 (m, 2H), 3.02 (dd, J = 4.4, 6.5 Hz, 1H), 2.15 (brs, 1H), 1.94 (ddd, J = 5.9, 6.0, 14.3 Hz, 1H), 1.78 (ddd, J = 6.6, 7.1, 14.3 Hz, 1H), 1.30 (s, 3H); 13C NMR (100 MHz, CDCl3): δ = 159.1 (s), 130.2 (s), 129.2 (d)×2, 113.8 (d)×2, 72.6 (t), 66.0 (t), 62.8 (d), 61.2 (t), 59.6 (s), 55.2 (q), 38.2 (t), 17.2 (q); IR (neat): ν∼ = 3441, 2932 cm−1; HRMS (ESI): m/z calcd for C14H20O4+Na+: 275.1259 [M+Na+]; found: 275.1247; Anal. Calcd for C14H20O4: C 66.65, H 7.99; found: C 66.64, H 8.14.
Determination of optical purity of synthetic (−)-epoxyalcohol 3: Before comparison between synthetic (−)-epoxyalcohol 3 and synthetic racemic epoxyalcohol rac-3 with Chirabite-AR, we examined the effect of differing amounts of Chirabite-AR regarding rac-3, to determine sufficient signal separations between (+)- and (−)-3. Consequently, a mixture of rac-3 with 75 mol% of Chirabite-AR was measured sequentially by 400 MHz 1H NMR at 25 °C in CDCl3, the methine proton at C-9 signal separations were observed between 3.32 to 3.14 ppm, and good enantiomeric discrimination was achieved for (+)- and (−)-3. NMR analysis of (−)-epoxyalcohol 3 under the same conditions as used to obtain the results indicated that separated signals exhibited >39/1 ratio in numerical integration value. Therefore, the optical purity of synthetic (−)-3 was determined as >95 %ee.
(2R,3R)-3-Benzyloxymethyl-2-(2-iodoethyl)-2-methyloxirane (4): To a stirring solution of epoxyalcohol 3 (16.5 g, 65.4 mmol) in THF (109 mL) were added NaH (55%, 5.70 g, 432 mmol), BnBr (11.7 mL, 98.5 mmol) and TBAI (2.40 g, 6.50 mmol) at 0 °C and then allowed to warm to room temperature. After stirring for 7 h, MeOH (10.0 mL) was slowly added at 0 °C. The mixture was then allowed to warm to room temperature. After stirring for 1 h, the mixture was diluted with Et2O, washed with saturated aqueous NaHCO3 solution, H2O and brine, and then concentrated in vacuo. The residue was passed through a pad of silica gel (hexane/AcOEt = 4:1) and then concentrated in vacuo to give a crude product.
To a stirring suspension of the crude product in CH2Cl2/saturated aqueous NaHCO3 solution (10:1, 127 mL) were added DDQ (22.3 g, 98.2 mmol) over 10 min at room temperature. After stirring for 30 min, the reaction mixture was diluted with Et2O, washed with saturated aqueous NH4Cl solution, H2O and brine, dried over anhydrous Na2SO4, and then concentrated in vacuo. The residue was passed through a pad of silica gel (hexane/AcOEt = 2:1) and then concentrated in vacuo to give a crude product.
To a cold (0 °C) solution of the crude product in CH2Cl2 (211 mL) were added Ph3P (19.9 g, 75.9 mmol), imidazole (6.47 g, 95.0 mmol). After stirring for 5 min at the same temperature, I2 (19.4 g, 76.4 mmol) was slowly added. The mixture was then allowed to warm to room temperature. After stirring for 10 min, the solvent was removed in vacuo. The residue was passed through a pad of silica gel (hexane/Et2O = 6:1) and then concentrated in vacuo. The residue was purified with flash column chromatography on silica gel (hexane/AcOEt = 9:1) to give epoxyiodide 4 (19.8 g, 91% yield for 3 steps) as a colorless oil. Rf = 0.60 (hexane/AcOEt 2:1); [α]D25 +7.19 (c 1.53 in CHCl3); 1H NMR (400 MHz, CDCl3): δ = 7.37-7.30 (m, 5H), 4.64 (d, J = 11.9 Hz, 1H), 4.55 (d, J = 11.9 Hz, 1H), 3.75 (dd, J = 4.2, 11.3 Hz, 1H), 3.55 (dd, J = 6.2, 11.3 Hz, 1H), 3.20 (ddd, J = 5.3, 8.6, 9.2 Hz, 1H), 3.15 (ddd, J = 1.9, 7.7, 8.6 Hz, 1H), 3.10 (dd, J = 4.2, 6.2 Hz, 1H), 2.24 (ddd, J = 5.3, 8.6, 14.3 Hz, 1H), 2.04 (ddd, J = 7.7, 9.2, 14.3 Hz, 1H), 1.26 (s, 3H); 13C NMR (100 MHz, CDCl3): δ = 137.8 (s), 128.4 (d)×2, 127.7 (d), 127.7 (d)×2, 73.2 (t), 68.5 (t), 61.1 (d), 60.1 (s), 42.2 (t), 16.2 (q), −1.2 (t); IR (neat): ν∼ = 2925, 2857 cm−1; HRMS (ESI): m/z calcd for C13H17IO2+H+: 333.0352 [M+H+]; found: 333.0335; Anal. Calcd for C13H17IO2: C 47.00, H 5.16; found: C 46.94, H 5.27.
