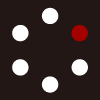
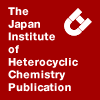
HETEROCYCLES
An International Journal for Reviews and Communications in Heterocyclic ChemistryWeb Edition ISSN: 1881-0942
Published online by The Japan Institute of Heterocyclic Chemistry
e-Journal
Full Text HTML
Received, 30th June, 2014, Accepted, 14th August, 2014, Published online, 27th August, 2014.
DOI: 10.3987/COM-14-S(K)58
■ Removal of Xylylene Groups from Tetrakis(o-xylylenedithio)phthalocyanines with Toluene/Aluminum Chloride and Construction of Dithiaphosphole Rings
Takeshi Kimura* and Shiduko Nakajo
Center for Instrumental Analysis, Iwate University, 4-3-5 Ueda, Morioka, Iwate 020-8551, Japan
Abstract
Xylylene groups were removed from tetrakis(o-xylylenedithio)phthalocyanines via C-S bond cleavage by treatment with toluene and aluminum chloride. Octathiolate anions generated were reacted with dichlorophenylphosphine to produce phthalocyanines with four dithiaphosphole rings. The structures of the products were determined by NMR and FABMS. Electrochemical and optical properties of them were verified by cyclic voltammetry and UV-vis spectroscopy.Phthalocyanines and related compounds have attracted much attention because of their potential applications to flexible electrochromic displays, field-effect transistors, solar cells, photosensitizers for photodynamic therapy of cancer, and so forth.1,2 New phthalocyanines have been explored for developing functional materials, which has stimulated a research field of functional dyes and pigments. To prepare symmetrically functionalized phthalocyanine derivatives, two methods can be mainly utilized; one is a tetramerization reaction of phthalonitriles or maleonitriles connected with special functional groups and the other is a functional group transformation of phthalocyanine or tetraazaporphyrin derivatives.1,2 The peripheral functional group transformation on the tetraazaporphyrins was first reported by Hoffman and co-workers using Birch reduction of C-S bonds with sodium metal in THF/liquid ammonia.3 Meanwhile, we reported the removal of the benzyl and o-xylylene groups on phthalocyanines via C-S bond cleavage, the deprotection reaction of which could be performed using lithium metal in THF/liquid ammonia to produce phthalocyanines with 1,3,2-dithiastannole and 1,2,3-trithiole rings after treatment with dibutyltindichloride and elemental sulfur, respectively.4 Recently, it was reported that the phosphorus, arsenic, and lead atoms strongly affected the absorption and emission spectra of phthalocyanines.5 If the phosphorus atom can be introduced on the five-membered ring instead of the tin atom of 1,3,2-dithiastannole, we can obtain phthalocyanine with 1,3,2-dithiaphosphole rings. On the other hand, there are few reports about phthalocyanines fused with the 1,3,2-dithiaphosphole rings because of their synthetic limitations.6 To obtain tetrakis(2-phenyl- 1,3,2-dithiaphospholo)phthalocyanine, we tried 1) the tetramerization of phthalonitrile fused with the 2-phenyl-1,3,2-dithiaphosphole ring and 2) the functional group transformation of phthalocyanine derivatives via C-S bond cleavage using toluene and aluminum chloride. This paper reports the preparation, structure determination, and optical and electrochemical properties of octabutylphthalocyanine fused with four 2-phenyl-1,3,2-dithiaphosphole rings and its nickel complex.
At first, 4,5-(o-xylylenedithio)-3,6-dibutylphthalonitrile (1) was prepared by a procedure reported previously.4 By a modified procedure of Decrutins’s deprotection method,7,8 1 was reacted with toluene in the presence of aluminum chloride under argon for 1 h to remove the o-xylylene group, and then dichlorophenylphosphine and triethylamine were added to the reaction mixture (Scheme 1). The solution was stirred at room temperature for 2 h to produce 2-phenyl-4,7-dibutyl-5,6-dicyanobenzo- 1,3,2-dithiaphosphole (2) in 57% yield.9 For the tetramerization of benzodithiaphosphole 2, the lithium alkoxide, prepared from lithium and excess 1-hexanol, was added to 2 and the solution was stirred at 110 °C for 1 h. However, we could not obtain the corresponding phthalocyanine. Compound 2 was decomposed under the reaction condition and produced a complex mixture.
