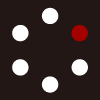
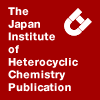
HETEROCYCLES
An International Journal for Reviews and Communications in Heterocyclic ChemistryWeb Edition ISSN: 1881-0942
Published online by The Japan Institute of Heterocyclic Chemistry
e-Journal
Full Text HTML
Received, 4th March, 2014, Accepted, 21st April, 2014, Published online, 1st May, 2014.
DOI: 10.3987/COM-14-S(K)16
■ Hexaaryl-benzodipyrroles: Properties and Application as Amorphous Carrier-Transporting Materials
Hayato Tsuji,* Yuki Yokoi, Shunsuke Furukawa, and Eiichi Nakamura*
Department of Chemistry, Graduate School of Science, The University of Tokyo, 1-1-1 Yayoi, Bunkyo-ku, Tokyo 113-8567, Japan
Abstract
We have newly synthesized 1,2,3,5,6,7-hexaarylbenzo[1,2-b:4,5-b′]dipyrroles (HABDPs) and studied their properties. The HABDPs showed UV absorption maxima at 377–390 nm, indicating their extended π-conjugation system. They were also found to serve as efficient amorphous materials, featuring high electrochemical and thermal stability with high carrier mobility of up to 10–3 cm2/Vs using the time-of-flight technique. Application of the HABDPs to organic light-emitting diodes resulted in significantly improved performance in driving voltage and efficiency compared with α-NPD, a commonly used hole-transporting material.INTRODUCTION
Pyrrole derivatives are acknowledged as an important class of compounds in a broad range of fields, such as natural products and bioactive molecular chemistry,1,2 supramolecular and coordination chemistry,3 and materials science.4 We have so far developed novel fused polycyclic heteroaromatic compounds,5–11 including N,N′-HexBn-protected (HexBn = (4-hexylphenyl)methyl) benzo[1,2-b:4,5-b′]dipyrroles (HexBn-BDPs), and found that the HexBn-BDPs serve as solution-processable hole injection materials in organic light-emitting diodes (OLEDs) and afforded mechanistic insights for the hole injection. In this manuscript, we report newly synthesized N,N′-diaryl-BDPs, that is, 1,2,3,5,6,7-hexaarylbenzo[1,2-b:4,5-b′]dipyrroles (HABDPs), which show intriguing properties, such as high stability and high carrier mobility, and are suitable to serve as a novel class of hole-transporting materials in OLEDs to achieve significant improvement in driving voltage and efficiency.
RESULTS AND DISCUSSION
Synthesis of the HABDPs is shown in Scheme 1. Using our previously developed zinc-mediated cyclization reaction, N,N′-HexBn-protected BDPs 2 were prepared, which was followed by deprotection using AlCl3 in anisole12 to obtain N,N′-protonated BDPs 3 in moderate yield. A palladium-catalyzed N-arylation reaction13 afforded the new class of compounds HABDPs 4a–d in 55%, 52%, 58%, and 38% yields, respectively.
Photophysical and electrochemical property measurements of the HABDPs 4 in solution demonstrated their extended π-conjugation character (Table 1). The UV absorption spectra of 4a–c (R1 = H) showed similar longest absorption maxima and onset wavelengths at 377–382 nm and 424–430 nm, respectively (Figure 1), while that of 4d (R1 = NPh2) is somewhat redshifted with shoulder-like transition around 390 nm and onset at 449 nm, indicating the extension of π-conjugation because of the additional diphenylamino group at the 2,6-positions. These absorption maximum wavelengths are similar to those of the benzo[1,2-b:4,5-b′]difurans (BDFs), which we have previously reported,5 showing a small difference in the absorption nature from the oxygen counterparts. The cyclic voltammogram of these BDPs in CH2Cl2 showed a reversible first oxidation wave with potentials of 0.23, 0.18, 0.25, and 0.12 V (vs Fc/Fc+) for 4a–d, respectively, indicating electrochemical stability toward oxidation. No reduction wave was observed within the solvent window. The HOMO energy levels were estimated to be –5.03, –4.98, –5.05, and –4.92 eV, respectively. The LUMO energy levels, calculated from the HOMO energy and the optical gap (Eg), were –2.15, –2.10, –2.13, and –2.16 eV, respectively. These HOMO and LUMO energy levels are somewhat higher than those of the corresponding BDFs (e.g., HOMO: –5.49 eV; LUMO: –2.54 eV for 2,3,6,7-tetraphenylbenzo[1,2-b:4,5-b′]difuran), representing the increased electron-donating nature of the incorporated nitrogen atoms compared with oxygen.