(1R,2S,3R)-2-Benzyloxymethyl-1-methyl-3-phenylperoxythio-3-prop-1-en-2-ylcyclopentanol (5): To a solution of methallyl phenyl sulfone (1.45 g, 7.39 mmol) in THF (24.0 mL) were added nBuLi (1.58 M solution in hexane, 4.50 mL, 7.11 mmol) at −78 °C. After stirring for 30 min at the same temperature, a solution of epoxyiodide 4 (1.89 g, 5.69 mmol) in THF (90.0 mL) was added and then allowed to warm to −45 °C over 18 h. After cooling to −78 °C, nBuLi (1.58 M solution in hexane, 7.20 mL, 11.4 mmol). After stirring for 15 min at the same temperature, Me3Al (1.07 M solution in hexane, 8.00 mL, 8.56 mmol) was introduced and then allowed to warm to −55 °C. After stirring for 2.5 h, the reaction mixture was diluted with Et2O, washed with saturated aqueous NH4Cl solution, 1.0 M aqueous HCl solution, H2O and brine, dried over anhydrous Na2SO4, and then concentrated in vacuo. The residue was purified with flash column chromatography on silica gel (hexane/AcOEt = 2:1) to give cyclopentane 5 (2.26 g, 99% yield) as a white needle-like crystalline solid. Rf = 0.15 (hexane/AcOEt 2:1); mp 108−109 °C (recrystallized from hexane/AcOEt); [α]D25 −45.7 (c 1.16 in CHCl3); 1H NMR (400 MHz, CDCl3): δ = 7.73−7.72 (m, 2H), 7.59 (m, 1H), 7.47−7.44 (m, 2H), 7.36−7.29 (m, 5H), 4.99 (s, 1H), 4.66 (d, J = 11.6 Hz, 1H), 4.61 (s, 1H), 4.59 (d, J = 11.6 Hz, 1H), 4.46 (dd, J = 4.8, 9.5 Hz, 1H), 4.41 (dd, J = 9.5, 11.4 Hz, 1H), 3.07 (brs, 1H), 2.75 (dd, J = 4.8, 11.4 Hz, 1H), 2.41 (dt, J = 6.4, 12.9 Hz, 1H), 1.98 (s, 3H), 1.92−1.81 (m, 2H), 1.71 (dt, J = 6.4, 12.9 Hz, 1H), 1.60 (s, 3H); NOE correlations (H/H): H-3 (δH 2.41)/ortho-H (δH 7.73), H-3 (δH 2.41)/H-10 (δH 1.60), H-3 (δH 1.71)/H-9 (δH 2.75), H-8 (δH 4.46)/H-10 (δH 1.60), H-8 (δH 4.41)/H-11 (δH 1.98), H-9 (δH 2.75)/H-11 (δH 1.98); 13C NMR (100 MHz, CDCl3): δ = 142.2 (s), 137.8 (s), 136.0 (s), 133.5 (d), 130.3 (d)×2, 128.4 (d)×2, 128.0 (d)×2, 127.7 (d), 127.7 (d)×2, 118.1 (t), 80.4 (s), 77.5 (s), 73.6 (t), 68.8 (t), 57.9 (d), 38.0 (t), 31.4 (t), 22.9 (q), 20.8 (q); IR (KBr): ν∼ = 3363, 2975, 2931, 1291, 1135 cm−1; HRMS (ESI): m/z calcd for C23H28O4S+Na+: 423.1606 [M+Na+]; found: 423.1591; Anal. Calcd for C23H27O4S: C 68.97, H 7.05; found: C 69.17, H 7.00.
(1R,2S,3S)-2-(Benzyloxymethyl)-1-methyl-3-(prop-1-en-2-yl)cyclopentanol (7) and (1R,2S,3R)-2- (benzyloxymethyl)-1-methyl-3-(prop-1-en-2-yl)cyclopentanol (8): nBu3P (0.82 mL, 3.28 mmol) was added to a solution of Pd2(dba)3·CHCl3 (1.36 g, 1.31 mmol) in 1,4-dioxane (200 mL) at room temperature and the mixture was stirred for 10 min. Et3N (18.3 mL, 131 mmol) and HCO2H (4.95 mL, 131 mmol) were added to the mixture at the same temperature. After stirring for 10 min, the mixture was refluxed. A solution of cyclopentane 5 (6.56 g, 16.4 mmol) in 1,4-dioxane (128 mL) was added to the mixture and the mixture was stirred for 15 min. The reaction mixture was concentrated in vacuo. The residue was purified with flash column chromatography on silica gel (hexane/AcOEt = 5:1) to give trans-cyclopentane 7 (3.89 g, 91% yield) as a colorless oil and cis-cyclopentane 8 (171 mg, 4% yield) as a colorless oil. trans-cyclopentane 7: Rf = 0.40 (hexane/AcOEt 3:1); [α]D25 +31.9 (c 1.23 in CHCl3); 1H NMR (400 MHz, CDCl3): δ = 7.37−7.29 (m, 5H), 4.68 (s, 1H), 4.68 (s, 1H), 4.52 (d, J = 11.8 Hz, 1H), 4.45 (d, J = 11.8 Hz, 1H), 3.55 (dd, J = 4.0, 9.4 Hz, 1H), 3.43 (t, J = 9.4 Hz, 1H), 2.85 (brs, 1H), 2.28−2.16 (m, 2H), 1.87−1.59 (m, 4H), 1.72 (s, 3H), 1.24 (s, 3H); 1H NMR (400 MHz, C6D6): δ = 7.31−7.16 (m, 5H), 4.84 (s, 1H), 4.81 (s, 1H), 4.33 (d, J = 11.9 Hz, 1H), 4.26 (d, J = 11.9 Hz, 1H), 3.55 (dd, J = 4.4, 9.4 Hz, 1H), 3.38 (t, J = 9.4 Hz, 1H), 2.72 (brs, 1H), 2.40 (dt, J = 4.4, 10.7 Hz, 1H), 2.24 (dt, J = 10.7, 8.7 Hz, 1H), 2.05 (dt, J = 11.0, 7.4 Hz, 1H), 1.78−1.64 (m, 3H), 1.71 (s, 3H), 1.33 (s, 3H); NOE correlations (H/H): H-4 (δH 2.24)/H-10 (δH 1.33), H-8 (δH 3.55 and 3.38)/H-10 (δH 1.33), H-9 (δH 2.40)/H-11 (δH 1.71); 13C NMR (100 MHz, CDCl3): δ = 146.7 (s), 138.0 (s), 128.4 (d)×2, 127.7 (d), 127.7 (d)×2, 110.5 (t), 80.1 (s), 73.5 (t), 70.8 (t), 51.5 (d), 47.6 (d), 39.4 (t), 27.2 (t), 23.5 (q), 18.7 (q); 13C NMR (100 MHz, C6D6): δ = 147.2 (s), 138.6 (s), 128.6 (d)×2, 128.5 (d), 127.8 (d)×2, 110.6 (t), 80.0 (s), 73.5 (t), 70.9 (t), 52.5 (d), 48.4 (d), 40.4 (t), 27.8 (t), 24.1 (q), 18.8 (q); IR (neat): ν∼ = 3446, 2962, 2871, 1645, 1098 cm−1; HRMS (ESI): m/z calcd for C17H24O2+Na+: 283.1674 [M+Na+]; found: 283.1677; Anal. Calcd for C17H24O2: C 78.42, H 9.29; found: C 78.26, H 9.03. cis-cyclopentane 8: Rf = 0.35 (hexane/AcOEt 3:1); [α]D25 −15.6 (c 1.27 in CHCl3); 1H NMR (400 MHz, CDCl3): δ = 7.35−7.27 (m, 5H), 4.85 (s, 1H), 4.74 (s, 1H), 4.40 (s, 2H), 3.40 (dd, J = 3.4, 9.8 Hz, 1H), 3.26 (dd, J = 7.7, 9.8 Hz, 1H), 3.08 (dd, J = 8.2, 16.2 Hz, 1H), 2.15 (dt, J = 3.4, 7.7 Hz, 1H), 1.89−1.73 (m, 4H), 1.76 (s, 3H), 1.62 (m, 1H), 1.41 (s, 3H); NOE correlations (H/H): H-4 (δH 3.08)/H-9 (δH 2.15), H-8 (δH 3.40 and 3.26)/H-10 (δH 1.41), H-8 (δH 3.40 and 3.26)/H-11 (δH 1.76); 13C NMR (100 MHz, CDCl3): δ = 145.7 (s), 138.5 (s), 128.3 (d)×2, 127.4 (d)×2, 127.4 (d), 110.5 (t), 81.9 (s), 73.2 (t), 68.7 (t), 52.7 (d), 47.0 (d), 39.3 (t), 26.3 (t), 25.5 (q), 23.7 (q); IR (neat): ν∼ = 3387, 2963, 2936, 2871, 1646, 1070 cm−1; HRMS (ESI): m/z calcd for C17H24O2+Na+: 283.1674 [M+Na+]; found: 283.1669; Anal. Calcd for C17H24O2: C 78.42, H 9.29; found: C 78.18, H 9.29.
(1R,2R,5S)-2-Hydroxy-2-methyl-5-(prop-1-en-2-yl)cyclopentanecarbaldehyde (11): A solution of trans-cyclopentane 7 (2.63 g, 10.1 mmol) in THF (50.5 mL) was added to a pre-prepared Na (2.53 g, 110 mmol)/liquid ammonia (50.5 mL) at −78 °C. After stirring for 20 min, NH4Cl (10.1 g, 189 mmol) was added to the mixture and excess NH3 was removed by warming. The reaction mixture was diluted with Et2O, washed with H2O and brine, dried over anhydrous Na2SO4, and then concentrated in vacuo. The residue was passed through a pad of silica gel (hexane/AcOEt = 3:2) and then concentrated in vacuo to give a crude product.
To a solution of IBX (5.66 g, 20.2 mmol) in DMSO (50.5 mL) was added a solution of the above crude product in THF (50.5 mL). After stirring for 2.5 h at room temperature, H2O was added to the mixture. After diluting with Et2O, the mixture was filtered through celite, washed with H2O and brine, dried over anhydrous Na2SO4, and then concentrated in vacuo. The residue was purified with flash column chromatography on silica gel (hexane/AcOEt = 2:1) to give aldehyde 11 (1.64 g, 96% yield for 2 steps) as a colorless oil. Rf = 0.30 (hexane/AcOEt 2:1); [α]D25 −29.5 (c 1.27 in CHCl3); 1H NMR (400 MHz, CDCl3): δ = 9.72 (d, J = 2.8 Hz, 1H), 4.74 (s, 1H), 4.73 (s, 1H), 2.99 (m, 1H), 2.75 (dd, J = 2.8, 9.8 Hz, 1H), 2.12 (brs, 1H), 1.95−1.67 (m, 4H), 1.72 (s, 3H), 1.34 (s, 3H); 13C NMR (100 MHz, CDCl3): δ = 203.7 (d), 145.7 (s), 110.5 (t), 81.3 (s), 65.8 (d), 45.7 (d), 41.9 (t), 28.0 (t), 25.3 (q), 20.3 (q); IR (neat): ν∼ = 3416, 2968, 1717, 1652, 1104 cm−1; HRMS (ESI): m/z calcd for C10H16O2+Na+: 191.1048 [M+Na+]; found: 191.1041; Anal. Calcd for C10H16O2: C 71.39, H 9.59; found: C 71.49, H 9.50.
