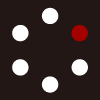
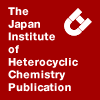
HETEROCYCLES
An International Journal for Reviews and Communications in Heterocyclic ChemistryWeb Edition ISSN: 1881-0942
Published online by The Japan Institute of Heterocyclic Chemistry
e-Journal
Full Text HTML
Received, 18th February, 2014, Accepted, 26th March, 2014, Published online, 2nd April, 2014.
DOI: 10.3987/COM-14-12965
■ One-Pot Three-Component Synthesis of 3,5-Disubstituted Isoxazoles by a Coupling–Cyclocondensation Sequence
Hai-Ling Liu,* Zhu-Feng Geng, Si-Yu Zhang, and Jie Han
Analytical & Testing Center, Beijing Normal University, No. 19, XinJieKouWai St., HaiDian District 100875, China
Abstract
A convenient one-pot procedure for the synthesis of 3,5-disubstituted isoxazoles from acid chloride, terminal alkyne, and hydroxylamine hydrochloride catalyzed by Pd(PPh3)2Cl2/CuI has been developed. The coupling of acid chlorides to terminal alkynes afforded α,β-unsaturated ynones that underwent in situ cyclocondensation with hydroxylamines to afford the desired isoxazoles in 44–76% isolated yields.A tandem reaction refers to two consecutive reactions in the same reaction vessel.1 Tandem one-pot reactions are powerful tools to construct important cyclic compounds2 such as pyrroles3 pyrimidines,4 furans,5 and triazoles.6 Recently, we reported the tandem reactions for the synthesis of polysubstituted tetrahydropyrimidines7 and pyrazoles.8 Encouraged by the results, we investigated similar tandem reactions for other N-containing heterocycles.
Isoxazoles are important five-membered heterocyclic compounds bearing both nitrogen and oxygen atoms and found in diverse natural products.9 They possess important biological and pharmaceutical activities10 and useful building blocks in organic synthesis.11 Therefore, the development of new methods for their synthesis is of great interest.12 In general, isoxazoles are prepared by (i) the reaction of 1,3-dicarbonyl compounds with hydroxylamine,13 (ii) the 1,3-dipolar cycloaddition of alkenes and alkynes with nitrile oxides,14 (iii) the reaction of hydroxylamines with α,β-unsaturated ketones,15 and other methods.16 One-pot syntheses of isoxazoles from in situ generated intermediates such as nitrile oxides,17 propargylic N-hydroxylamines,18 oximes,19 oxime ether20 and ynones21 have been reported. Unfortunately, certain reagents used in these cases are toxic, air sensitive, difficult to obtain, and hazardous.
The one-pot synthesis of isoxazoles from inexpensive, safe, and easily available starting materials would be more facile and efficient. Several methods for the synthesis of ynones by the coupling of acid chlorides to terminal alkynes are known.22 Herein, we report the one-pot synthesis of 3,5-disubstituted isoxazoles by the cyclocondensation of hydroxylamines with ynones generated in situ from acid chlorides and terminal alkynes.
First, the reaction of benzoyl chloride 1a with phenylacetylene 2a and hydroxylamine hydrochloride (Table 1) was investigated. When Na2CO3 as the base and acetonitrile (MeCN) as the cosolvent at the second step, the desired isoxazole 3aa was obtained in only 27% GC yield. The yield of 3aa could be improved by refluxing the reaction mixture (Table 1, entry 2, 68% yield). Different solvents were screened to improve the yield of the reaction with hydroxylamine hydrochloride. When water was used as the cosolvent, 3aa was obtained in 73% yield (Table 1, entry 3). When 1,4-dioxane was used as the cosolvent, 3aa was obtained in 80% yield (Table 1, entry 4). When no cosolvent was used, 3aa was obtained in 81% yield (Table 1, entry 5). Next, the effect of different bases was examined in the absence of a cosolvent. When triethylamine (Et3N) was used as the base, 3aa was obtained in 27% yield (Table 1, entry 6). When sodium acetate (NaOAc) was used as the base, 3aa was obtained in the highest yield (Table 1, entry 7, 87% yield). Thus, the reaction of 1a (1.5 mmol) with 2a (1.0 mmol) in the presence of 1 mol% Pd (PPh3)2Cl2, 3 mol% CuI, and 2 mmol Et3N for 2 h in THF (5 mL) under nitrogen atmosphere followed by the addition of hydroxylamine (2.0 mmol) and NaOAc (3.0 mmol) and reflux for 12 h afforded isoxazole 3aa in 87% yield.
