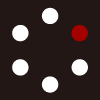
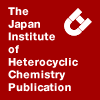
HETEROCYCLES
An International Journal for Reviews and Communications in Heterocyclic ChemistryWeb Edition ISSN: 1881-0942
Published online by The Japan Institute of Heterocyclic Chemistry
e-Journal
Full Text HTML
Received, 26th June, 2014, Accepted, 1st October, 2014, Published online, 6th October, 2014.
DOI: 10.3987/COM-14-S(K)44
■ Redox Responsive Polymer Incorporated with Mesogenic Unit and Bis(benzodithiolyl)bithienyl Scaffold
Toshihiro Ohtake,* Hideki Tanaka, Tetsuro Matsumoto, Mutsumi Kimura, and Akira Ohta*
Department of Chemistry, Faculty of Science, Shinshu University, Asahi 3-1-1, Matsumoto, Nagano 390-8621, Japan
Abstract
A redox-active polymer consisting of a poly(siloxane) backbone, a liquid crystalline (LC) unit, and bis(benzodithiolyl)bithienyl moieties was designed and synthesized. The resulting polymer after the incorporation of the redox-active and LC functional units into the poly(siloxane) backbone exhibits a redox response associated with the electrochemical interconversion of the bis(benzodithiolyl)bithienyl moieties.Stimulus-responsive molecules, which undergo changes in their structure or properties induced by external stimuli such as light,1 pH,2 or ionic interaction,3 have attracted considerable interest due to their potential application to dynamic functional materials. In particular, redox-triggered conformational changes in molecules4–10 have been intensively studied as a key component to fabricate actuating or sensing materials. If the dynamic nature of molecules undergoing conformational changes is incorporated into a liquid crystalline (LC) polymer backbone,11–13 novel deformable materials exhibiting effective changes in volume or strain can be achieved. Recently, redox-active molecular switches14 and bistable rotaxane molecules15 have been functionalized with LC molecular units. However, a limited number of redox-active polymers incorporated within conformationally changing unit molecules have been reported.16,17 We have designed and synthesized redox-active polymers 4 and 5 based on a poly(siloxane) backbone as illustrated in Scheme 1. We have employed a 2,2'-bis(benzodithiolyl)-3,3'-bithienyl unit18 as the conformational changing unit, which undergoes redox-triggered conformational interconversion as shown in Scheme 2. When this functional unit is incorporated into a linear polymer chain, the conformational change in the 2,2'-bis(benzodithiolyl)-3,3'-bithienyl unit is expected to induce a change in the free volume of the polymer chain,11 which alters the stable packing structures of the polymer chains (Figure 1). Our intention is to induce macroscopic deformation in the bulk polymer by enhancing the deformation and free volume change of the polymer chain in the ordered molecular field. We have used a fluorinated terphenyl19 compound 1 and a vinyl terminated bis(benzodithiolyl)bithienyl derivative 2 to incorporate the LC unit and the conformation changing function, respectively, in poly(dimethylsiloxane-co-hydrogenmethylsiloxane) (3). Herein, we demonstrate the synthesis and electrochemical properties of the redox-active polymer containing mesogenic units.
The reactive terphenyl mesogenic monomer 1 incorporated with a terminal vinyl group was prepared via 5 steps from 4-bromophenol (Scheme S1). The terphenyl compound 1 exhibits nematic and smectic C phases as confirmed by differential scanning calorimetry (Figure S1), polarized optical microscopy, and X-ray diffraction (XRD). Schlieren textures typical of nematic and smectic C phases (Figure S2) were observed. A sharp diffraction peak at 2θ = 3.12° (d = 28.3 Å), which is characteristic of a smectic layer structure, was observed at 98 °C in the XRD pattern (Figure S3). The redox-active molecular switch 2 was synthesized from 2,2'-diformyl-4,4'-dibromo-3,3'-bithienyl (Scheme S2). UV-Vis spectra of 2 and 22+ were measured in dichloromethane (CH2Cl2) (Figure S4). The absorption maximum of 22+ was 424 nm, whereas the absorption band in visible region was not observed for 2. This behavior is similar to that of 2,2'-bis(benzodithiolyl)-3,3'-bithienyl (Scheme 2).18
The structure of 2 was characterized by X-ray crystallography (Figure 2).20 Single crystals of 2 suitable for X-ray crystal analysis were recrystallized from a toluene/hexane solution by slow evaporation. Single crystals of 2 were crystallized in the space group C2/c. The dihedral angle of the bithienyl moiety of 2 was 28.0°, which is much larger than that of 2,2'-bis(benzodithiolyl)bithienyl (8.3°)18 or bis(thioxanthyl)-substituted oligothiophene (1.4°),21 suggesting that the incorporation of a phenylene group in the thiophene ring caused the more twisted conformation of the bithienyl moiety than that in the unsubstituted 2,2'-bis(benzodithiolyl)-3,3'-bithienyl.18 The bond length of the C–C bond formed through reduction is 1.582(3) Å, which is approximately the length reported in our previous results (1.583(3) Å).18
The cyclic voltammogram of 2 showed irreversible anodic and broad cathodic peaks at +0.74 V and −0.40 V (vs. Fc/Fc+), respectively (Figure 3). The large peak separation between oxidation and reduction is similar to that of unsubstituted parent 2,2'-bis(benzodithiolyl)-3,3'-bithienyl, indicating the redox-induced C–C bond formation and cleavage.18 The electrochemically triggered interconversion between the cyclic form and the dicationic form was further confirmed by spectroelectrochemical studies (Figure 4).