4-((1R,2R,5S)-2-Hydroxy-2-methyl-5-(prop-1-en-2-yl)cyclopentyl)-2-methylene-4-oxobutyl acetate: To a stirring solution of aldehyde 11 (22.7 mg, 0.135 mmol) in THF (1.4 mL) were added 2-(bromomethyl)allyl acetate (65.2 mg, 0.338 mmol), Zn powder (44.2 mg, 0.675 mmol) and saturated aqueous NH4Cl solution (2.7 mL) at room temperature. After stirring for 2 h, H2O (1.4 mL) was slowly added. After stirring for 30 min, the mixture was diluted with Et2O, passed through a pad of celite (Et2O). The resulting solution was washed with H2O and brine, dried over anhydrous Na2SO4, and then concentrated in vacuo to give a crude product.
To a solution of IBX (133 mg, 0.405 mmol) in DMSO (1.4 mL) was added a solution of the above crude product in THF (1.4 mL). After stirring for 10 h at room temperature, H2O was added to the mixture. After diluting with Et2O, the mixture was filtered through celite, washed with H2O and brine, dried over anhydrous Na2SO4, and then concentrated in vacuo. The residue was purified with flash column chromatography on silica gel (hexane/AcOEt = 3:1) to give ketone (32.9 mg, 87% yield for 2 steps) as a colorless oil. Rf = 0.30 (hexane/AcOEt 3:1); [α]D25 −52.3 (c 1.17 in CHCl3); 1H NMR (400 MHz, CDCl3): δ = 5.24 (s, 1H), 5.03 (s, 1H), 4.69 (s, 2H), 4.60 (d, J = 13.2 Hz, 1H), 4.52 (d, J = 13.2 Hz, 1H), 3.39 (d, J = 16.9 Hz, 1H), 3.25 (d, J = 16.9 Hz, 1H), 3.08 (d, J = 10.6 Hz, 1H), 2.98 (dd, J = 7.8, 16.2 Hz, 1H), 2.06 (s, 3H), 1.78−1.89 (m, 3H), 1.70 (s, 3H), 1.65 (m, 1H), 1.21 (s, 3H); 13C NMR (100 MHz, CDCl3): δ = 208.3, 170.8, 146.4, 137.2, 117.4, 110.2, 81.3, 66.6, 64.9, 49.2, 47.2, 42.2, 27.3, 25.2, 20.9, 20.1; IR (neat): ν∼ = 3481, 3080, 2964, 1737, 1652, 1237 cm−1; HRMS (ESI): m/z calcd for C16H24O4+H+: 281.1753 [M+H+]; found: 281.1748; Anal. Calcd for C16H24O4: C 68.54, H 8.63; found: C 68.32, H 8.61.
(R)-4-((1R,2R,5S)-2-Hydroxy-2-methyl-5-(prop-1-en-2-yl)cyclopentyl)-2-methylenehept-6-ene-1,4-diol (13): To a stirring solution of above ketone (32.9 mg, 0.117 mmol) in Et2O (2.3 mL) were added allylmagnesium bromide (1.0 M solution in Et2O, 0.35 mL, 0.35 mmol) at 0 °C. After stirring for 30 min, the mixture was diluted with Et2O, washed with saturated aqueous NH4Cl solution, H2O and brine, dried over anhydrous Na2SO4, and then concentrated in vacuo. The residue was purified with flash column chromatography on silica gel (hexane/AcOEt = 3:2) to give triol 13 (27.8 mg, 85% yield) as a white scaly crystal. mp 103−104 °C; Rf = 0.25 (hexane/AcOEt 3:2); [α]D25 +38.4 (c 1.33 in CHCl3); 1H NMR (400 MHz, CDCl3): δ = 5.96 (m, 1H), 5.19 (s, 1H), 5.15 (d, J = 10.3 Hz, 1H), 5.05 (d, J = 16.2 Hz, 1H), 4.89 (s, 1H), 4.78 (s, 1H), 4.73 (s, 1H), 4.14 (d, J = 12.3 Hz, 1H), 4.07 (d, J = 12.3 Hz, 1H), 3.92−4.02 (m, 3H), 2.74 (d, J = 13.6 Hz, 1H), 2.67 (m, 1H), 2.45 (m, 1H), 2.34 (d, J = 13.6 Hz, 1H), 2.28 (d, J = 11.6 Hz, 1H), 2.01 (dd, J = 8.1, 14.8 Hz, 1H), 1.51−1.81 (m, 4H), 1.72 (s, 3H), 1.48 (s, 3H); 13C NMR (100 MHz, CDCl3): δ = 147.8, 144.8, 134.8, 118.6, 118.4, 112.1, 81.5, 75.6, 67.3, 53.6, 46.1, 43.6, 41.9, 40.8, 26.0, 25.0, 17.4; IR (KBr): ν∼ = 3292, 3075, 2959, 1699, 1653 cm−1; HRMS (ESI): m/z calcd for C17H28O3+H+: 303.1936 [M+H+]; found: 303.1947; Anal. Calcd for C17H28O3: C 72.82, H 10.06; found: C 72.83, H 10.07.
