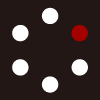
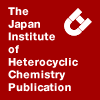
HETEROCYCLES
An International Journal for Reviews and Communications in Heterocyclic ChemistryWeb Edition ISSN: 1881-0942
Published online by The Japan Institute of Heterocyclic Chemistry
e-Journal
Full Text HTML
Received, 27th June, 2014, Accepted, 29th July, 2014, Published online, 8th August, 2014.
DOI: 10.3987/COM-14-S(K)50
■ The Cycloaddition of 2-Phenylamino-1-azaazulene with Diphenylacetylene Using Palladium Catalytic Systems
Hiroyuki Fujii,* Shigeki Oka, Ippei Nakamura, Yu Kawai, Reiko Ikeda, Takeo Konakahara, and Noritaka Abe
Scientific Research Center, Yamaguchi University, 1677-1 Yoshida, Yamaguchi 753-8512, Japan
Abstract
Selective cycloaddition was achieved by the reaction of 2-phenylamino-1-azaazulene (3) with diphenylacetylene (4) in the presence of Pd catalyst providing N-(1-azaazulen-2-yl)-2,3-diphenylindoles.1-Azaazulene is a non-alternant aza-heterocyclic system containing a fused five- and seven-membered ring, and it has interesting chemical, structural, and pharmaceutical properties.1 Recently, we reported that heterocycle-substituted 1-azaazulenes showed various bioactivities and some interesting functionalities.2 For example, benzimidazole-substituted 1-azaazulenes showed cytotoxic activity against HeLa S-3 cells.3
Indole is one of the most well-known heterocycles. The Fischer indole synthesis4 is the classic method for obtaining indole, and recently cyclization reactions using a Pd catalyst have been developed. Catalytic cyclizations of N-aryl-2-aminopyridines and internal alkynes have also been reported.5 Therefore, we tried to synthesize indole-substituted 1-azaazulenes, which should show interesting properties, by the catalytic reaction of 2-phenylamino-1-azaazulene (3) with diphenylacetylene (4).
A halogen at C-2 of 1-azaazulenes could be easily replaced with amino groups by treatment with amines or amide ions (amine treatment under basic conditions)6 or Buchwald-Hartwig heteroarylation (catalytic reaction using Pd).7 The 2-phenylamino-1-azaazulene (3) substrate was prepared in 67% yield by treating 2-chloro-1-azaazulene (1) and aniline (2) in 1,4-dioxane at 110 °C for 5 h (Scheme 1).
For the cycloaddition, we examined the reactions of 2-phenylamino-1-azaazulene (3) with diphenylacetylene (4) in the presence of a Pd catalyst and a Cu salt in DMF at 110 °C. Cycloaddition occurred and N-(1-azaazulen-2-yl)-2,3-diphenylindoles were obtained (Table 1).
The reaction of 3 with 4 was performed in the presence of PdCl2(MeCN)2 and CuCl2 for 24 h at 110 °C in DMF, and cycloadducts 5 (7%) and 6 (37%) were obtained (entry 1). Compound 6 was the product Cl-substituted at C-3 of the 1-azaazulene moiety of 5. When the reaction was carried out for 48 h, yields of each product were improved, and 5 and 6 were obtained in 11% and 60% yield, respectively (entry 2). When CuBr2 was used as an oxidant for the reaction, only traces of 5 were obtained and 7 was obtained in moderate yield (entries 3, 4). When PdCl2(PPh3)2 was used as a palladium catalyst or Cu(OAc)2 was used as an oxidant, the yields were not improved (entries 5, 6). The conversion of CuX2 to CuX decreased the reactivity, although 5 was obtained in moderate yield and the halogenation of C-3 at azaazulene did not occur (entries 7-9).
In the reaction, cycloaddition formed the indole framework; however, the halogen-substituted compounds at C-3 of the azaazulene moiety were obtained under these conditions. Therefore, we examined the reaction of compound 8, which contained bromine at C-3 of the 1-azaazulene ring system (Table 2). Compound 8 can be readily synthesized from compound 3 with NBS.
The reaction of 8 with 4 produced an interesting result. Treatment of 8 with diphenylacetylene for 24 h gave 7 as a major product together with debrominated product 5. Prolonging the reaction decreased the yield of 7 and increased that of 5. These results suggest that the halogen at C-3 of azaazulene was eliminated after cycloaddition to the aniline ring moiety.
The structure of 5 was determined by X-ray crystallography, 1H NMR, 13C NMR, and HRMS. The ORTEP drawing of 5 is shown in Figure 1. The structure of 7 was assigned by 1H NMR and HRMS by comparison with those of 5. In the 1H NMR spectrum of 5, the proton signal at C-3 of the azaazulene moiety appeared at δ 6.27 as a singlet. In the 1H NMR spectrum of 7, the corresponding peak disappeared. HRMS of 7 confirmed the presence of bromine. Furthermore, 7 was prepared by the reaction of 5 with NBS.
