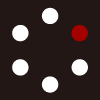
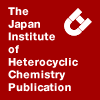
HETEROCYCLES
An International Journal for Reviews and Communications in Heterocyclic ChemistryWeb Edition ISSN: 1881-0942
Published online by The Japan Institute of Heterocyclic Chemistry
e-Journal
Full Text HTML
Received, 7th February, 2014, Accepted, 24th March, 2014, Published online, 4th April, 2014.
DOI: 10.3987/COM-14-12958
■ C-H Trifluoromethylations of 1,3-Dimethyluracil and Reactivity of the Products in C-H Arylations
Miroslava Ćerňová, Radek Pohl, Blanka Klepetářová, and Michal Hocek*
Institute of Organic Chemistry and Biochemistry, Academy of Sciences of the Czech Republic, Flemingovo nam. 2, CZ-16610 Prague 6, Czech Republic
Abstract
Diverse electrophilic, nucleophilic and radical C-H trifluoromethylations of 1,3-dimethyluracil were systematically studied in order to prepare either 5- or 6-(trifluoromethyl)uracil derivatives. Electrophilic reagents led only to dimeric bis-uracil products, whereas the radical trifluoromethylation by CF3SO2Na in presence of t-BuOOH gave 1,3-dimethyl-5-(trifluoromethyl)uracil (2) in good yield. The 6-(trifluoromethyl)uracil derivative 3 was only prepared in low yield and in a mixture with 2 by Ir-catalyzed borylation followed by treatment with the Togni's reagent. Attempted Pd-catalyzed C-H arylations of 2 in the presence of Cs2CO3 gave mixtures of de-trifluoromethylated products, whereas the reaction with 4-iodotoluene in the presence of CsF gave the desired 6-aryl-5-trifluoromethyluracil derivative 8 in moderate yield and the reaction was not general for other aryl halides.INTRODUCTION
5-(Trifluoromethyl)uracil and –uridine are known as antitumor and antiviral compounds1 and in the past they were prepared either by heterocyclization2 or by cross-coupling of 5-halogenouracil derivatives with diverse CF3-M species.3 As fluorinated compounds in general, and trifluoromethyl derivatives of heterocycles in particular, are of great current interest in medicinal chemistry,4 in recent years, diverse methodologies of trifluoromethylations have been developed, either based on improved Cu or Pd-catalyzed cross-coupling reactions of (hetero)aryl halides or boronates,5 or based on direct C-H activations. The C-H trifluoromethylations can proceed either as metal catalyzed (Cu or Pd) reactions with either electrophilic6 or nucleophilic7 "CF3" species or as radical reactions with CF3· generated either by photoredox catalysis,8 Fenton oxidation9 or by reaction with peroxides.10 A two-step procedure based on Ir-catalyzed C-H borylation followed by electrophilic trifluoromethylation has also been reported.11
Recently we have developed regioselective C-H arylations12 of 1,3-dialkyluracils which in the presence of Pd catalysts proceeded preferentially at position 5, whereas in presence of CuI at position 6. Also other groups have extensively studied and used these reactions in synthesis of either 6- or 5-substituted uracil derivatives.13 However, synthesis of 5,6-disubstituted uracils has never been achieved by these reactions. Based on the above mentioned biological activities of 5-trifluoromethyluracils, the logical continuation of our project was to attempt the synthesis of uracil derivatives bearing one trifluoromethyl and one aryl group at positions 5 and 6 by two consecutive C-H activations.
RESULTS AND DISCUSSION
C-H trifluoromethylation of 1,3-dimethyluracil8a,9a or uracil10 has been previously reported by radical reactions to proceed at position 5 in good yields. Only trace amounts (<1%) of 6-trifluoromethyluracil was detected using Fenton oxidation. Therefore, we started our study by systematic screening of diverse trifluoromethylating agents and conditions in analogy to literature (usually C-H trifluoromethylations of other heterocycles)6,7 in the presence or in the absence of Cu(I) salts in order to see whether the trifluoromethylation of dimethyluracil (1) proceeds and what is the regioselectivity if formation of either 5- (2) or 6-(trifluoromethyl)-1,3-dimethyluracil (3) (Scheme 1).
The study started by screening of electrophilic trifluoromethylating agents. The Umemoto's reagent (S-(trifluoromethyl)dibenzothiophenium trifluoroborate)7a was used in combination with Pd(OAc)2 and/or CuI or Cu(OAc)2 in the presence or absence of P(C6F5)3 ligand and/or Cs2CO3 or CsF base in DMF or DCE at 160 °C. No trifluoromethylation reaction was observed under any of these conditions. Only in the reaction in the presence of Cu(OAc)2 and TFA, formation of unexpected 5,5- (4) and 5-6-dimers (5) of 1,3-dimethyluracil was observed (Scheme 2). This confirms that the C-H activation at positions 5 and 6 is indeed possible but the oxidative dimerization of the heterocycle is preferred over trifluoromethylation. The Togni's reagent (3,3-dimethyl-1-(trifluoromethyl)-1,2-benziodoxole)6c was also tried in the presence of CuOAc, CuTC, Pd(OAc)2 or AuCl with P(C6F5)3 or phenanthroline and Cs2CO3 or LiOH.H2O, but no lation was observed either.