(R)-5-Allyl-5-((1R,2R,5S)-2-hydroxy-2-methyl-5-(prop-1-en-2-yl)cyclopentyl)-3-methylenedihydro-furan-2(3H)-one (14): To a stirring solution of triol 13 (27.8 mg, 0.099 mmol) in CH2Cl2 (5.0 mL) was added manganese dioxide (556 mg, 6.40 mmol) at room temperature. After stirring for 1 h, the mixture was diluted with Et2O, passed through a pad of silica gel (hexane/AcOEt = 3:1), and then concentrated in vacuo. The residue was purified with flash column chromatography on silica gel (hexane/AcOEt = 3:1) to give α-methylene-lactone 14 (27.4 mg, quantitative yield) as a colorless oil. Rf = 0.30 (hexane/AcOEt 3:1); [α]D25 +3.81 (c 0.93 in CHCl3); UV λmax (MeOH) nm (ε): 208 (8900, sh); 1H NMR (400 MHz, CDCl3): δ = 6.23 (t, J = 3.0 Hz, 1H), 5.74 (ddt, J = 9.2, 17.0, 7.0 Hz, 1H), 5.58 (t, J = 2.5 Hz, 1H), 5.15 (d, J = 9.2 Hz, 1H), 5.12 (d, J = 17.0 Hz, 1H), 4.75 (s, 1H), 4.70 (s, 1H), 3.05 (dt, J = 17.6, 3.1 Hz, 1H), 2.69 (dt, J = 17.6, 2.3 Hz, 1H), 2.45−2.62 (m, 3H), 2.42 (d, J = 10.2 Hz, 1H), 2.17 (brs, 1H), 1.77−1.89 (m, 2H), 1.75 (s, 3H), 1.52−1.68 (m, 2H), 1.33 (s, 3H); 13C NMR (100 MHz, CDCl3): δ = 169.8, 148.3, 135.0, 131.5, 122.4, 120.7, 111.8, 86.9, 81.0, 56.2, 46.4, 45.4, 41.9, 34.6, 27.6, 24.9, 18.4; IR (neat): ν∼ = 3444, 3075, 2955, 1746, 1649, 1272 cm−1; HRMS (ESI): m/z calcd for C17H24O3+Na+: 299.1627 [M+Na+]; found: 299.1623; Anal. Calcd for C17H24O3: C 73.88, H 8.75; found: C 73.78, H 8.74.
(2R,3'R,3a'R,7a'S)-3'-Hydroxy-3',4,7'-trimethyl-1',2',3',3a',5',7a'-hexahydro-5H-spiro[furan-2,4'-inden]-5-one (8-epi-sinularianin B, 15): To a stirring solution of α-methylene-lactone 14 (27.4 mg, 0.099 mmol) in EtOH (19.8 mL) was added rhodium chloride trihydrate (26.1 mg, 0.099 mmol) at room temperature and then refluxed for 5 h. The mixture was cooled to room temperature, diluted with EtOH, passed through a pad of silica gel (Et2O), and then concentrated in vacuo to give a crude product.
To a stirring solution of the above crude α,β-unsaturated lactone in degassed toluene (19.8 mL) was added Grubbs 2nd generation catalyst (17.0 mg, 0.020 mmol) at room temperature. After stirring for 12 h, the reaction mixture was diluted with Et2O and the residue was passed through a pad of silica gel (hexane/AcOEt = 12:1) and then concentrated in vacuo. The residue was purified with flash column chromatography on silica gel (hexane/AcOEt = 2:1) to give 8-epi-sinularianin B (15) (7.4 mg, 30% yield for 2 steps) as a colorless oil. Rf = 0.30 (hexane/AcOEt 2:1); [α]D25 −52.2 (c 0.24 in CHCl3); UV λmax (MeOH) nm (ε): 213 (5400, sh); 1H NMR (400 MHz, CDCl3): δ = 7.19 (d, J = 1.5 Hz, 1H), 5.23 (s, 1H), 2.81 (m, 1H), 2.46 (d, J = 13.1 Hz, 1H), 2.33 (m, 1H), 1.89−1.99 (m, 4H), 1.91 (d, J = 1.5 Hz, 3H), 1.71 (s, 3H), 1.70 (m, 1H), 1.51 (m, 1H), 1.06 (s, 3H); 13C NMR (100 MHz, CDCl3): δ = 173.5, 150.8, 136.8, 129.2, 118.4, 88.2, 78.8, 58.8, 45.5, 40.8, 40.6, 27.9, 26.5, 19.8, 10.7; IR (neat): ν∼ = 3435, 2963, 2925, 2849, 1747, 1645 cm−1; HRMS (ESI): m/z calcd for C15H20O3+Na+: 271.1310 [M+Na+]; found: 271.1317; Anal. Calcd for C15H20O3: C 72.55, H 8.12; found: C 72.26, H 8.13.
1-((1R,2R,5S)-2-Hydroxy-2-methyl-5-(prop-1-en-2-yl)cyclopentyl)but-3-en-1-one (16): To a stirring solution of aldehyde 11 (432 mg, 2.57 mmol) in THF (25.7 mL) were added 3-bromoprop-1-ene (777 mg, 6.42 mmol), Zn powder (841 mg, 12.9 mmol) and saturated aqueous NH4Cl solution (51.4 mL) at room temperature. After stirring for 2 h, H2O (25.7 mL) was slowly added. After stirring for 30 min, the mixture was diluted with Et2O, passed through a pad of celite (Et2O). The resulting solution was washed with H2O and brine, dried over anhydrous Na2SO4, and then concentrated in vacuo to give a crude product.