The thermal stabilities of the BDPs are high: thermogravimetric analysis (TGA) showed 5% weight loss (Td) at 424 °C for 4a because of sublimation and 489, >500, and 486 °C for 4b–d, respectively. The BDP 4d exhibited a glass transition temperature (Tg) at 121 °C seen by differential scanning calorimetry (DSC). The observed Tg values are substantially higher than those for a commonly used p-type material α-NPD (95 °C. Structure is shown in Figure 3),14 indicating that the compounds show high stability in their amorphous state. The BDP 4b did not show perceivable Tg, but the amorphous state was secured by the X-ray diffraction measurement of the powder sample, whereas amorphous samples of BDPs 4a and 4c were not successfully prepared.
The carrier mobilities of the BDPs 4a–c were very high for their amorphous state (Figure 2). Time-of-flight (TOF) technique was applied for the vacuum deposited µm-thick amorphous film of BDPs. The BDPs 4a and 4b showed nondispersive transient currents for hole and no current for electron, indicating p-type noncarrier trap transportation. The hole mobility at room temperature of these compounds was 2 × 10–3 cm2/Vs for both compounds at an electric field of 2.5 × 105 V/cm with little electric field dependence (Figure 2a). Interestingly, the carbazole-substituted BDP 4c displayed transient currents for both hole and electron, i.e., ambipolar character,15 similar to the carbazole-substituted BDF (CZBDF) that we previously reported5 or commonly known 1,1′-di(N-carbazolyl)-4,4′-biphenyl (CBP).16 The hole and electron mobilities were 6 × 10–4 and 2 × 10–3 cm2/Vs, respectively, at an electric field of 2.5 × 105 V/cm.17
Based on the measurements above, we have revealed the advantageous character of BDPs for their application to OLEDs: transparency in the visible region, appropriate orbital energy (HOMO level for carrier injection from an indium–tin oxide (ITO) anode, and LUMO for electron-blocking ability), high electrochemical and thermal stability, and high carrier mobility. Taking advantage of these features, we applied the p-type BDPs 4a and 4b as a hole transport layer (HTL) in OLEDs. The application of ambipolar BDP 4c is under investigation and will be reported elsewhere. OLEDs have found practical uses as flexible, lightweight, and power-saving display and illumination devices.18,19 For further improvement of device performance in driving voltage and efficiency, development of new materials for various parts of these devices has been extensively explored.20
The OLEDs have a standard configuration (Figure 3a): ITO (anode)/PEDOT:PSS (hole injection layer)/HTL (45 nm)/Alq3 (emitting and electron transport layer; 60 nm)/lithium fluoride (LiF) (electron injection layer; 1 nm)/Al, where the PEDOT:PSS21 layer was fabricated by spin-coating, and the following layers by vacuum deposition. We fabricated OLEDs using α-NPD as a HTL as a reference. The OLED performance was evaluated for three standard criteria at a luminance of 1,000 cd/m2: driving voltage (V1000), luminance efficiency (η1000), and current efficiency (L/J1000). The data are summarized in Table 2. These devices showed green electroluminescence from Alq3, indicating that the carriers are effectively confined within the Alq3 layer. The driving voltage of the device using 4a was 7.0 V, which is 1.5 V lower than that of the reference device (i.e., 8.5 V for the α-NPD-based device), while that of the 4b-based device was the same as for the reference (8.5 V). The luminance and the current efficiencies were also significantly improved: η1000 = 0.81, 0.84, and 0.59 lm/W; L/J1000 = 1.80, 2.29, and 1.60 cd/A for 4a, 4b, and α-NPD, respectively. We attribute the improved performance of the BDP-based devices to their HOMO energy levels, which are suitable for carrier injection processes both from the anode and to Alq3, and for facile carrier transport because of their high carrier mobility.