Next, we tested selected nucleophilic trifluoromethylating agents themselves or after generation of radicals. Rupert's reagent (CF3SiMe3)7a,b was used in a series of experiments in reaction with 1 in the presence of Pd(OAc)2, PhI(OAc)2, TEMPO and CsF or in the presence of Cu(OAc)2, phenanthroline, (CH3)3COOC(CH3)3, (t-BuO)2 and NaOt-Bu but no trifluoromethylation was observed. Only, a reaction of 1 with CF3SiMe3 in the presence of Cu(OAc)2, phenanthroline, Ag2CO3 and KF gave the desired 1,3-dimethyl-5-trifluoromethyluracil (2) in moderate yield of 27%. The reaction of 1 with CF3SO2Na in the presence of CuI and Cs2CO3 did not work. However, the same reagent under radical conditions in the presence of t-BuOOH at rt (analogy to ref.10) gave the 5-trifluoromethylated uracil 2 in good yield of 67% (Scheme 3, X-ray structure in Figure 1). No formation of 6-(trifluoromethyl)uracil (3) was observed in any of the direct C-H activations.
Another possible way to trifluoromethylated heterocycles is based on Ir-catalyzed C-H borylation followed by electrophilic trifluoromethylation.11 Therefore, we have tried to perform the C-H borylation of 1 with bis(pinacolato)diboron under [{Ir(cod)OMe}2] + di-tert-butylbipyridine (dtbpy) catalysis in THF. This reaction led to an unseparable mixture of starting compound 1, 5-pinacolatoboryl- (6) and 5,6-bis(pinacolatoboryl)uracil (7) in ca. 2:5:3 ratio. Therefore, the whole reaction mixture was only evaporated and directly used in the second step in the reaction with the Togni's reagent, in the presence of CuTC, phenanthroline and LiOH·H2O in air. The two-step sequence then gave 1,3-dimethyl-5-(trifluoromethyl)uracil (2) in 21% and 6-1,3-dimethyl-6-(trifluoromethyl)uracil (3) in 8%. Any attempted optimization did not improve the yields of the final trifluoromethylated uracils. Since no 6-(pinacolatoboryl)uracil was observed in the reaction mixture after the borylation, the formation of 3 apparently must have resulted from trifluoromethylation (at position 6) and proto-deborylation (at position 5) of diborylated uracil 7. Altough, no method examined in this paper gave higher yield than the previously reported MacMillan radical trifluoromethylation,8a the synthesis of 1,3-dimethyl-5-(trifluoromethyl)uracil (2) by the Baran protocol is also very efficient and it was used for larger scale synthesis of this compound. On the other hand, the borylation/trifluoromethylation sequence gave for the first time a trifluoromethylation at position 6 (though in low yield).
Having sufficient amount of 1,3-dimethyl-5-(trifluoromethyl)uracil (2) in hand, we set up a series of Pd-catalyzed reactions with 4-iodotoluene to explore the possibility of further C-H arylation at position 6 (Scheme 5, Table 1). At first, the conditions from previously reported C-H arylations of 112 were attempted. The reaction in the presence of Pd(OAc)2, P(C6F5)3, CuI and Cs2CO3 did not give even a trace amounts of the desired product 8. Surprisingly, it gave only a mixture of 6-tolyl-1,3-dimethyluracil (9) and 1,3-dimethyluracil (1) where both products lost the trifluoromethyl group at position 5 (entry 1). The same reaction in the absence of CuI gave the same products in lower yields accompanied by unexpected phenanthrene-fused uracil 10, as a result of double arylation at positions 5 and 6 followed by oxidative C-H coupling (entry 2). Also the reaction in presence of pivalic acid and K2CO3 (analogy to ref. 14) led to formation of de-trifluoromethylated products 9 and 1 (entry 3).