The incorporation of mesogenic and redox-active units in the poly(siloxane) backbone was carried out by conventional hydrosilylation of 1 and 2 with poly(dimethylsiloxane-co-hydrogenmethylsiloxane) (3) in the presence of the (1,3-divinyl-1,1,3,3-tetramethyldisiloxane)platinum(0) complex in toluene at 85 °C.22 The formations of 4 and 5 were confirmed by NMR spectra and the molar ratios of the redox-active unit, mesogenic core, and unreacted SiH groups of 5 were calculated to be 38%, 35%, and 27%, respectively (Figure S5). In contrast, the signals corresponding to SiH groups in the 1H NMR (near 4.7 ppm) and IR (near 2160 cm−1) were not observed for 4, indicating that the SiH groups were completely consumed. After the attachment of 2 to 3, polymer 4 was obtained as an orange transparent gel (Figure 5A), indicating the formation of porous and fibrous network structures.23 The microstructure of polymer 4 was studied by SEM (Figure 5B), and the porous structure was observed. Such gel formation was not observed for 5, which was obtained as a light brown powder that was soluble in toluene or CH2Cl2.
Attempts to evaluate the electrochemical properties of 4 in the solution by cyclic voltammetry (CV) were not successful because the polymer gel 4 does not dissolve in organic solvents commonly used for CV. Thus the polymer gel 4 was prepared directly on a transparent indium tin oxide (ITO) electrode, and electrochemical analysis was performed for the modified electrodes. In contrast, the LC-incorporated polymer 5 was dissolvable in CH2Cl2, and CV measurements were carried out in the solution. The results of the CV measurements for 4 and 5 are shown in Figure 6. Similar CV curves to that of 2 were obtained
for both 4 and 5. Using the same rationale as for the CV of 2, the redox peaks found for 4 or 5 may be assigned to the oxidation and reduction of the bis(benzodithiolyl)bithienyl moiety attached to the poly(siloxane) backbone.
The electrochemical response for 5 was monitored by UV-Vis spectroscopy (Figure 7). Oxidation of 5 caused the increase in the absorption band around 350 and 480 nm. These peaks almost disappeared when 5 was reduced. This behavior is slightly different from that of 2. This is likely due to the influence of the structure of 5 in which the bis(benzodithiolyl)bithienyl units were incorporated into the polymer chain. Such spectral changes in the visible region upon electrochemical oxidation and reduction indicate the interconversion between the cationic and cyclized forms of the bis(benzodithiolyl)bithienyl moiety. Unexpectedly, 5 did not exhibit a liquid crystalline phases. The increase in the number of mesogenic units in 5 may induce LC properties. When the SiH units were substituted completely with only 1 (14, see Supporting Information), a birefringence texture characteristic of the smectic A phase was observed under polarized optical microscopy (Figure S6). The optimization of the molar ratio of the LC unit and the redox-active unit are necessary to form the stable ordered structure of 5.
In summary, we have prepared redox-active polymeric materials in which a liquid crystalline mesogenic moiety and a bis(benzodithiolyl)bithienyl scaffold are attached to poly(siloxane) backbone as a pendant group and bridging unit, respectively. The redox-active function of the polymers has been confirmed by CV and UV-Vis spectroscopy. The actuation behaviors of these functional polymers and the optimization of components of the functional units to construct ordered structures are currently under investigation.
ACKNOWLEDGEMENTS
The authors wish to thank Dr. Masahiro Furusawa (Seiko Epson) for helpful discussions. Tomohiro Fujita and Etsuko Takei (Seiko Epson) are thanked for HRMS measurements. The authors also thank the Instrument Center of the Institute of Molecular Science for X-ray crystal structure analysis. Dr. Masaaki Tomura (Institute of Molecular Science) is thanked for HRMS measurements.