In summary, we found that HABDPs serve as excellent amorphous materials with high stability. The carrier mobility measurement and application to OLEDs unambiguously demonstrated that BDP itself has hole-transporting ability. Incorporation of substituents would synergistically improve the device performance by tuning the material properties.
EXPERIMENTAL
Synthesis.
General. Proton nuclear magnetic resonance (1H NMR) and carbon nuclear magnetic resonance (13C NMR) spectra were recorded using a JEOL ECA-500 (500 MHz) NMR spectrometer. High-vacuum train sublimation (pressure below 30 mTorr) was performed using a P-100HT instrument (ALS Technology Company). Melting points of solid materials were determined using a Mel-Temp II capillary melting-point apparatus and are uncorrected. Mass spectra were obtained on a JEOL Accu TOF JMS-T100LC spectrometer. N,N′-HexBn-protected BDPs 2 were prepared according to our previously reported method.7
2,3,6,7-Tetraphenylbenzo[1,2-b:4,5-b′]dipyrrole (3a). To a suspension of 1,5-bis[(4-hexylphenyl)methyl]-2,3,6,7-tetraphenylbenzo[1,2-b:4,5-b′]dipyrrole (167 mg, 0.206 mmol) in anisole (1 mL) was added AlCl3 (440 mg, 3.30 mmol) at 0 °C. The mixture was stirred at 100 °C for 12 h. After cooling to room temperature, the reaction was quenched with H2O. The organic layer was separated and the aqueous layer was extracted with CH2Cl2. The combined organic layers were concentrated in vacuo. After dissolving in toluene, 2-propanol was added to precipitate. The precipitate was collected by filtration and washed with 2-propanol to afford the title compound as a yellow solid (66.7 mg, 0.145 mmol, 70%). Mp 340–341 °C. 1H NMR (500 MHz, THF-d8) δ 7.19–7.29 (m, 8H, ArH), 7.36 (t, J = 7.6 Hz, 4H, ArH), 7.48–7.50 (m, 8H, ArH), 7.55 (s, 2H, ArH). 13C NMR (125 MHz, THF-d8) δ 99.2, 114.0, 126.4, 127.7, 128.6, 129.04, 129.06, 129.13, 131.0, 134.7, 135.4, 135.9, 137.8. MS (APCI+): 461 (M+1). Anal. Calcd for C34H24N2: C, 88.67; H, 5.25; N, 6.08. Found: C, 88.43; H, 5.29; N, 5.90.
2,6-Bis(4-N,N-diphenylaminophenyl)-3,7-diphenylbenzo[1,2-b:4,5-b′]dipyrrole (3b). The reaction was conducted at 100 °C. Mp 344–345 °C. 1H NMR (500 MHz, THF-d8) δ 7.00–7.03 (m, 8H, ArH), 7.07 (d, J = 8.3 Hz, 4H, ArH), 7.14–7.18 (m, 116H, ArH), 7.23–7.28 (m, 12H, ArH), 7.35 (d, J = 7.5 Hz, 4H, ArH), 7.63 (s, 2H, ArH). 13C NMR (125 MHz, 1,1,2,2-tetrachloroethane-d2) δ 98.88, 113.62, 123.66, 123.85, 125.42, 126.35, 128.61, 128.80, 129.13, 129.49, 130.06, 131.10, 135.24, 135.41, 138.07, 147.67, 148.64. MS (APCI+): 795 (M+1). Anal. Calcd for C58H42N4: C, 87.63; H, 5.33; N, 7.05. Found: C, 87.33; H, 5.56; N, 6.86.