Based on this result, we tried the functional group transformation of phthalocyanine derivatives. Although the peripheral functional group modification of phthalocyanine could have been performed by Birch reduction,3,4 we challenged a new process. Thus, phthalocyanine 4 and its nickel complex 4-Ni, prepared by a method reported previously,4 were treated with toluene and aluminum chloride (Scheme 2).7,8 In the case of 4-Ni, the green-colored solution changed to a red-brown color. The solution was stirred for 1 h and the C-S bonds of 4-Ni were cleaved by the electrophilic aromatic substitution reaction with toluene. Then, dichlorophenylphosphine and triethylamine were added and the solution was stirred for 2 h. After usual work-up and purification with silica gel column chromatography (hexane/chloroform) and bio-beads column chromatography (chloroform), tetrakis(2-phenyl- 1,3,2-dithiaphospholo)octabutylphthalocyaninato nickel (3-Ni) was obtained as a green powder in 30% yield.10 Compound 3 was obtained by the similar procedure but the yield was lower than that of the nickel derivative. Compounds 3 and 3-Ni are soluble in dichloromethane, chloroform, THF, and toluene.
1H NMR spectra of 3 and 3-Ni showed slightly broadened peaks for the butyl and phenyl groups, which may be related to structural isomers about the direction of the phenyl groups. The signal for two inner pyrrole protons of 3 was observed at δ = 0.37 ppm. Since 3 and 3-Ni have four phosphorus atoms, we could characterize the molecules by 31P NMR spectroscopy. The spectra showed split signals at δ = 41.5 ppm for 3 and δ = 40.5 ppm for 3-Ni. These signals are a slightly higher magnetic field than that of 2 (δ = 42.8 ppm) which is observed as a singlet peak. While the 31P NMR signal of 3-Ni splits into six peaks, that of 3 shows more complex peaks. Fast atom bombardment mass spectra (FABMS), measured using m-nitrobenzyl alcohol as a matrix, showed the corresponding molecular ion peaks at m/z = 1642.4 (M+) for 3 and m/z = 1698.4 (M+) for 3-Ni, suggesting that the four 2-phenyl-1,3,2-dithiaphosphole units are fused with the central phthalocyanine core.
In the UV-vis spectrum, the Q-band absorption of 3-Ni was observed at 742.5 nm (ε = 193,000) as a sharp signal (Figure 1a). In contrast, 3 showed the Q band absorption at 770.5 nm (ε =140,000) as a slightly broadened peak based on the Qx00 and Qy00 absorption. The Q band absorption of 3-Ni is observed at the shorter wavelength about 28 nm than that of 3. To determine the electrochemical properties of 3 and 3-Ni, oxidation and reduction potentials of them were measured by cyclic voltammetry (Table 1). The voltammogram of 3 (Figure 1b) shows one reversible couple for oxidation (E1/2 = 0.48 V) and two reversible couples for reduction (E1/2 = –1.33 and –1.05 V). In the voltammogram of 3-Ni, we can find one quasi-reversible couple for oxidation (E1/2 = 0.54 V) and two quasi-reversible couples for reduction (E1/2 = –1.60 and –1.16 V). While the first oxidation potential of 3 is lower than that of 3-Ni, these are slightly higher than those of 4 and 4-Ni, respectively. Compound 2 did not show clear oxidation peaks between 0 and 1.8 V.
In conclusion, phthalocyanines 3 and 3-Ni were prepared from 4 and 4-Ni via the removal of the o-xylylene groups and the reaction with dichlorophenylphosphine, respectively. The deprotection of the o-xylylene groups would be easier than that with Birch reduction; hence, the reaction could provide a new method for the peripheral functional group modification of phthalocyanine derivatives. The signals of 3 and 3-Ni in the 31P NMR spectra are observed at a slightly higher magnetic field than that of 2. The Q bands of 3 and 3-Ni are shorter absorption than those of 4 and 4-Ni, respectively.
References
1. a) K. M. Kadish, K. M. Smith, and R. Guilard, ‘The Porphyrin Handbook’, Vols. 15-20, Academic Press, San Diego, 2003; b) C. C. Leznoff and A. B. P. Lever, ‘Phthalocyanines: Properties and Applications’, Vols. 1-4, VCH, New York, 1989-1996.