The one-pot tandem reactions of different terminal alkynes 2 (2a and 2b) with benzoyl chloride 1a and hydroxylamine under the optimized reaction conditions were investigated (Table 2). The corresponding isoxazoles 3aa and 3ab were obtained in 66% and 70% isolated yields, respectively. Next, the reactions of diverse acid chlorides 1 with 2 and hydroxylamine were investigated. The corresponding isoxazoles 3ba to 3bb were obtained in 44–76% isolated yields.
The ynones were obtained via Sonogashira coupling of acid chloride and terminal alkyne.22,23 Subsequently, the ynones maybe take place 1,4-addition with hydroxylamine and then undergo dehydration-cyclization process to afford isoxazole. The possible reaction mechanism was listed in Scheme 1.
In summary, we developed a simple and efficient one-pot three-component protocol for the synthesis of 3,5-disubstituted isoxazoles directly from acid chlorides, terminal alkynes, and hydroxylamine hydrochloride catalyzed by Pd(PPh3)2Cl2/CuI system in moderate to good yields. Furthermore, the protocol compatible to various substrates and has easy work-up procedures, facilitating heterocycle synthesis.
EXPERIMENTAL
The NMR spectra were recorded using a Bruker Avance 400 MHz spectrometer. The infrared (IR) spectra were recorded using a Bruker Vector 22 spectrometer. The GC–MS analyses were performed using a Finnigan Trace DSQ spectrometer. All regents were commercially available.
A typical procedure for the preparation of compound 3. To a 25 mL round bottom flask, PdCl2 (PPh3)2 (0.01 mmol), CuI (0.03 mmol), Et3N (2.0 mmol), 1 (1.5 mmol) and 2 (1.0 mmol) were added in THF (5 mL) at room temperature for 2 h under N2. Then hydroxylamine hydrochloride (2.0 mmol) and NaOAc (3.0 mmol) were added and refluxed for 12 h. Then the reaction mixture was diluted with water (10 mL) and extracted with CH2Cl2 (2×20 mL). The combined organic layers were dried with sodium sulfate, concentrated to dryness and isolated by preparative TLC to obtain pure products 3.
3,5-Diphenylisoxazole (3aa).24 1H NMR (CDCl3, 400 MHz) δ: 6.80 (s, 1H), 7.42–7.51 (m, 6H), 7.80–7.86 (m, 4H). IR (KBr) ν: 2917, 1641, 1447, 1359, 1096 cm-1. MS (70 eV) m/z (%): 221 (M.+), 218, 193, 165, 144, 105, 89, 77, 51.
5-Phenyl-3-p-tolylisoxazole (3ab).24 1H NMR (CDCl3, 400 MHz) δ: 2.45 (s, 3H), 6.81 (s, 1H), 7.28–7.33 (m, 2H), 7.49-7.52 (m, 3H), 7.76 (d, J=8 Hz 2H), 7.88-7.91 (m, 2H). IR (KBr) ν: 2918, 2850, 1658, 1446, 1104 cm-1. MS (70 eV) m/z (%): (235, M.+), 207, 165, 144, 119, 105, 91, 77.
3-Phenyl-5-p-tolylisoxazole (3ba).24 1H NMR (CDCl3, 400 MHz) δ: 2.45 (s, 3H), 6.81 (s, 1H), 7.28–7.33 (m, 2H), 7.49-7.52 (m, 3H), 7.76 (d, J=8 Hz 2H), 7.88-7.91 (m, 2H). IR (KBr) ν: 3109, 2922, 1650, 1500, 1116 cm-1. MS (70 eV) m/z (%): 235 (M.+), 207, 165, 144, 119, 91, 65, 28.