To a solution of IBX (1.44 g, 5.14 mmol) in DMSO (12.9 mL) was added a solution of the above crude product in THF (12.9 mL). After stirring for 3 h at room temperature, H2O was added to the mixture. After diluting with Et2O, the mixture was filtered through celite, washed with H2O and brine, dried over anhydrous Na2SO4, and then concentrated in vacuo. The residue was purified with flash column chromatography on silica gel (hexane/AcOEt = 2:1) to give β,γ-unsaturated ketone 16 (493 mg, 92% yield for 2 steps) as a colorless oil. Rf = 0.25 (hexane/AcOEt 2:1); [α]D25 −65.5 (c 1.00 in CHCl3); 1H NMR (400 MHz, CDCl3): δ = 5.92 (dddd, J = 6.8, 7.0, 10.2, 17.2 Hz, 1H), 5.17 (d, J = 10.2 Hz, 1H), 5.12 (d, J = 17.2 Hz, 1H), 4.69 (s, 2H), 3.35 (dd, J = 6.8, 17.1 Hz, 1H), 3.23 (dd, J = 7.0, 17.1 Hz, 1H), 3.05 (d, J = 10.4 Hz, 1H), 2.98 (d, J = 7.8 Hz, 1H), 1.95 (brs, 1H), 1.70−1.89 (m, 3H), 1.62−1.71 (m, 1H), 1.69 (s, 3H), 1.18 (s, 3H); 13C NMR (100 MHz, CDCl3): δ = 209.4, 146.4, 130.4, 118.8, 110.0, 81.3, 65.2, 49.4, 47.2, 42.3, 27.6, 25.5, 20.4; IR (neat): ν∼ = 3446, 3080, 1966, 1699, 1646 cm−1; HRMS (ESI): m/z calcd for C13H20O2+Na+: 231.1361 [M+Na+]; found: 231.1370; Anal. Calcd (%) for C13H20O2: C 74.96, H 9.68; found: C 75.23, H 9.80.
(3R,3aR,4R,7aS)-4-(tert-Butyldimethylsilyloxy)-3-hydroxy-3,7-dimethyl-2,3,3a,4,5,7a-hexahydro-1H-indene-4-carbaldehyde (20): To a stirring solution of aldehyde 11 (121 mg, 0.718 mmol) in THF (109 mL) were added TBSCN (122 mg, 0.862 mmol), and PNPCl (41.3 mg, 0.0718 mmol) at room temperature. After stirring for 2 h, TMSCN (0.14 mL, 1.12 mmol) was added at the same temperature. The mixture was concentrated in vacuo, and the residue was passed through a pad of silica gel (hexane/AcOEt = 15:1) and then concentrated in vacuo to give a crude TMS-protected cyanohydrin 18.
A solution of the above crude TMS-protected cyanohydrin 18 in THF (4.00 mL) was added to a pre-prepared LDA (0.39 M solution in THF, 4.97 mL, 1.94 mmol) at −78 °C and then allowed to warm to 0 °C. After stirring for 40 min, the mixture was cooled to −78 °C, and then pre-mixed allyl bromide (0.31 mL, 3.59 mmol) and HMPA (0.31 mL, 1.80 mmol) was introduced to the mixture. After stirring for 15 min at the same temperature, the reaction mixture was diluted with Et2O, washed with saturated aqueous NH4Cl solution, H2O and brine, dried over anhydrous Na2SO4, and then concentrated in vacuo. The residue was passed through a pad of silica gel (hexane/AcOEt = 30:1) and then concentrated in vacuo to give a crude diene 19.
To a stirring solution of the above crude diene 19 in degassed 1,2-dichloroethane (144 mL) was added Grubbs 2nd generation catalyst (61.0 mg, 0.0718 mmol) at room temperature, and then refluxed. After stirring for 6 h, cooled to room temperature, and then added DMSO (0.51 mL, 7.18 mmol). After stirring for 12 h, the solvent was removed in vacuo, the residue was diluted with Et2O and the residue was passed through a pad of silica gel (hexane/AcOEt = 12:1) and then concentrated in vacuo to give a crude product.
To a stirring solution of the above crude product in toluene (14.4 mL) was added DIBAH (1.02 M solution in hexane, 1.41 mL, 1.44 mmol) at −78 °C and then allowed to warm to 0 °C. After stirring for 30 min, the mixture was cooled to −78 °C, and then Et2O (14.4 mL), saturated aqueous NH4Cl solution (7.18 mL), and 0.5 M aqueous H2SO4 solution (14.4 mL) were introduced to the mixture. The mixture was then allowed to warm to room temperature and then the reaction mixture was diluted with Et2O, washed with saturated aqueous NH4Cl solution, H2O and brine, dried over anhydrous Na2SO4, and then concentrated in vacuo. The residue was passed through a pad of silica gel (hexane/AcOEt = 15:1) and then concentrated in vacuo to give a crude product.