However, when CsF was used as a base in the presence of P(C6F5)3 and CuI, the formation of the desired 5-(trifluoromethyl)-6-tolyluracil 8 (for X-ray structure, see Figure 1) was observed in very low yield and no CF3 group cleavage was observed (entry 4). The same reaction in the absence of CuI gave the fused product 10 in low yield (entry 5), whereas the presence of PivOH led to low yield of the desired 8 (entry 6). The reaction in the presence of piperidine as a base did not proceed (entry 7), while the use of DBU gave low yield of 8 again (entry 8). Some improvement of the yield was achieved by the use of (t-Bu)2PMe·HBF4 as ligand precursor to give product 8 in 15%. The best conversion was achieved in the reaction in the presence of CuI and CsF in the absence of any ligand. Under these conditions, desired product 8 was isolated in 25% yield (entry 10). The use of copper thiophene-2-carboxylate (CuTC) did not lead to improvement of the yield (entry 11), whereas the absence of any Cu(I) salt led to formation of 10 (entry 12). Prolongation of reaction time, use of microwave irradiation, various temperatures or change of solvent (DMA) did not lead to any improvement of the reactivity. Attempted applications of the best conditions (from entry 10) to the analogous reactions of 2 with iodobenzene or 4-iodoanisole did not lead to any detectable amounts of 6-arylated products so, apparently, the reaction is not general and C-H arylations with other aryl halides would require complete re-optimization of the conditions. Also attempted C-H trifluoromethylation (with CF3SO2Na in the presence of tBuOOH) or C-H borylation (with bis(pinacolato)diboron under [{Ir(cod)OMe}2] + di-tert-butylbipyridine) of 1,3-dimethyl-6-tolyluracil (6) or isomeric 1,3-dimethyl-5-tolyluracil did not lead to any 5,6-disubstituted uracil products.
Since the C-H arylation reactions in presence of Cs2CO3 led to loss of the CF3 group, it was interesting to look into possible mechanism of this C-C bond cleavage. Therefore a model reaction in the absence of aryl halide and catalyst was performed. Thus, 1,3-dimethyl-5-(trifluoromethyl)pyrimidine-2,4(1H,3H)-dione (2) was treated with Cs2CO3 in DMF at 160 °C. When the reaction was stopped after 3 h using acid work up, formation of 1,3-dimethyluracil-5-carboxylic acid 11 was observed, whereas, after prolonged reaction time, dimethyluracil 1 was observed as the major product (by NMR of the crude mixture). To verify whether the transformation of CF3 to CO2H is a substitution or a "hydrolysis", the same reaction was performed with K213CO3 which showed no 13C enrichment of product 11 confirming the CF3 "hydrolysis" hypothesis.
CONCLUSIONS
In conclusion, C-H trifluoromethylation of 1,3-dimethyluracil (1) was systematically studied. While attempted electrophilic trifluoromethylations led to uracil dimers and nucleophilic trifluoromethylations did not work or gave low conversions, radical trifluoromethylation with CF3SO2Na in the presence of tBuOOH (analogy to ref.10) gave 1,3-dimethyl-5-(trifluoromethyl)uracil (2) in good yield. C-H borylation of 1 gave an unseparable mixture of mono- and diborylated products which upon reaction with the Togni's reagent gave separable mixture of 2 and 1,3-dimethyl-6-(trifluoromethyl)uracil (3). Attempted C-H arylation of 2 in presence of Cs2CO3 was difficult due to electron withdrawing effect of the CF3 group on the pyrimidine ring and was also accompanied by cleavage of the CF3 group due to hydrolysis followed by decarboxylation. C-H arylations of 2 with tolyl iodide in the presence of a Cu(I) salt and CsF gave the desired 6-aryl-5-CF3-uracil 8 in moderate yields only and the reaction did not work for other aryl halides. This study shows possible severe limitations of further derivatizations and reactivity of trifluoromethyl-derivatives of heterocycles which can undergo number of side-reactions resulting from reactivity and instability of the CF3 group.
EXPERIMENTAL
1,3-Dimethyluracil (1) and all reagents were purchased from commercial suppliers and used without any further treatment. Dichloromethane, dry DMF, THF and dichloroethane were used as received from supplier. Cs2CO3 and CsF are extremely hygroscopic and must be kept away from moisture, therefore were dried at 500 °C under vacuo for 15 minutes prior to each use. All reactions were carried out in flame-dried glassware with magnetic stirring. All compounds were fully characterized by NMR spectroscopy and spectra were recorded on a Bruker Avance II 600 (1H at 600.1 MHz, 13C at 150.9 MHz) or on a Bruker Avance II 500 (499.8 or 500.0 MHz for 1H, 125.7 MHz for 13C and 470.3 MHz for 19F) spectrometers. The samples were measured in CDCl3 and chemical shifts (in ppm, δ-scale) were referenced to solvent signal for 1H and 13C (δ(1H) = 7.26 ppm, δ(13C) = 77.0 ppm) and to external standard C6F6 (-163 ppm) for 19F (1H decoupling), or in D2O solutions with 1,4-dioxane as internal standard (δ(1H) = 3.75 ppm, δ(13C) = 69.3 ppm). Coupling constants (J) are given in Hz. 1H and 13C resonances were assigned using H,C-HSQC and H,C-HMBC spectra. IR spectra (wavenumbers in cm-1) were recorded on a Bruker Alpha FT-IR spectrometer using ATR technique. Melting points were determined on a Kofler block and are uncorrected. High resolution mass spectra were measured on a LTQ Orbitrap XL (Thermo Fisher Scientific) spectrometer using EI or ESI ionization technique. X-ray diffraction experiment of single crystals was carried out on an Xcalibur X-ray diffractometr with CuKα (λ=1.54180 Å) at 190 K.