5-(Furan-2-yl)-3-phenylisoxazole (3ca).1 1H NMR (CDCl3, 400 MHz) δ: 6.54 (dd, J1=3.2 Hz, J2=1.6 Hz, 1H), 6.67 (d, J=3.2 Hz 1H), 6.78 (s, 1H), 7.41–7.48 (m, 4H), 7.82–7.85 (m, 2H). IR (KBr) ν: 2923, 1653, 1501, 1356, 1112 cm-1. MS (70 eV) m/z (%): 211 (M.+), 183, 154, 144, 116, 95, 77, 51.
3-Phenyl-5-(thiophen-2-yl)isoxazole (3da).25 1H NMR (CDCl3, 400 MHz) δ: 6.68 (s, 1H), 7.13 (dd, J1=5.2 Hz, J2=4.0 Hz, 1H), 7.45–7.47 (m, 4H), 7.55 (dd, J1=3.6 Hz, J2=1.2 Hz, 1H), 7.82–7.84 (m, 2H). IR (KBr) ν: 3106, 2926, 2854, 1659, 1595, 1516, 1446, 1361, 1237 cm-1. MS (70 eV) m/z (%): 227 (M.+), 199, 186, 111 (100), 77, 51.
5-Cyclohexyl-3-phenylisoxazole (3ea).19 1H NMR (CDCl3, 400 MHz) δ: 1.28–1.55 (m, 6H), 1.84–1.88 (m, 2H), 2.11–2.15 (m, 2H), 2.82–2.85 (m, 1H), 6.27 (s, 1H), 7.44–7.48 (m, 3H), 7.81–7.83 (m, 2H). IR (KBr) ν: 3008, 2928, 1664, 1567, 1497, 1102 cm-1. MS (ESI) m/z: 228 (M+1).
3,5-Dip-tolylisoxazole (3bb).26 1H NMR (CDCl3, 400 MHz) δ: 2.44 (s, 6H), 6.78 (s, 1H), 7.29–7.32 (m, 4H), 7.74–7.79 (m, 8H). IR (KBr) ν: 3033, 2919, 1665, 1568, 1116 cm-1. MS (70 eV) m/z (%): 249 (M.+), 221, 208, 179, 158, 119, 103, 91, 65, 63.
ACKNOWLEDGEMENTS
The authors thank the Fundamental Research Funds for the Central Universities (No. 2013YB11) for financial support of this work.
References
1. Y. S. Chun, Z. Xuan, J. H. Kim, and S. G. Lee, Org. Lett., 2013, 15, 316; CrossRef Y. J. Feng, J. X. Lo, Y. C. Lin, S. L. Huang, Y. Wang, and Y. H. Liu, Organometallics, 2013, 32, 6379; CrossRef . CrossRef
2. W. Y. Zhao and F. E. Chen, Curr. Org. Synth., 2012, 9, 873; CrossRef X. M. Ren, W. Wan, H. Z. Jiang, and J. Hao, Mini-Rev. Org. Chem., 2007, 4, 330. CrossRef
3. S. D. Joshi, U. A. More, V. H. Kulkarni, and T. M. Aminabhavi, Curr. Org. Chem., 2013, 17, 2279. CrossRef
4. A. S. Karpov, E. Merkul, F. Rominger, and T. J. J. Müller, Angew. Chem. Int. Ed., 2005, 44, 6951. CrossRef
5. M. Zhang, H. F. Jiang, H. Neumann, M. Beller, and P. H. Dixneuf, Angew. Chem. Int. Ed., 2009, 48, 1681. CrossRef
6. J. H. Song, P. Choi, S. E. Lee, K. H. Jeong, T. Kim, K. S. Kang, Y. S. Choi, and J. Ham, Eur. J. Org. Chem., 2013, 6249. CrossRef
7. M. Zhang, H. F. Jiang, H. L. Liu, and Q. H. Zhu, Org. Lett., 2007, 9, 4111. CrossRef
8. H. L. Liu, H. F. Jiang, M. Zhang, W. J. Yao, Q. H. Zhu, and Z. Tang, Tetrahedron Lett., 2008, 49, 3805. CrossRef
9. Y. Yamashita, Y. Hirano, A. Takada, H. Takikawa, and K. Suzuki, Angew. Chem. Int. Ed., 2013, 52, 6658; CrossRef H. G. Cutter, Crit. Rev. Plant Sci., 1995, 14, 413. CrossRef
