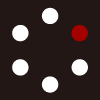
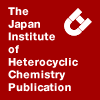
HETEROCYCLES
An International Journal for Reviews and Communications in Heterocyclic ChemistryWeb Edition ISSN: 1881-0942
Published online by The Japan Institute of Heterocyclic Chemistry
e-Journal
Full Text HTML
Received, 7th February, 2014, Accepted, 26th February, 2014, Published online, 4th March, 2014.
DOI: 10.3987/COM-14-12957
■ Sequential Radical Addition and Fischer-Type Indolization Reactions of Conjugated Hydrazones for the Preparation of α-Alkylindole-3-acetic Acids
Yuta Ito, Masafumi Ueda,* Naomi Matsuda, Yuika Nishida, and Okiko Miyata*
Department of Medicinal Chemistry, Kobe Pharmaceutical University, 4-19-1, Motoyamakitamachi, Higashinada, Kobe 658-8558, Japan
Abstract
A domino reaction, consisting of sequential regioselective radical addition and Fischer-type indolization reactions, has been developed for the construction of α-alkylindole-3-acetates from conjugated hydrazones.Radical reactions represent a powerful synthetic tool for the construction of carbon–carbon bonds under mild and neutral conditions. In particular, carbon radical addition reaction to hydrazone can be useful for the synthesis of amines and amino acids.1 In contrast to the rich chemistry associated with the 1,2-addition of carbon radicals to hydrazones, less is known about the 1,4-addition reaction.2 To further develop the scope of hydrazones as radical acceptors, we will investigate a domino reaction based on 1,4-radical addition to conjugated hydrazones 1 bearing the aryl group at nitrogen atom (Scheme 1).
We anticipated that an alkyl radical would add to 1 in a β-selective manner and that subsequent trapping of the resulting intermediate with triethylborane would generate N-boryl enehydrazine A, which would undergo a Fischer-type indolization3 reaction to give the α-alkylindole-3-acetate 2. To the best of our knowledge, this reaction would be the first reported example of a domino reaction involving sequential radical addition and Fischer-type indolization reactions. Furthermore, indole-3-acetic acid is a key structural unit of indometacin which is a particularly valuable anti-inflammatory drug,4 and indole-3-acetic acids bearing an alkyl chain at their α-position were recently found to be potent inhibitors of plant growth.5 The development of a simple synthetic process for the construction of α-alkylindole-3-acetic acid derivatives is therefore highly desirable from the perspective of the agricultural and pharmaceutical industries, as well as synthetic organic chemistry. As a part of our ongoing research towards exploring the domino reactions of conjugated imines,6 we report herein the development of a novel alkylative Fischer indolization reaction, which proceeds via the addition of an alkyl radical to a conjugated hydrazone followed by a [3,3]-sigmatropic rearrangement.
Initially, we chose conjugated hydrazone 3a bearing a p-methoxyphenyl group on its terminal nitrogen atom as a model substrate to optimize the reaction conditions (Table 1). The treatment of 3a with triethylborane as an ethyl radical source in benzene at room temperature led to the complete consumption of the starting material within 1 h, α-ethyl-5-methoxyindole-3-acetic acid ethyl ester (4a) being formed as the major product, albeit in low yield (entry 1). To improve the efficiency of the transformation, the domino reaction was subsequently conducted at reflux and the indole 4a was obtained in an improved yield of 35% (entry 2).
The solvent polarity and reaction temperature had a significant impact on the outcome of the reaction. For example, when the domino reaction was conducted in MeCN at reflux it gave 4a in 43% yield, whereas refluxing in chlorobenzene or DMF led to a decrease in the chemical yield (entries 3-5). The effect of the addition of a Lewis acid to the domino reaction was also investigated. It is noteworthy that the addition of zinc salts7 to the reaction led to a significant improvement in the chemical yield (entries 8-10). The reaction of 3a in the presence of 3 equivalents of ZnCl2 or ZnBr2 gave 4a in good yield (entries 8 and 9). Furthermore, ZnI2 was found to be the most effective Lewis acid for this particular transformation (entry 10). When ZnI2 was used as the Lewis acid, only 0.5 equivalents were required to provide a high chemical yield of 4a (entry 12). Attempts to further reduce the amount of ZnI2 to 0.1 equivalent, however, led to a decrease in the chemical yield (entry 13).