1,3-Dimethyl-5-(trifluoromethyl)pyrimidine-2,4(1H,3H)-dione (2)
To a solution of 1.3-dimethyluracil (1) (70.1 mg, 0.5 mmol, 1.0 equiv) and sodium trifluoromethylsulfinate (234 mg, 1.5 mmol, 3.0 equiv) in CH2Cl2 (2.0 mL) and water (0.8 mL) at 0 ºC was slowly added tert-butylhydroperoxide (70% solution in water, 0.34 mL, 2.5 mmol, 5.0 equiv) with vigorous stirring under an air atmosphere. The reaction was allowed to warm to room temperature and monitored by thin layer chromatography (hexanes / EtOAc 1:1) until completion. Upon consumption of starting material (4 h), the reaction was partitioned between CH2Cl2 (4.0 mL) and saturated sodium bicarbonate (4.0 mL). The organic layer was separated, and the aqueous layer was extracted with CH2Cl2 (3 x 4.0 mL). The organic layers were dried with magnesium sulfate, concentrated, and isolated by column chromatography on 50 g of silica gel in eluent hexanes / EtOAc 8:2, in 67% yield, as a white crystals from hexanes / EtOAc, mp 100-102 °C (lit15 mp 101-102 °C). 1H NMR (499.8 MHz, CDCl3): 3.37 (s, 3H, CH3-3); 3.49 (s, 3H, CH3-1); 7.67 (q, 1H, JH,F = 1.1, H-6). 13C NMR (125.7 MHz, CDCl3): 28.03 (CH3-3); 37.77 (CH3-1); 104.17 (q, JC,F = 33.3, C-5); 121.95 (q, JC,F = 269.8, CF3); 143.45 (q, JC,F = 5.8, CH-6); 150.90 (C-2); 158.65 (C-4). 19F{1H} NMR (470.3 MHz, CDCl3): -59.99. IR: 1727, 1665, 1648, 1501, 1462, 1391, 1360, 1329, 1215, 1119, 1074, 1021. MS (EI+), m/z (% relative intensity): 42 (43), 56 (10), 60 (8), 75 (10), 91 (7), 103 (5), 123 (31), 132 (13), 150 (33), 160 (10), 179 (4), 188 (34), 208 (M+, 100). HR MS (M+): 208.0462 (calcd for C7H7N2O2F3 208.0460).
1,3-Dimethyl-6-(trifluoromethyl)pyrimidine-2,4(1H,3H)-dione (3)
1.3-Dimethyluracil (1) (70.1 mg, 0.5 mmol), [Ir(cod)(OMe)]2 (1.7 mg, 0.5 mol%), dtbpy (1.4 mg, 1.0 mol%), and B2pin2 (101.6 mg, 0.4 mmol) were placed into an oven-dried sealed vial. The vial was evacuated and refilled with argon three times. Under flow of argon, dry THF (1.0 mL) was added. The reaction mixture was stirred at 80 °C for 24 h. After cooling to rt, the reaction mixture was diluted with CHCl3 and the volatiles were removed under vacuum. To the residue was added CuTc (9.5 mg, 0.05 mmol), 1,10-phenanthroline (18.0 mg, 0.10 mmol), LiOH·H2O (42.0 mg, 1.0 mmol), Togni’s reagent (181.5 mg, 0.55 mmol) and 2.0 mL of CH2Cl2. The reaction mixture was refluxed under an air atmosphere at 50 °C for 22 h. After cooling to rt, the solvent was evaporated under reduced pressure and products were isolated by column chromatography on silica gel (70 g) in eluent hexanes / EtOAc 8:2. It gave compound 2 (21.8 mg, 21%) and compound 3 (8.4 mg, 8%). Compound 3: white powder, mp 84-86 °C (lit16 mp 85-87 °C). 1H NMR (499.8 MHz, CDCl3): 3.37 (s, 3H, CH3-3); 3.52 (q, 3H, JH,F = 1.3 CH3-1); 6.25 (s, 1H, H-5). 13C NMR (125.7 MHz, CDCl3): 28.48 (CH3-3); 32.48 (q, JC,F = 3.6, CH3-1); 102.63 (q, JC,F = 5.6, CH-5); 119.45 (q, JC,F = 275.1, CF3); 140.51 (q, JC,F = 34.3, C-6); 151.66 (C-2); 161.05 (C-4). 19F{1H} NMR (470.3 MHz, CDCl3): -62.05. IR: 1721, 1684, 1661, 1637, 1498, 1444, 1411, 1377, 1274, 1219, 1178, 1163, 1128, 1077, 1024. MS (EI+), m/z (% relative intensity): 44 (12), 82 (100), 115 (9), 141 (20), 151 (12), 208 (M+, 51). HR MS (M+): 208.0465 (calcd for C7H7N2O2F3 208.0460).