10. Y. L. Chen, C. H. Tseng, Y. C. Lo, R. W. Lin, C. F. Chen, G. J. Wang, M. L. Ho, and C. C. Tzeng, Med. Chem., 2013, 9, 748; CrossRef P. Bamborough, H. Diallo, J. D. Goodacre, L. Gordon, A. Lewis, J. T. Seal, D. M. Wilson, M. D. Woodrow, and C. W. Chung, J. Med. Chem., 2012, 55, 5876. CrossRef
11. J. S. Wzorek, T. F. Knopfel, I. Sapountzis, and D. A. Evans, Org. Lett., 2012, 14, 5840; CrossRef T. M. Kaiser, J. H. Huang, and J. Yang, J. Org. Chem., 2013, 78, 6297. CrossRef
12. T. M. V. D. P. E. Melo, Curr. Org. Chem., 2005, 9, 925. CrossRef
13. M. M. Heravi, F. Derikvand, A. Haeri, H. A. Oskooie, and F. F. Bamoharram, Synth. Commun., 2008, 38, 135; CrossRef V. Kumar, R. Aggarwal, and S. P. Singh, Heterocycles, 2008, 75, 2893. CrossRef
14. A. M. Jawalekar, E. Reubsaet, F. P. J. T. Rutjes, and F. L. van Delft, Chem. Commun., 2011, 47, 3198; CrossRef H. Kawai, Y. Sugita, E. Tokunaga, and N. Shibata, Eur. J. Org. Chem., 2012, 1295. CrossRef
15. A. Baranczak and G. A. Sulikowski, Org. Lett., 2012, 14, 1027; CrossRef K. M. Short and C. B. Ziegler, Jr., Tetrahedron Lett., 1993, 34, 75. CrossRef
16. C. Praveen, A. Kalyanasundaram, and P. T. Perumal, Synlett, 2010, 5, 777; CrossRef E. F. Ullman and B. Singh, J. Am. Chem. Soc., 1966, 88, 1844. CrossRef
17. T. V. Hansen, P. Wu, and V. V. Fokin, J. Org. Chem., 2005, 70, 7761; CrossRef S. B. Bharate, A. K. Padala, B. A. Dar, R. R. Yadav, B. Singh, and R. A. Vishwakarma, Tetrahedron Lett., 2013, 54, 3558. CrossRef
18. R. Raji, V. Jonnalagadda, J. Enukonda, P. K. R. Gangireddy, and G. René, Eur. J. Org. Chem., 2012, 5767. CrossRef
19. S. Tang, J. He, Y. Sun, L. He, and X. She, Org. Lett., 2009, 11, 3982. CrossRef
20. M. Ueda, S. Sugita, A. Sato, T. Miyoshi, and O. Miyata, J. Org. Chem., 2012, 77, 9344; CrossRef M. Ueda, A. Sato, Y. Ikeda, T. Miyoshi, T. Naito, and O. Miyata, Org. Lett., 2010, 12, 2594; CrossRef M. Ueda, Y. Ikeda, A. Sato, Y. Ito, M. Kakiuchi, H. Shono, T. Miyoshi, T. Naito, and O. Miyata, Tetrahedron, 2011, 67, 4612. CrossRef
21. X. F. Wu, H. Neumann, and M. Beller, Chem. Eur. J., 2010, 16, 12104; CrossRef M. S. M. Ahmed, K. Kobayashi, and A. Mori, Org. Lett., 2005, 7, 4487. CrossRef
22. R. J. Cox, D. J. Ritson, T. A. Dane, J. Berge, J. P. H. Charmant, and A. Kantacha, Chem. Commun., 2005, 1037; CrossRef L. Chen and C. J. Li, Org. Lett., 2004, 6, 3151. CrossRef
23. R. Chinchilla and C. Najera, Chem. Rev., 2007, 107, 874. CrossRef
24. A. Yoshimura, K. R. Middleton, A. D. Todora, B. J. Kastern, S. R. Koski, A. V. Maskaev, and V. V. Zhdankin, Org. Lett., 2013, 15, 4010. CrossRef
25. B. Elena, B. Ricardo, D. S. Francesco, M. Stefano, and P. Roberto, Heterocycles, 1983, 20, 501. CrossRef
26. M. A. El-Kasaby and M. A. I. Salem, Egypt. J. Chem., 1981, 23, 123.