2. a) G. de la Torre, P. Vázquez, F. Agulló-López, and T. Torres, Chem. Rev., 2004, 104, 3723; CrossRef b) J. Mack and N. Kobayashi, Chem. Rev., 2011, 111, 281. CrossRef
3. a) C. S. Velázquez, W. E. Broderick, M. Sabat, A. G. M. Barrett, and B. M. Hoffman, J. Am. Chem. Soc., 1990, 112, 7408; CrossRef b) C. S. Velázquez, G. A. Fox, W. E. Broderick, K. A. Anderson, O. P. Anderson, A. G. M. Barrett, and B. M. Hoffman, J. Am. Chem. Soc., 1992, 114, 7416. CrossRef
4. a) T. Kimura, A. Yomogita, T. Matsutani, T. Suzuki, I. Tanaka, Y. Kawai, Y. Takaguchi, T. Wakahara, and T. Akasaka, J. Org. Chem., 2004, 69, 4716; CrossRef b) T. Kimura, T. Suzuki, Y. Takaguchi, A. Yomogita, T. Wakahara, and T. Akasaka, Eur. J. Org. Chem., 2006, 1262. CrossRef
5. a) N. Kobayashi, T. Furuyama, and K. Satoh, J. Am. Chem. Soc., 2011, 133, 19642; CrossRef b) T. Furuyama, K. Satoh, T. Kushiya, and N. Kobayashi, J. Am. Chem. Soc., 2014, 136, 765. CrossRef
6. a) A. C. Sau and R. R. Holmes, J. Organomet. Chem., 1978, 156, 253; CrossRef b) N. Burford, B. W. Royan, A. Linden, and T. S. Cameron, Inorg. Chem., 1989, 28, 144; CrossRef c) S. Ogawa, M. Saito, M. Ogasawara, and R. Sato, Heterocycles, 1995, 41, 889. CrossRef
7. a) C. Loosli, C. Jia, S.-X. Liu, M. Haas, M. Dias, E. Levillain, A. Neels, G. Labat, A. Hauser, and S. Decurtins, J. Org. Chem., 2005, 70, 4988; CrossRef b) C. A. Donders, S.-X. Liu, C. Loosli, L. Sanguinet, A. Neels, and S. Decurtins, Tetrahedron, 2006, 62, 3543. CrossRef
8. a) T. Kimura, T. Namauo, N. Takahashi, Y. Takaguchi, T. Hoshi, and N. Kobayashi, J. Porphyrins Phthalocyanines, 2011, 15, 547; CrossRef b) T. Kimura, Heterocycles, 2013, 87, 245. CrossRef
9. 2: 1H NMR (500 MHz, CDCl3) δ 0.95 (t, J = 7.4 Hz, 6H, CH3), 1.41 (sext, J = 7.4 Hz, 4H, CH2), 1.54-1.67 (m, 4H, CH2), 2.93-3.04 (m, 4H, CH2), 7.28-7.37 (m, 3H, ArH), 7.41-7.47 (m, 2H, ArH); 31P NMR (202 MHz, CDCl3) δ 42.8; HRMS (EI): calcd for C22H23N2PS2, 410.1040; found, 410.1024 (M+).
10. 3: 1H NMR (500 MHz, CDCl3) δ 0.37 (bs, 2H, NH), 0.80 (t, J = 7.3 Hz, 24H, CH3), 1.37-1.49 (m, 16H, CH2), 1.75-1.89 (m, 16H, CH2), 4.50 (bs, 16H, CH2), 7.17-7.27 (m, 12H, ArH), 7.61-7.73 (m, 8H, ArH); 31P NMR (202 MHz, CDCl3) δ 41.5; FABMS m/z 1642.4 [M+]; 3-Ni: 1H NMR (500 MHz, CDCl3) δ 0.80 (t, J = 7.4 Hz, 24H, CH3), 1.34-1.47 (m, 16H, CH2), 1.71-1.89 (m, 16H, CH2), 4.43 (bs, 16H, CH2) 7.19-7.27 (m, 12H, ArH), 7.64-7.71 (m, 8H, ArH); 31P NMR (202 MHz, CDCl3) δ 40.5 (JP-C = 46, 18 Hz); FABMS m/z 1698.4 [M+].