With the optimized conditions in hand, we proceeded to investigate the effect of various substituents on the phenyl group of the hydrazone moiety (Table 2). Substrates bearing an electron-donating group such as 4-OBn, 4-NHMe and 4-SMe reacted smoothly under the optimized conditions to afford the corresponding 5-benzyloxyindole 4b, 5-acetamidoindole 4c and 5-methylthioindole 4d in good yields, respectively (entries 1-3). A similar good yield was observed for the indole 4e bearing a methyl group (entry 4), whereas substrate 3f bearing a bulky tert-butyl group gave the corresponding indole 4f in slightly lower yield (entry 5). In contrast to substrates with an electron-donating substituent, the application of the optimized reaction conditions to hydrazones bearing a halogen atom or no substituent led to a decrease in the chemical yield or decomposition, probably due to inefficient Fischer indolization reaction (entries 6-8). Interestingly, the reaction of 1-naphthylhydrazone 3j proceeded effectively to afford the corresponding indole 4j in 92% yield, whereas the 2-naphthylhydrazone 3k gave 4k in 62% yield without the concomitant formation of any regioisomer (entries 9 and 10). Substrate 3l bearing an o-methoxy group gave 4l in relatively lower yield, most likely because of steric repulsion from the ortho substituent (entry 11).
To synthesize various α-alkylindole-3-acetic acid derivatives and further expand the scope of this reaction, we investigated the domino reaction using a range of different alkyl iodides as the alkyl radical precursors (Table 3). The domino reaction with more stable and nucleophilic secondary alkyl radical such as isopropyl, sec-butyl and cycloalkyl radicals proceeded effectively to give the α-alkylindole-3-acetates 5a–d in good to high yields (entries 1-4). On the other hand, relatively lower yields were observed in the reaction with electrophilic or much more stable alkyl radicals generated from benzyl bromide, iodoacetate and iodoacetonitrile, because the addition reaction of these radicals to electron-deficient alkene are generally less efficient (entries 5-7).8
A plausible reaction pathway is shown in Scheme 2. The first step would involve the generation of an ethyl radical by the reaction of triethylborane with oxygen. Iodine atom-transfer from an alkyl iodide to the ethyl radical would then produce an alkyl radical.8 The regioselective addition of the alkyl radical to the α position of the carbonyl group would give aminyl radical B, which would be trapped by triethylborane as a radical terminator to generate N-boryl enehydrazine C. The N-boryl enehydrazine C would then undergo a [3,3]-sigmatropic rearrangement to give the α–arylimine D, with subsequent cyclization and aromatization giving rise to the α-alkylindole-3-acetate 5.
In summary, we have developed a novel domino reaction for the construction of α-alkylindole-3-acetates via the addition of alkyl radicals to conjugated hydrazones to generate the corresponding N-boryl enehydrazines followed by a Fischer-type indolization. This methodology allows for straightforward synthesis of various α-alkylindole-3-acetates from simple substrate.
ACKNOWLEDGEMENTS
This work was supported by Grants-in-Aid from the Ministry of Education, Culture, Sports, Science and Technology of Japan (MEXT), the MEXT-Supported Program for the Strategic Research Foundation at Private Universities, and Takeda Science Foundation. M.U. is grateful to the Mitsubishi Chemical Award in Synthetic Organic Chemistry of Japan.