PRESENT ADDRESS
†Seiko Epson Corporation, 1010 Fujimi, Fujimi-machi, Suwa-gun, Nagano 399-0295, Japan
SUPPORTING INFORMATION
Supporting information for this article is available on the web site under http://www.heterocycles.jp or from the corresponding authors.
References
1. M. C. Basheer, Y. Oka, M. Mathews, and N. Tamaoki, Chem. Eur. J., 2010, 16, 3489. CrossRef
2. M. V. R. Raju, P. Raghunath, M.-C. Lin, and H.-C. Lin, Macromolecules, 2013, 46, 6731. CrossRef
3. J. Cao, J.-B. Guo, P.-F. Li, and C.-F. Chen, J. Org. Chem., 2011, 76, 1644. CrossRef
4. T. Suzuki, K. Hanada, R. Katoono, Y. Ishigaki, S. Higasa, H. Higuchi, H. Kikuchi, K. Fujiwara, H. Yamada, and T. Fukushima, Chem. Lett., 2014, 43, 982. CrossRef
5. T. Suzuki, Y. Ishigaki, T. Iwai, H. Kawai, K. Fujiwara, H. Ikeda, Y. Kano, and K. Mizuno, Chem. Eur. J., 2009, 15, 9434. CrossRef
6. J. Nishida, T. Suzuki, M. Ohkita, and T. Tsuji, Angew. Chem. Int. Ed., 2001, 40, 3251. CrossRef
7. H. Kanazawa, M. Higuchi, and K. Yamamoto, J. Am. Chem. Soc., 2005, 127, 16404. CrossRef
8. V. J. Chebny, R. Shukla, S. V Lindeman, and R. Rathore, Org. Lett., 2009, 11, 1939. CrossRef
9. I. Okamoto, R. Yamasaki, M. Sawamura, T. Kato, N. Nagayama, T. Takeya, O. Tamura, H. Masu, I. Azumaya, K. Yamaguchi, H. Kagechika, and A. Tanatani, Org. Lett., 2007, 9, 5545. CrossRef
10. H. G. Kim, C.-W. Lee, S. Yun, B. H. Hong, Y.-O. Kim, D. Kim, H. Ihm, J. W. Lee, E. C. Lee, P. Tarakeshwar, S.-M. Park, and K. S. Kim, Org. Lett., 2002, 4, 3971. CrossRef
11. A. Priimagi, A. Shimamura, M. Kondo, T. Hiraoka, S. Kubo, J.-I. Mamiya, M. Kinoshita, T. Ikeda, and A. Shishido, ACS Macro Lett., 2012, 1, 96. CrossRef
12. C. L. van Oosten, D. Corbett, D. Davies, M. Warner, C. W. M. Bastiaansen, and D. J. Broer, Macromolecules, 2008, 41, 8592. CrossRef
13. Y. Hayata, S. Nagano, Y. Takeoka, and T. Seki, ACS Macro Lett., 2012, 1, 1357. CrossRef
14. T. Ohtake, H. Tanaka, T. Matsumoto, M. Kimura, and A. Ohta, J. Org. Chem., 2014, 79, 6590. CrossRef
15. I. Aprahamian, T. Yasuda, T. Ikeda, S. Saha, W. R. Dichtel, K. Isoda, T. Kato, and J. F. Stoddart, Angew. Chem. Int. Ed., 2007, 46, 4675. CrossRef
16. M. J. Marsella and R. J. Reid, Macromolecules, 1999, 32, 5982. CrossRef
17. M. J. Marsella, R. J. Reid, S. Estassi, and L.-S. Wang, J. Am. Chem. Soc., 2002, 124, 12507. CrossRef
18. A. Ohta, C. Ueki, Y. Uchiyama, and K. Fujimori, Heterocycles, 2006, 69, 365. CrossRef
19. K. Kishimoto, T. Suzawa, T. Yokota, T. Mukai, H. Ohno, and T. Kato, J. Am. Chem. Soc., 2005, 127, 15618. CrossRef
20. Crystallographic data have been deposited at the Cambridge Crystallographic Data Centre: CCDC 1010071. These data can be obtained free of charge from The Cambridge Crystallographic Data Centre via www.ccdc.cam.ac.uk/data_request/cif.
21. J. Nishida, T. Miyagawa, and Y. Yamashita, Org. Lett., 2004, 6, 2523. CrossRef
22. A. Matsui, M. Funahashi, T. Tsuji, and T. Kato, Chem. Eur. J., 2010, 16, 13465. CrossRef
23. T. Kato, T. Kutsuna, K. Yabuuchi, and N. Mizoshita, Langmuir, 2002, 18, 7086. CrossRef