To a stirring solution of the above crude product in THF (7.18 mL) were added acetic acid (0.82 mL, 14.4 mmol) and TBAF (1.0 M solution in THF, 7.18 mL, 7.18 mmol) at room temperature, and then allowed to warm to 40 °C. After stirring for 5 h, the reaction mixture was diluted with Et2O, washed with saturated aqueous NaHCO3 solution, H2O and brine, dried over anhydrous Na2SO4, and then concentrated in vacuo. The residue was purified with flash column chromatography on silica gel (hexane/AcOEt = 4:1) to give α-siloxyaldehyde 20 (156 mg, 67% yield for 5 steps) as a white needle-like crystalline solid. Rf = 0.60 (hexane/AcOEt 2:1); mp 77−78 °C (recrystallized from hexane/AcOEt); [α]D25 +9.70 (c 1.51 in CHCl3); 1H NMR (400 MHz, CDCl3): δ = 9.77 (s, 1H), 5.21 (s, 1H), 2.58 (m, 1H), 2.43 (m, 1H), 1.99 (d, J = 12.6 Hz, 1H), 1.96−1.73 (m, 4H), 1.69 (3H, s), 1.45 (m, 1H), 1.30 (s, 3H), 0.85 (s, 9H), 0.18 (s, 3H), 0.02 (s, 3H); 13C NMR (100 MHz, CDCl3): δ = 204.0 (d), 137.4 (s), 116.5 (d), 81.3 (s), 78.4 (s), 58.9 (d), 41.5 (t), 39.8 (d), 35.3 (t), 27.9 (q), 25.8 (q)×3, 25.6 (t), 20.2 (q), 18.6 (s), −2.5 (q), −3.1 (q); IR (KBr): ν∼ = 3420, 2956, 2931, 2857, 1733, 1653 cm−1; HRMS (ESI): m/z calcd for C10H16O2+Na+: 347.2018 [M+Na+]; found: 347.2023; Anal. Calcd for C18H32O3Si: C 66.62, H 9.94; found: C 66.54, H 9.90.
(4aS,4a1R,7aS,9aR)-4a-(tert-Butyldimethylsilyloxy)-3,7,9a-trimethyl-4a,5,7a,8,9,9a-hexahydro-indeno[1,7-bc]oxepin-2(4a1H)-one (21): To a stirring solution of α-siloxyaldehyde 20 (101 mg, 0.311 mmol) in CH2Cl2 (6.22 mL) were added 2-(diethoxyphosphoryl)propanoic acid (211 mg, 0.933 mmol), and WSC (179 mg, 0.933 mmol) at room temperature. After stirring for 30 min, the mixture was diluted with Et2O, washed with saturated aqueous NaHCO3 solution, H2O and brine, and then concentrated in vacuo. The residue was passed through a pad of silica gel (hexane/AcOEt = 3:2) and then concentrated in vacuo to give a crude product.
To a stirring solution of the above crude product in THF (62.4 mL) was added KOtBu (48.9 mg, 0.435 mmol) at room temperature and then allowed to warm to 60 °C. After stirring for 2 h, the mixture was cooled to room temperature and then the reaction mixture was diluted with Et2O, washed with saturated aqueous NH4Cl solution, H2O and brine, and then concentrated in vacuo. The residue was purified with flash column chromatography on silica gel (hexane/AcOEt = 12:1) to give α,β-unsaturated lactone 21 (88.1 mg, 78% yield for 2 steps) as a colorless oil. Rf = 0.35 (hexane/AcOEt 12:1); [α]D25 +162 (c 1.29 in CHCl3); 1H NMR (400 MHz, CDCl3): δ = 6.20 (s, 1H), 5.14 (s, 1H), 2.46 (m, 1H), 2.31 (dd, J = 11.4, 13.8 Hz, 1H), 2.24 (m, 1H), 2.13 (m, 1H), 2.08 (d, J = 12.7 Hz, 1H), 2.05 (s, 3H), 1.96 (m, 1H), 1.86 (dt, J = 14.8, 8.8 Hz, 1H), 1.68 (s, 3H), 1.51 (s, 3H), 1.49 (m, 1H), 0.83 (s, 9H), 0.11 (s, 6H); 13C NMR (100 MHz, CDCl3): δ = 167.9 (s), 140.7 (d), 137.0 (s), 127.7 (s), 117.7 (d), 85.8 (s), 73.1 (s), 58.7 (d), 41.3 (d), 41.1 (t), 40.7 (t), 26.5 (t), 25.7 (q)×3, 24.6 (q), 24.1 (q), 20.1 (q), 18.4 (s), −2.1 (q), −2.4 (q); IR (neat): ν∼ = 2957, 2930, 2857, 1698, 1684, 1254 cm−1; UV/Vis: λmax (MeOH)/nm 215sh (ε /dm3 mol−1 cm−1 9100); HRMS (ESI): m/z calcd for C21H34O3Si+H+: 363.2355 [M+H+]; found: 363.2350; Anal. Calcd for C21H34O3Si: C 69.56, H 9.45; found: C 69.39, H 9.49.