1,1',3,3'-Tetramethyl-[5,5'-bipyrimidine]-2,2',4,4'(1H,1'H,3H,3'H)-tetraone (4) and 1,1',3,3'-tetramethyl-[4,5'-bipyrimidine]-2,2',4',6(1H,1'H,3H,3'H)-tetraone (5)
In a 5 mL sealed tube, 1.3-dimethyluracil (1) (70.1 mg, 0.5 mmol, 1.0 equiv), Pd(OAc)2 (11.2 mg, 0.05 mmol, 0.1 equiv), Cu(OAc)2 (90.8 mg, 0.5 mmol, 1.0 equiv) and 5-(trifluoromethyl)-dibenzothiophenium tetrafluoroborate (255.1 mg, 0.75 mmol, 1.5 equiv) were dissolved in 2.5 mL dry CH2Cl2 under air, and then trifluoroacetic acid (371.5 μL, 5.0 mmol, 10 equiv) was added. The tube was sealed with a cap and the reaction mixture was stirred at 100 °C for 48 h. After cooling to rt, the volatiles were removed under vacuum and the residue was purified by column chromatography on 40 g of silica gel in gradient 10% of hexanes in EtOAc to 100% of EtOAc to afford compound 4 (22.8 mg, 16%) and compound 5 (9.5 mg, 7%). Compound 4: yellowish powder, identical spectroscopic data to those previously described.17 1H NMR (499.8 MHz, CDCl3): 3.40 (s, 6H, CH3-3); 3.48 (s, 6H, CH3-1); 8.41 (s, 2H, H-6). 13C NMR (125.7 MHz, CDCl3): 28.33 (CH3-3); 37.58 (CH3-1); 104.03 (C-5); 142.96 (CH-6); 150.68 (C-2); 162.58 (C-4). IR: 1696, 1647, 1442, 1380, 1344, 1314, 1220, 1192, 1130, 1112, 1006. MS (EI+), m/z (% relative intensity): 43 (47), 55 (87), 69 (61), 83 (73), 97 (77), 111 (51), 125 (29), 139 (15), 149 (43), 167 (13), 180 (26), 193 (57), 221 (10), 252 (6), 278 (M+, 100). HR MS (M+): 278.1013 (calcd for C12H14N4O4 278.1015). Compound 5: yellowish powder, identical spectroscopic data to those previously described.17 1H NMR (499.8 MHz, CDCl3): 3.29 (s, 3H, CH3-1B); 3.37 (s, 3H, CH3-3B); 3.40 (s, 3H, CH3-3A); 3.50 (s, 3H, CH3-1A); 5.65 (s,1H, H-5B); 7.40 (s, 2H, H-6A). 13C NMR (125.7 MHz, CDCl3): 28.09 (CH3-3B); 28.40 (CH3-3A); 33.57 (CH3-1B); 37.60 (CH3-1A); 103.71 (CH-5B); 108.31 (C-5A); 143.08 (CH-6A); 147.56 (C-6B); 151.00 (C-2A); 152.14 (C-2B); 160.34 (C-4A); 162.33 (C-4B). IR: 1707, 1649, 1607, 1440, 1402, 1379, 1350, 1189, 1102, 1083, 1011. MS (ESI+), m/z (% relative intensity): 279 (M++H, 100), 579 (2M++Na, 11). HR MS (M++H): 279.10882 (calcd for C12H15O4N4 279.10878).
1,3-Dimethyl-6-(p-tolyl)-5-(trifluoromethyl)pyrimidine-2,4(1H,3H)-dione (8)
Dry DMF (2 mL) was added through a septum to an argon purged vial containing a 1,3-dimethyl-5-(trifluoromethyl)pyrimidine-2,4(1H,3H)-dione (2) (62.4 mg, 0.3 mmol), 4-iodotoluene (130.8 mg, 0.6 mmol), Pd(OAc)2 (3.4 mg, 0.015 mmol, 5 mol %), CuI (171.4 mg, 0.9 mmol) and CsF (113.9 mg, 0.75 mmol). Reaction mixture was stirred at 160 °C for 48 h. After cooling to rt, the mixture was diluted with CHCl3 (20 mL) and solvents were evaporated under reduced pressure. Products were isolated by column chromatography on 40 g of silica gel in eluent hexanes / EtOAc 8:2. Compound 8 (22.5 mg, 25%) was obtained as a white crystals by recrystallization from hexanes / EtOAc, mp 191-193 °C. 1H NMR (499.8 MHz, CDCl3): 2.24 (s, 3H, CH3-C6H4); 3.05 (s, 3H, CH3-1); 3.44 (s, 3H, CH3-3); 7.11 (m, 2H, H-o-C6H4Me); 7.32 (m, 2H, H-m-C6H4Me). 13C NMR (125.7 MHz, CDCl3): 21.43 (CH3-C6H4); 28.39 (CH3-3); 34.47 (CH3-1); 103.94 (q, JC,F = 30.3, C-5); 122.42 (q, JC,F = 272.2, CF3); 126.77 (q, JC,F = 1.3, CH-o-C6H4Me); 128.33 (C-i-C6H4Me); 129.78 (CH-m-C6H4Me); 140.58 (C-p-C6H4Me); 151.12 (C-2); 155.95 (C-6); 158.82 (C-4). 19F{1H} NMR (470.3 MHz, CDCl3): -51.84. IR: 1721, 1663, 1623, 1609, 1520, 1455, 1433, 1405, 1370, 1334, 1152, 1114, 1058, 1017. MS (ESI+), m/z (% relative intensity): 299 (M++H, 10), 321 (M++Na, 100). HR MS (M++Na): 321.08211 (calcd for C14H13O2N2F3Na 321.08213).