The X-ray crystallography data for 5 showed that both the 1-azaazulene and indole rings are nearly co-planar and they retain their aromaticity. The results suggest that the π-conjugation should extend further. The phenyl group at C-2 of the indole ring rotates from the indole plane because of the steric repulsion between H-3 of 1-azaazulene and the phenyl group.
The terminal acetylenes phenylacetylene and trimethylsilylacetylene and the electron deficient acetylene DMAD were used to examine the utility of this reaction. However, the desired product was not obtained, except for the products halogenated at C-3 of azaazulene. The cycloaddition of 2-phenylamino-1-azaazulene (3) with diphenylacetylene (4) occurred only on the aniline moiety, and afforded the indole derivatives as products.
Ring formation creates the five-membered ring, and cyclization on the five-membered ring of the 1-azaazulene is disfavored because azapentalene ring formation would be harder than indole ring formation. Therefore, cycloaddition occurred preferentially at the aniline moiety and generated a stable indole ring.
UV/Vis spectra of 2-phenylamino-1-azaazulene (3) and the cyclized products (5, 6, and 7) in MeCN are shown in Figure 2. The spectra of 3 and 5 are similar, suggesting that the π-conjugation of 1-azaazulene with aniline is comparable to that of 1-azaazulene with indole. On the other hand, the spectra of 6 and 7 differ from those of 3 and 5. In the spectra of 6 and 7, the dihedral angle of the 1-azaazulene ring and the indole ring increases because of the steric repulsion of the phenyl group at C-2 of the indole and the halogen at C-3 of 1-azaazulene resulting from the halogen substitution at C-3 of 1-azaazulene. Thus, the π-conjugation between 1-azaazulene and indole is decreased.
Some heteroaryl-substituted 1-azaazulenes and fused 1-azaazulenes showed strong cytotoxic activity against HeLa S-3 cells. We evaluated the cytotoxic activity of compound 5 against HeLa S-3 cells. However, the IC50 value of 5 was >50.4 µM, which is not sufficiently high activity against HeLa S-3 cells.
EXPERIMENTAL
Melting points were measured using a Yanagimoto micro-melting apparatus and were uncorrected. 1H NMR (400 MHz) and 13C NMR (100.6 MHz) spectra were recorded on a Bruker AVANCE 400S using deuterochloroform as a solvent and tetramethylsilane as an internal standard. J values are recorded in Hz. IR spectra were recorded for KBr pellets on a Nicolet FT-IR Impact 410 unless otherwise stated. Electronic spectra were recorded with Shimadzu UV-1600PC spectrophotometer. Mass spectra (ESI-MS) were measured with a JEOL JMS-T100CS spectrometer or a BRUKER DALTONICS microTOF II (APCI) instrument. GC-Mass spectra were taken with a Shimadzu GC-MS QP2010 Plus instrument. Elemental analyses were performed with a Perkin Elmer 2400 II system. Kieselgel 60 was used for column chromatography and Kieselgel 60G was used for thin-layer chromatography.
Synthesis of 2-phenylamino-1-azaazulene (3)
Under an argon atmosphere, a mixture of 2-chlolo-1-azaazulene (1) (80 mg, 0.5 mmol) and aniline (2) (61 mg, 0.6 mmol) in 1,4-dioxane (5 mL) was stirred for 5 h at 110 °C. The reaction mixture was poured into water (40 mL) and extracted with CHCl3. The organic layer was dried over Na2SO4, and the solvent was evaporated in vacuo. Column chromatography (SiO2, CHCl3:AcOEt = 1:1) of the residue gave 3 (74 mg, 67%).
3: Red-orange powder (from CH2Cl2/n-hexane), mp 155–156 °C; δH 6.94 (1H, s), 7.11 (1H, m), 7.34–7.42 (4H, m), 7.48 (3H, d, J = 7.7), 8.0 (2H, dd, J = 9.0, 0.4), 8.9-9.6 (1H, br); δC 98.5, 119.5, 123.3, 127.2, 128.4, 129.4, 129.5, 130.5, 130.8, 140.4, 148.6, 158.1, 165.3; HRMS ESI+: Calcd for C15H12N2: 221.1084. Found: m/z 221.1073 ([M + H]+).