Typical procedure for palladium-catalyzed N-arylation: 1,2,3,5,6,7-Hexaphenylbenzo[1,2-b:4,5-b′]dipyrrole (4a). To a suspension of 2,3,6,7-hexaphenylbenzo[1,2-b:4,5-b′]dipyrrole (1.38 g, 3.00 mmol) in toluene (15 mL) were added Pd2(dba)3•CHCl3 (124 mg, 0.120 mmol), P(t-Bu)3 in toluene (0.24 mL, 1.0 M, 0.24 mmol), sodium tert-butoxide (1.15 g, 12.0 mmol), and iodobenzene (1.01 mL, 14.4 mmol). The mixture was stirred at 120 °C for 24 h. After cooling to room temperature, the reaction was quenched with H2O. The mixture was filtered and the precipitate was washed several times with toluene and EtOAc. The resulting crude solid was purified by high vacuum (pressure under 20 mTorr) train sublimation at 300–320 °C to afford the title compound as a yellowish white solid (1.01 g, 1.65 mmol, 55%). Mp: sublimation. 1H NMR (500 MHz, 1,1,2,2-tetrachloroethane-d2) 7.08–7.09 (m, 10H, ArH), 7.15 (t, J = 7.2 Hz, 2H, ArH), 7.23–7.26 (m, 10H, ArH), 7.30–7.35 (m, 8H, ArH), 7.59 (s, 2H, ArH). 13C NMR (125 MHz, 1,1,2,2-tetrachloroethane-d2) δ 99.0, 116.5, 125.7, 126.4, 126.8, 127.1, 127.7, 128.2, 128.5, 129.0, 130.3, 131.3, 132.3, 135,6, 136.4, 138.6, 139. MS (APCI+): 613 (M+1). Anal. Calcd for C46H32N2: C, 90.16; H, 5.26; N, 4.57. Found: C, 90.13; H, 5.39; N, 4.62.
1,5-Bis(4-N,N-diphenylaminophenyl)-2,3,6,7-tetraphenylbenzo[1,2-b:4,5-b′]dipyrrole (4b). Yield: 52%. Mp 360–361 °C. 1H NMR (500 MHz, THF-d8) δ 7.00–7.03 (m, 8H, ArH), 7.07 (d, J = 8.3 Hz, 4H, ArH), 7.14–7.18 (m, 116H, ArH), 7.23–7.28 (m, 12H, ArH), 7.35 (d, J = 7.5 Hz, 4H, ArH), 7.63 (s, 2H, ArH). MS (APCI+): 947 (M+1). Anal. Calcd for C70H50N4: C, 88.76; H, 5.32; N, 5.92. Found: C, 88.69; H, 5.60; N, 5.82.
1,5-Bis(carbazolyl)-2,3,6,7-tetraphenylbenzo[1,2-b:4,5-b′]dipyrrole (4c). Yield: 58%. Mp 410–411 °C. 1H NMR (500 MHz, CDCl3) δ 7.22–7.25 (m, 10H, ArH), 7.29–7.33 (m, 4H, ArH), 7.35–7.38 (m, 4H, ArH), 7.42–7.44 (m, 6H, ArH), 7.46–7.48 (m, 6H, ArH), 7.50–7.52 (m, 4H, ArH), 7.58–7.59 (m, 4H, ArH), 7.85 (s, 2H, ArH), 8.15–8.17 (m, 4H, ArH). MS (APCI+): 943 (M+1). Anal. Calcd for C70H46N4: C, 89.14; H, 4.92; N, 5.94. Found: C, 88.86; H, 5.03; N, 5.77.