1,3-Dimethyluracil (1), 1,3-dimethyl-6-p-tolylpyrimidine-2,4(1H,3H)-dione (9) and 1,3,7,10-tetramethyldibenzo[f,h]quinazoline-2,4(1H,3H)-dione (10)
Dry DMF (2 mL) was added through a septum to an argon purged vial containing a 1,3-dimethyl-5-(trifluoromethyl)pyrimidine-2,4(1H,3H)-dione (2) (62.4 mg, 0.3 mmol), 4-iodotoluene (130.8 mg, 0.6 mmol), Pd(OAc)2 (3.4 mg, 0.015 mmol, 5 mol %), P(C6F5)3 (16.0 mg, 0.03 mmol, 10 mol %) and Cs2CO3 (244.4 mg, 0.75 mmol). Reaction mixture was stirred at 160 °C for 24 h. After cooling to rt, the mixture was diluted with CHCl3 (20 mL) and solvents were evaporated under reduced pressure. Products were isolated by column chromatography on 40 g of silica gel in gradient 10% of hexanes in EtOAc to 100% EtOAc. It gave compound 1 (12.4 mg, 30%), compound 9 (17.3 mg, 25%) and compound 10 (10.2 mg, 11%). Compound 1: white crystals from EtOAc, identical spectroscopic data to authentic commercial sample. Compound 9: white crystals from hexanes / EtOAc, identical spectroscopic data to those previously described.12a Compound 10: white powder, 1H NMR (600.1 MHz, CDCl3): 2.61 (s, 3H, CH3-7); 2.64 (s, 3H, CH3-10); 3.55 (s, 3H, CH3-3); 3.80 (s, 3H, CH3-1); 7.42 (ddq, 1H, J11,12 = 8.5, J11,9 = 1.7, J11,CH3 = 0.5, H-11); 7.53 (ddq, 1H, J6,5 = 8.5, J6,8 = 1.7, J6,CH3 = 0.5, H-6); 8.05 (d, 1H, J12,11 = 8.5, H-12); 8.38 (dq, 1H, J8,6 = 1.7, J8,CH3 = 0.7, H-8); 8.45 (dq, 1H, J9,11 = 1.7, J9,CH3 = 0.7, H-9); 9.68 (d, 1H, J5,6 = 8.5, H-5). 13C NMR (150.9 MHz, CDCl3): 21.74 (CH3-7); 22.05 (CH3-10); 28.48 (CH3-3); 41.73 (CH3-1); 108.76 (C-4a); 121.05 (C-12a); 122.10 (CH-8); 123.72 (CH-9); 126.38 (CH-5); 126.43 (CH-12); 126.46 (C-4b); 127.37 (CH-11); 127.94 (C-8a); 130.26 (CH-6); 134.33 (C-8b); 135.98 (C-7); 140.05 (C-10); 14.31 (C-12b); 153.31 (C-2); 162.48 (C-4). IR: 1724, 1692, 1653, 1621, 1587, 1514, 1443, 1369, 1317, 1295, 1264, 1233, 1192, 1168, 1111, 1011. MS (ESI+), m/z (% relative intensity): 319 (M++H, 8), 341 (M++Na, 100), 359 (2M++Na, 17). HR MS (M++Na): 341.12606 (calcd for C20H18O2N2Na 341.12605).
1,3-Dimethyl-2,4-dioxo-1,2,3,4-tetrahydropyrimidine-5-carboxylic acid (11)
Dry DMF (1 mL) was added through a septum to an argon purged vial containing a 1,3-dimethyl-5-(trifluoromethyl)pyrimidine-2,4(1H,3H)-dione (2) (25 mg, 0.12 mmol, 1.0 equiv) and Cs2CO3 (98 mg, 0.30 mmol, 2.5 equiv). The reaction mixture was heated at 160 °C until the reaction was complete (3 h). After cooling to rt, DMF was evaporated under reduced pressure. The residue was dissolved in H2O and acidified by HCl forming precipitate. The product was isolated by filtration as a white powder, mp 185-187 °C (lit15 mp 188-189 °C). 1H NMR (499.8 MHz, D2O): 3.34 (s, 3H, CH3-3); 3.54 (s, 3H, CH3-1); 8.65 (s, 1H, H-6). 13C NMR (125.7 MHz, D2O): 30.84 (CH3-3); 40.84 (CH3-1); 103.49 (C-5); 154.65 (C-2); 155.88 (CH-6); 167.68 (C-4); 169.22 (COOH). IR: 1745, 1720, 1634, 1529, 1495, 1459, 1425, 1404, 1367, 1339, 1208, 1156, 1077, 1033, 1004. MS (ESI+), m/z (% relative intensity): 185 (M++H, 100), 207 (M++Na, 27), 391 (2M++Na, 14). HR MS (M++Na): 207.03768 (calcd for C7H8O4N2Na 207.03763).