Reaction of 2-phenylamino-1-azaazulene (3) with diphenylacetylene (4) in the presence of PdCl2(MeCN)2 and CuCl
Typical procedure: Under an argon atmosphere, a mixture of 2-phenylamino-1-azaazulene (3) (44 mg, 0.2 mmol), diphenylacetylene (53 mg, 0.3 mmol), PdCl2(MeCN)2 (2.1 mg, 4 mol %), and CuCl (41 mg, 0.42 mmol) in DMF (5 mL) was stirred for 48 h at 110 °C. The reaction mixture was poured into water (40 mL) and extracted with CHCl3. The organic layer was dried over Na2SO4, and the solvent was evaporated in vacuo. Column chromatography (SiO2, n-hexane:AcOEt = 9:1) of the residue gave 5 (9 mg, 11%) and 6 (51 mg, 60%).
5: Red-orange needles (from CH2Cl2/n-hexane), mp 201–202 °C; δH 6.27 (1H, s), 7.21–7.25 (2H, m), 7.26–7.29 (4H, m), 7.30–7.35 (4H, m), 7.35–7.42 (3H, m), 7.45–7.52 (1H, m), 7.66–7.76 (3H, m), 8.10 (1H, d, J = 10.0), 8.62 (1H, dd, J = 8.0, 2.2), 8.71 (1H, d, J = 8.3); δC 107.8, 114.3, 119.4, 120.4, 122.3, 124.1, 126.3, 127.9, 128.2, 128.2, 128.9, 129.0, 129.8, 130.2, 131.3, 132.1, 133.5, 134.0, 134.2, 135.3, 136.0, 137.5, 145.9, 155.9, 161.2; HRMS ESI+: Calcd for C29H21N2: 397.17047. Found: m/z 397.16990 ([M + H]+).
6: Red needles (from CH2Cl2/n-hexane), mp 167–168 °C; δH 7.01–7.16 (5H, m), 7.20–7.30 (3H, m), 7.32–7.42 (4H, m), 7.53 (1H, dd, J = 6.6, 2.1), 7.68–7.76 (2H, m), 7.77–7.83 (1H, m), 7.87–7.95 (1H, m), 8.42 (1H, d, J = 9.9), 8.63 (1H, dd, J = 9.6, 0.74); δC 110.0, 111.7, 118.6, 119.6, 121.7, 123.4, 126.3, 127.2, 127.7, 128.2, 128.8, 129.3, 130.3, 130.4, 130.5, 131.6, 133.6, 134.5, 136.8, 137.3, 137.8, 138.6, 141.6, 153.4, 156,1; HRMS ESI+: Calcd for C29H19ClN2Na: 453.11345. Found: m/z 453.11257 ([M + Na]+).
Reaction of 2-phenylamino-1-azaazulene (3) with diphenylacetylene (4) in the presence of PdCl2(MeCN)2 and CuBr2
Typical procedure: Under an argon atmosphere, a mixture of 2-phenylamino-1-azaazulene (3) (44 mg, 0.2 mmol), diphenylacetylene (4) (53 mg, 0.3 mmol), PdCl2(MeCN)2 (2.1 mg, 4 mol%), and CuBr2 (98 mg, 0.42 mmol) in DMF (5 mL) was stirred for 48 h at 110 °C. The reaction mixture was poured into water (40 mL), and extracted with CHCl3. The organic layer was dried over Na2SO4, and the solvent was evaporated in vacuo. Column chromatography (SiO2, n-hexane:AcOEt = 9:1) of the residue gave 7 (44 mg, 46%).
7: Red needles (from CH2Cl2/n-hexane), mp 168–169 °C; δH 7.00–7.17 (5H, m), 7.20–7.23 (1H, m), 7.24–7.29 (2H, m), 7.32–7.36 (2H, m), 7.39–7.42 (3H, m), 7.68–7.81 (3H, m), 7.86–7.91 (1H, m), 8.42 (1H, d, J = 9.8), 8.62 (1H, dd, J = 9.6, 0.8); δC 98.5, 111.7, 118.5, 119.6, 121.6, 123.3, 126.2, 127.2, 127.6, 128.3, 128.8, 129.7, 130.3, 130.4, 130.6, 131.6, 134.6, 135.2, 137.0, 137.3, 138.0, 138.7, 143.7, 154.7, 158.0; HRMS ESI+: Calcd for C29H19BrN2Na: 497.06293. Found: m/z 497.06054 ([M + Na]+).
Synthesis of 3-bromo-2-phenylamino-1-azaazulene (8)
Under an argon atmosphere, a mixture of 2-phenylamino-1-azaazulene (3) (110 mg, 0.5 mmol) and NBS (107 mg, 0.6 mmol) in CHCl3 (10 mL) was stirred for 1 h at rt. The reaction mixture was poured into water (40 mL) and extracted with CHCl3. The organic layer was dried over Na2SO4, and the solvent was evaporated in vacuo. Column chromatography (SiO2, CHCl3:AcOEt = 3:1) of the residue gave 8 (109 mg, 73%).