Sinularianin B (1): To a stirring solution of α,β-unsaturated lactone 21 (71.0 mg, 0.196 mmol) in THF (1.96 mL) was added TBAF (1.0 M solution in THF, 0.49 mL, 0.490 mmol) at room temperature. After stirring for 2 h, a suspension of 4Å molecular sieves (14.0 mg) and K2CO3 (271 mg, 1.96 mmol) in MeOH (9.80 mL) was added and the mixture was warmed to 40 °C. After stirring for 36 h, the solvent was removed in vacuo. The residue was passed through a pad of silica gel (Et2O) and then concentrated in vacuo. The residue was purified with flash column chromatography on silica gel (hexane/AcOEt = 2:1) to give sinularianin B (1) (48.0 mg, 99% yield) as a colorless oil. Rf = 0.15 (hexane/AcOEt 2:1); [α]D25 −111 (c 1.55 in CHCl3); 1H NMR (500 MHz, CDCl3): δ = 7.16 (d, J = 1.5 Hz, 1H), 5.24 (dd, J = 1.9, 2.3 Hz, 1H), 2.57 (m, 1H), 2.53 (m, 1H), 1.98 (d, J = 12.8 Hz, 1H), 1.95−1.75 (m, 4H), 1.93 (d, J = 1.5 Hz, 3H), 1.71 (s, 3H), 1.47 (brs, 1H), 1.43 (m, 1H), 1.12 (s, 3H); 13C NMR (125 MHz, CDCl3): δ = 173.7 (s), 152.2 (d), 137.3 (s), 129.1 (s), 117.2 (d), 85.4 (s), 78.4 (s), 56.6 (d), 41.8 (t), 40.9 (d), 39.7 (t), 26.0 (q), 25.3 (t), 20.2 (q), 10.6 (q); IR (neat): ν∼ = 3445, 2962, 1734, 1658 cm−1; UV/Vis λmax (MeOH)/nm 213sh (ε /dm3 mol−1 cm−1 9600); HRMS (ESI): m/z calcd for C15H20O3+Na+: 271.1310 [M+Na+]; found: 271.1313; Anal. Calcd for C15H20O3: C 72.55, H 8.12; found: C 72.37, H 7.93.
ACKNOWLEDGEMENT
The authors are grateful to Professor Jyh-Horng Sheu, National Sun Yat-sen University, for providing the NMR spectra of sinularianin B.
References
1. C.-H. Chao, C.-H. Hsieh, S.-P. Chen, C.-K. Lu, C.-F. Dai, and J.-H. Sheu, Tetrahedron Lett., 2006, 47, 5889. CrossRef
2. a) H. R. W. Dharmaratne, N. P. D. Nanayakkara, and I. A. Khan, Planta Med., 2002, 68, 661; CrossRef b) C. Paul, A. W. Koning, and J. Muhle, Phytochemistry, 2001, 57, 307; CrossRef c) G. Francois, C. Philippe, and K. Ilias, Phytochemistry, 1995, 40, 1421; CrossRef d) R. Bos, H. Hendriks, A. P. Bruins, J. Kloosterman, and G. Sipma, Phytochemistry, 1986, 25, 133. CrossRef
3. M. Kobayashi, T. Yasuzawa, Y. Kyogoku, M. Kido, and I. Kitagawa, Chem. Pharm. Bull., 1982, 30, 3431. CrossRef
4. K. Ota and H. Miyaoka, Chem. Commun., 2013, 49, 8148. CrossRef
5. a) H. Miyaoka, T. Shigemoto, I. Shinohara, A. Suzuki, and Y. Yamada, Tetrahedron, 2000, 56, 8077; CrossRef b) K. Ota, T. Kurokawa, E. Kawashima, and H. Miyaoka, Tetrahedron, 2009, 65, 8668. CrossRef
6. D. Menche, J. Hassfeld, J. Li, K. Mayer, and S. Rudolph, J. Org. Chem., 2009, 74, 7220. CrossRef
7. T. Katsuki and K. B. Sharpless, J. Am. Chem. Soc., 1980, 102, 5974. CrossRef
8. T. Ema, D. Tanida, and T. Sakai, Org. Lett., 2006, 8, 3773. CrossRef
9. M. Mohri, H. Kinoshita, K. Inomata, and H. Kotake, Chem. Lett., 1985, 451. CrossRef
10. B. M. Trost, M. R. Machacek, and H. C. Tsui, J. Am. Chem. Soc., 2005, 127, 7014. CrossRef
11. a) J. Tsuji, I. Minami, and I. Shimizu, Synthesis, 1986, 623; CrossRef b) T. Konno, T. Takehana, M. Mishima, and T. Ishihara, J. Org. Chem., 2006, 71, 3545. CrossRef
12. M. Frigerio and M. Santagostino, Tetrahedron Lett., 1994, 35, 8019. CrossRef
13. G. W. Breton, J. H. Shugart, C. A. Hughey, B. P. Conrad, and S. M. Perala, Molecules, 2001, 6, 655. CrossRef
14. a) R. M. Carlson, Tetrahedron Lett., 1978, 19, 111; CrossRef b) H. Nishiyama, H. Yokoyama, S. Narimatsu, and K. Itoh, Tetrahedron Lett., 1982, 23, 1267. CrossRef
15. C. W. Jefford, J.-C. Rossier, J. Boukouvalas, A. W. Sledeski, and P.-Z. Huang, J. Nat. Prod., 2004, 67, 1383. CrossRef
16. M.-A. Lacour, N. J. Rahier, and M. Taillefer, Chem. Eur. J., 2011, 17, 12276. CrossRef
17. M. Scholl, T. M. Trnka, J. P. Morgan, and R. H. Grubbs, Tetrahedron Lett., 1999, 40, 2247. CrossRef
18. a) M. Nahmany and A. Melman, Org. Lett., 2001, 3, 3733; CrossRef b) R. Shelkov, M. Nahmany, and A. Melman, J. Org. Chem., 2002, 67, 8975. CrossRef
19. a) L. Horner, H. M. R. Hoffmann, and H. G. Wippel, Ber., 1958, 91, 61; CrossRef b) W. S. Wadsworth and W. D. Emmons, J. Am. Chem. Soc., 1961, 83, 1733; CrossRef c) W. S. Wadsworth, Org. React., 1977, 25, 73.