Single crystal X-ray structure analysis
The diffraction data of single crystals of 2 (colorless, 0.27 x 0.32 x 0.78 mm) and 8 (0.07x 0.61x 0.79 mm) were collected on Xcalibur X-ray diffractometr with CuKα (λ=1.54180 Å) at 190 K. The structures were solved by direct methods with SIR9218 and by charge flipping with SUPERFLIP19 and refined by full-matrix least-squares on F with CRYSTALS.20 All hydrogen atoms were located in a difference map but later were repositioned geometrically and then refined with riding constraints, while all other atoms were refined anisotropically in both cases.
Crystal data for 2: C7H7F3N2O2, orthorhombic, space group P212121, a = 6.19806(12) Å, b = 10.53566(18) Å, c = 13.2460(2) Å, V = 864.97(3) Å3, Z = 4, M = 208.14, 3031 reflections measured, 1759 independent reflections. Final R = 0.046, wR = 0.051, GoF = 1.110 for 1730 reflections with I > 2σ(I) and 129 parameters, Flack parameter x = 0.0(2). CCDC 945178.
Crystal data for 8: C14H13F3N2O2, monoclinic, space group P21/n, a = 8.2089(5) Å, b = 12.4775(6) Å, c = 13.4481(7) Å, β = 97.690(5)°, V = 1365.05(12) Å3, Z = 4, M = 298.26, 8971 reflections measured, 2770 independent reflections. Final R = 0.131, wR = 0.141, GoF = 0.876 for 2590 reflections with I > 2σ(I) and 191 parameters. CCDC 945179. Unfortunately, the crystals of 8 were of rather poor quality and did not diffract strongly, which led to higher R factor value. The precision of the structure determination is therefore not excellent, but it still describes reasonably well all the main structural features of 8.
ACKNOWLEDGEMENTS
This work was supported by the institutional support of the Charles University and Academy of Sciences of the Czech Republic (RVO: 61388963), by the Czech Science Foundation (P207/12/0205) and by Gilead Sciences, Inc.
References
1. (a) C. Heidelberger, D. G. Parsons, and D. C. Remy, J. Med. Chem., 1964, 7, 1; CrossRef (b) B. L. Wigdahl and J. R. Parkhurst, Antimicrob. Agents Chemother., 1978, 14 , 470; CrossRef (c) R. Salvetti, A. Marchand, M. Pregnolato, A. Verri, S. Spadari, F. Focher, M. Briant, J.-P. Sommadossi, C. Mathé, and G. Gosselin, Bioorg. Med. Chem., 2001, 9, 1731. CrossRef
2. (a) A. Giner-Sorolla and A. Bendich, J. Am. Chem. Soc., 1958, 80, 5744; CrossRef (b) C. Kaiser and A. Burger, J. Org. Chem., 1959, 24, 113; CrossRef (c) A. V. Popov, A. N. Pushin, and E. L. Luzina, Russ. Chem. Bull., 1998, 47, 1232; CrossRef (d) A. Sidduri, J. W. Tilley, J. Lou, N. Tare, G. Cavallo, K. Frank, A. Pamidimukkala, D. S. Choi, L. Gerber, A. Railkar, and L. Renzetti, Bioorg. Med. Chem. Lett., 2013, 23, 1026. CrossRef
3. (a) Y. Kobayashi, K. Yamamoto, T. Asai, M. Nakano, and I. Kumadaki, J. Chem. Soc., Perkin Trans. 1, 1980, 2755; CrossRef (b) I. Nowak and M. J. Robins, J. Org. Chem., 2007, 72, 2678; CrossRef (c) L. Strekowski, M. A. Ismail, and H. H. Zoorob, Heterocycl. Commun., 1998, 4, 399; (d) H. Morimoto, T. Tsubogo, N. D. Litvinas, and J. F. Hartwig, Angew. Chem. Int. Ed., 2011, 50, 3793. CrossRef
4. N.-A. Meanwell, J. Med. Chem., 2011, 54, 2529; CrossRef (b) T. Furuya, A.-S. Kamlet, and T. Ritter, Nature, 2011, 473, 470. CrossRef
5. Reviews: (a) J.-A. Ma and D. Cahard, J. Fluorine. Chem., 2007, 128, 975; CrossRef (b) O. A. Tomashenko and V. V. Grushin, Chem. Rev., 2011, 111, 4475; CrossRef Examples: (c) E. J. Cho, T. D. Senecal, T. Kinzel, Y. Zhang, D. A. Watson, and S. L. Buchwald, Science, 2010, 328, 1679; CrossRef (d) C.-P. Zhang, Z.-L. Wang, Q.-Y. Chen, C.-T. Zhang, Y.-C. Gu, and J.-C. Xiao, Angew. Chem. Int. Ed., 2011, 50, 1896; CrossRef (e) T. Knauber, F. Arikan, G.-V. Röschenthaler, and L. J. Gooßen, Chem. Eur. J., 2011, 17, 2689; CrossRef (f) M. Oishi, H. Kondo, and H. Amii, Chem. Commun., 2009, 1909.