References
1. a) G. K. Friestad, A. Ji, J. Baltrusaitis, C. S. Korapala, and J. Qin, J. Org. Chem., 2012, 77, 3159; CrossRef b) G. K. Friestad, A. Ji, C. S. Korapala, and J. Qin, Org. Biomol. Chem., 2011, 9, 4039; CrossRef c) G. K. Friestad and K. Banerjee, Org. Lett., 2009, 11, 1095; CrossRef d) G. K. Friestad and A. Ji, Org. Lett., 2008, 10, 2311; CrossRef e) G. K. Friestad, J.-C. Marié, J. Baltrusaitis, Y. Suh, and J. Qin, J. Org. Chem., 2006, 71, 7016; CrossRef f) G. K. Friestad, C. Draghici, J. Baltrusaitis, M. Soukri, and J. Qin, J. Org. Chem., 2005, 70, 6330; CrossRef g) G. K. Friestad, J.-C. Marié, and A. M. Deveau, Org. Lett., 2004, 6, 3249; CrossRef h) G. K. Friestad and J. Qin, J. Am. Chem. Soc., 2001, 123, 9922; CrossRef i) G. K. Friestad and J. Qin, J. Am. Chem. Soc., 2000, 122, 8329; CrossRef j) S. Y. Kim, S. J. Kim, and D. O. Jang, Chem. Eur. J., 2010, 16, 13046; CrossRef k) M. Fernández and R. Alonso, Org. Lett., 2003, 5, 2461; CrossRef l) M. Ueda, H. Miyabe, H. Sugino, and T. Naito, Org. Biomol. Chem., 2005, 3, 1124; CrossRef m) M. Ueda, H. Miyabe, A. Nishimura, H. Sugino, and T. Naito, Tetrahedron: Asymmetry, 2003, 14, 2857; CrossRef n) O. Miyata, K. Muroya, T. Kobayashi, R. Yamanaka, S. Kajiya, J. Koide, and T. Naito, Tetrahedron, 2002, 58, 4459. CrossRef
2. a) M. Ueda, E. Iwasada, H. Miyabe, O. Miyata, and T. Naito, Synthesis, 2010, 1999; CrossRef b) M. Ueda, H. Miyabe, M. Torii, T. Kimura, O. Miyata, and T. Naito, Synlett, 2010, 1341; CrossRef c) M. Ueda, H. Miyabe, T. Kimura, E. Kondoh, T. Naito, and O. Miyata, Org. Lett., 2009, 11, 4632. CrossRef
3. a) B. Robinson, “The Fischer Indole Synthesis”, John Wiley and Sons, Chichester, 1982; b) B. Robinson, Chem. Rev., 1963, 63, 373; CrossRef c) B. Robinson, Chem. Rev., 1969, 69, 227. CrossRef
4. E. J. Corey, B. Czakó, and L. Kürti, “Molecules and Medicine”, Wiley, Hoboken, 2007.
5. a) K. Hayashi, J. Neve, M. Hirose, A. Kuboi, Y. Shimada, S. Kepinski, and H. Nozaki, ACS Chem. Biol., 2012, 7, 590; CrossRef b) K. Hayashi, X. Tan, N. Zheng, T. Hatate, Y. Kimura, S. Kepinski, and H. Nozaki, Proc. Natl. Acad. Sci. U. S. A., 2008, 105, 5632. CrossRef
6. a) M. Ueda, H. Matsubara, K. Yoshida, A. Sato, T. Naito, and O. Miyata, Chem. Eur. J., 2011, 17, 1789; CrossRef b) M. Ueda, Y. Ikeda, A. Sato, Y. Ito, M. Kakiuchi, H. Shono, T. Miyoshi, T. Naito, and O. Miyata, Tetrahedron, 2011, 67, 4612; CrossRef c) M. Ueda, A. Sato, Y. Ikeda, T. Miyoshi, T. Naito, and O. Miyata, Org. Lett., 2010, 12, 2594; CrossRef d) M. Ueda, H. Miyabe, H. Shimizu, H. Sugino, O. Miyata, and T. Naito, Angew. Chem. Int. Ed., 2008, 47, 5600; CrossRef e) M. Ueda, H. Miyabe, H. Sugino, O. Miyata, and T. Naito, Angew. Chem. Int. Ed., 2005, 44, 6190. CrossRef
7. ZnI2 would promote Fischer indolization reaction, see; a) F. Zhan and G. Liang, Angew. Chem. Int. Ed., 2013, 52, 1266; CrossRef b) J. G. Phillips, M. Jaworska, and W. Lew, Synthesis, 2010, 714; CrossRef c) A. Pews-Davtyan, A. Tillack, A.-C. Schmöle, S. Ortinau, M. J. Frech, A. Rolfs, and M. Beller, Org. Biomol. Chem., 2010, 8, 1149; CrossRef d) T. M. Lipińska and S. J. Czarnocki, Org. Lett., 2006, 8, 367; CrossRef e) L. H. Franco and J. A. Palermo, Chem. Pharm. Bull., 2003, 51, 975; CrossRef f) C. Cao, Y. Shi, and A. L. Odom, Org. Lett., 2002, 4, 2853. CrossRef
8. H. Miyabe, M. Ueda, and T. Naito, Synlett, 2004, 1140. CrossRef