8: Red-brown powder (from CH2Cl2/n-hexane), mp 135–136 °C; δH 7.10 (1H, m), 7.12–7.16 (1H, br), 7.36–7.56 (5H, m), 7.90–7.96 (3H, m), 8.09 (1H, dd, J = 9.5, 0.4); δC 89.3, 119.1, 123.2, 127.4, 128.6, 129.2, 129.8, 130.7, 131.7, 139.4, 142.3, 157.2, 160.2; HRMS ESI+: Calcd for C15H11N2Br: 229.0178. Found: m/z 229.0178 ([M + H]+).
X-Ray structure determination
Single-crystal XRD analysis was performed using a Rigaku Mercury CCD area detector with graphite-monochromated Mo-Kα radiation. The data were collected at 173 K to a maximum 2θ value of 55.0. The structure was determined by direct methods and expanded using Fourier techniques.
Crystal data for 5: Red-orange needles, C29H20N2, M = 396.48 g/mol, tetragonal, a = 26.38(3), b = 26.38(3), c = 11.949(11) Å, V = 8316(14) Å3, T = 173 K, space group I41/a, Z = 16, Dcalcd = 1.263 g/cm3, crystal dimensions 0.20 × 0.20 × 0.20 mm, 25620 reflections measured, 4730 unique (Rint = 0.0820) which were used in all calculations. The final R1 (observed data) and wR2 (all data) were 0.0461 and 0.1811, respectively. The crystallographic data in CIF format are available from the Cambridge Crystallographic Data Centre, CCDC reference number 1012404.
References
1. a) N. Abe and T. Gunji, Heterocycles, 2010, 82, 201; CrossRef b) N. Abe, ‘Recent Research Developments in Organic and Bioorganic Chemistry’, 2001, 4, 14; Transworld Research Network; c) N. Abe, ‘Trends in Heterocyclic Chemistry’, 2001, 7, 25; Research Trends; d) T. Nishiwaki and N. Abe, Heterocycles, 1981, 15, 547; CrossRef e) M. Kimura, Yuki Gousei Kagaku Kyokai Shi, 1981, 39, 690. CrossRef
2. a) T. Ariyoshi, K. Yoshinaga, K. Koizumi, H. Fujii, R. Ikeda, T. Konakahara, and N. Abe, Heterocycles, 2010, 80, 427; CrossRef b) E. Yoshioka, H. Fujii, T. Murafuji, R. Ikeda, T. Konakahara, T. Gunji, and N. Abe, Heterocycles, 2011, 83, 1409; CrossRef c) M. Nakatani, H. Fujii, T. Murafuji, T. Gunji, R. Ikeda, T. Konakahara, and N. Abe, Heterocycles, 2012, 84, 461; CrossRef d) E. Yoshioka, k. Koizumi, K. Nakashima, H. Fujii, T. Murafuji, T. Gunji, and N. Abe, Heterocycles, 2012, 85, 1683. CrossRef
3. a) K. Koizumi, K. Shimabara, A. Takemoto, S. Yamazaki, N. Yamauchi, H. Fujii, M. Kurosawa, T. Konakahara, and N. Abe, Heterocycles, 2009, 79, 319; CrossRef b) N. Yamauchi, H. Fujii, A. Kakehi, M. Shiro, M. Kurosawa, T. Konakahara, and N. Abe, Heterocycles, 2008, 76, 617. CrossRef
4. A. Guy and J. Guette, Synthesis, 1980, 222. CrossRef
5. a) J. Chen, Q. Pang, Y. Sun, and X. Li, J. Org. Chem., 2011, 76, 3523; CrossRef b) X. Chen, X. Li, N. Wang, J. Jin, P. Lu, and Y. Wang, Eur. J. Org. Chem., 2012, 4380. CrossRef
6. a) N. Abe, T. Nishiwaki, H. Yamamoto, and N. Kunishige, Bull. Chem. Soc. Jpn., 1983, 56, 3703; CrossRef b) K. Fujimori, N. Hirako, and K. Yamane, Bull. Chem. Soc. Jpn., 1983, 56, 1247. CrossRef
7. a) K. Koizumi, K. Shimabara, A. Takemoto, S. Yamazaki, N. Yamauchi, H. Fujii, M. Kurosawa, T. Konakahara, and N. Abe, Heterocycles, 2009, 79, 319; CrossRef b) E. Yoshioka, K. Koizumi, S. Yamazaki, H. Fujii, and N. Abe, Heterocycles, 2009, 78, 3065. CrossRef