6. (a) X. Wang, L. Truesdale, and J.-Q. Yu, J. Am. Chem. Soc., 2010, 132, 3648; CrossRef (b) R. N. Loy and M. S. Sanford, Org. Lett., 2011, 13, 2548; CrossRef (c) R. Shimizu, H. Egami, T. Nagi, J. Chae, Y. Hamashima, and M. Sodeoka, Tetrahedron Lett., 2010, 51, 5947; CrossRef (d) M. S. Wiehn, E. V. Vinogradova, and A. Togni, J. Fluorine Chem., 2010, 131, 951. CrossRef
7. (a) X. Mu, S. Chen, X. Zhen, and G. Liu, Chem. Eur. J., 2011, 17, 6039; CrossRef (b) L. Chu and F.-L. Qing, J. Am. Chem. Soc., 2012, 134, 1298; CrossRef (c) Y. Ye, S. H. Lee, and M. S. Sanford, Org. Lett., 2011, 13, 5464. CrossRef
8. (a) D. A. Nagib and D. W. C. MacMillan, Nature, 2011, 480, 224; CrossRef (b) N. Iqbal, S. Choi, E. Ko, and E. J. Cho, Tetrahedron Lett., 2012, 53, 2005. CrossRef
9. (a) D. Uraguchi, K. Yamamoto, Y. Ohtsuka, K. Kokuhisa, and T. Yamakawa, Appl. Catal. A. General, 2008, 342, 137; CrossRef (b) T. Kino, Y. Nagase, Y. Ohtsuka, K. Yamamoto, D. Uraguchi, K. Tokuhisa, and T. Yamakawa, J. Fluorine Chem., 2010, 131, 98. CrossRef
10. Y. Ji, T. Brueckl, R. D. Baxter, Y. Fujiwara, I. B. Seiple, S. Su, D. G. Blackmond, and P. S. Baran, Proc. Natl. Acad. Sci. USA, 2011, 108, 14411. CrossRef
11. T. Liu, X. Shao, Y. Wu, and Q. Shen, Angew. Chem. Int. Ed., 2012, 51, 540. CrossRef
12. (a) M. Čerňová, R. Pohl, and M. Hocek, Eur. J. Org. Chem., 2009, 3698; CrossRef (b) M. Čerňová, I. Čerňa, R. Pohl, and M. Hocek, J. Org. Chem., 2011, 76, 5309. CrossRef
13. (a) K. H. Kim, H. S. Lee, and J. N. Kim, Tetrahedron Lett., 2011, 52, 6228; CrossRef (b) H. S. Lee, K. H. Kim, S. H. Kim, and J. N. Kim, Tetrahedron Lett., 2012, 53, 497; CrossRef (c) C. Cheng, Y.-C. Shih, H.-T. Chen, and T.-C. Chien, Tetrahedron, 2013, 69, 1378; CrossRef (d) Y.-Y. Yu and G. I. Georg, Chem. Commun., 2013, 49, 3694. CrossRef
14. Y.-N. Wang, X.-Q Guo, Z.-H. Zhu, R. Zhong, L.-H. Cai, and X.-F. Hou, Chem. Commun., 2012, 48, 10437. CrossRef
15. D. V. Santi and T. T. Sakai, Biochemistry, 1971, 10, 3598. CrossRef
16. A. Sidduri and J. W. Tilley, Hoffmann-La Roche Inc. Patent: US6380387 B1, 2002.
17. K. H. Kim, H. S. Lee, S. H. Kim, and J. N. Kim, Tetrahedron Lett., 2012, 53, 1323. CrossRef
18. A. Altomare, G. Cascarano, G. Giacovazzo A. Guagliardi, M. C. Burla, G. Polidori, and M. Camalli, J. Appl. Cryst., 1994, 27, 435. CrossRef
19. L. Palatinus and G. Chapuis, J. Appl. Cryst., 2007, 40, 786. CrossRef
20. P. W. Betteridge, J. R. Carruthers, R. I. Cooper, K. Prout, and D. J. Watkin, J. Appl. Cryst., 2003, 36, 1487. CrossRef