2,6-Bis(4-N,N-diphenylaminophenyl)-1,3,5,7-tetraphenylbenzo[1,2-b:4,5-b′]dipyrrole (4d). Yield: 38%. Mp 363–364 °C. 1H NMR (500 MHz, THF-d8) δ 6.77 (d, J = 8.9 Hz, 4H, ArH), 6.94–7.00 (m, 16H, ArH), 7.14 (t, J = 7.5 Hz, 2H, ArH), 7.19–7.22 (m, 8H, ArH), 7.25–7.31 (m, 10H, ArH), 7.37–7.42 (m, 8H, ArH), 7.61 (s, 2H, ArH). 13C NMR (125 MHz, 1,1,2,2-tetrachloroethane-d2) δ 99.12, 116.68, 122.79, 123.91, 125.47, 126.39, 126.78, 127.23, 127.51, 128.94, 129.30, 129.80, 130.01, 130.93, 132.87, 136.80, 137.14, 138.53, 140.25, 147.73, 148.46. MS (APCI+): 947 (M+1). Anal. Calcd for C70H50N4: C, 88.76; H, 5.32; N, 5.92. Found: C, 88.80; H, 5.57; N, 5.91.
Property measurements. UV–visible absorption spectra were recorded using a JASCO V-570 spectrophotometer at a resolution of 0.5 nm. Spectroscopy-grade CH2Cl2 was used as a solvent. Ca. 10–5 M sample solutions in a 1-cm square quartz cell were used for the measurements. All measurements were conducted at room temperature.
Cyclic voltammetry (CV) measurements were conducted using a Hokuto Denko HZ-5000 voltammetric analyzer. All measurements were conducted at room temperature in a one-compartment cell under N2 gas, equipped with a glassy-carbon working electrode, a platinum wire counter electrode, and an Ag/Ag+ reference electrode (10 mM AgClO4 in 0.1 M Bu4NPF6).
Differential scanning calorimetry (DSC) was performed using a Netzsch DSC 204/F1 thermal analyzer. The glassy state sample was heated at a rate of 5 K min–1 under N2 gas, at a flow rate of 18 mL min–1.
Thermogravimetry-differential thermal analysis (TG-DTA) was performed using a Rigaku TG8120 analyzer. Temperature was raised at 10 K min–1 under N2 using Al2O3 as a reference.
Carrier mobility measurement using time-of-flight (TOF) technique. Films were prepared on ITO-coated (145 nm) glass substrates by vacuum sublimation under heating (3–4 × 10–4 Pa and 2–4 × 10–4 Pa for 4a and 4b, respectively), with an average deposition rate of 4.0 nm/s. An ITO-coated glass substrate was spaced at 100 mm from the sample crucible, and it was maintained at 25 °C. The thickness of the film obtained was 4.57 and 3.83 µm for 4a and 4b, respectively. After Al cathode deposition (80 nm), the device was encapsulated under nitrogen atmosphere. The vacuum deposition was performed using a Ulvac Kiko VPC-260 system. The TOF measurement was conducted using a Sumitomo TOF-401 instrument.
Device fabrication and evaluation. Compounds of analytical purity were further purified by train sublimation. All the other materials were commercially available and were used as purchased. The typical fabrication procedure for a phosphorescent green OLED is described for the device using either compound 4a or 4b as a hole-transporting material. An ITO-coated glass substrate treated by O3-plasma was used as the anode. A PEDOT:PSS (poly(3,4-ethylenedioxythiophene):poly(styrenesulfonate)) water dispersion was spin-coated into this substrate, it was dried at 120 °C, then it was annealed at 180 °C under nitrogen. Next, a HTL with the thickness of 45 nm was fabricated by vacuum deposition. An emitting layer was subsequently fabricated by vacuum deposition of Alq3 with the thickness of 60 nm. An ultrathin layer (1 nm) of LiF was deposited at the cathode interface to enhance electron injection. After Al cathode deposition (80 nm), the device was sealed by encapsulation with a fresh desiccant under nitrogen. The emissive area of the device was 2 × 2 mm2.
Electroluminescence spectra and luminance-voltage characteristics of the OLED devices were measured using a Precise Gauges EL 1003 instrument. All measurements were conducted under ambient atmosphere.
ACKNOWLEDGEMENTS
We thank financial support (Strategic Promotion of Innovative Research and Development Programs) from the Japan Science and Technology Agency (JST) for E.N. Financial support in part by Kakenhi for E.N. (No. 22000008) and H.T. (No. 20685005) is also acknowledged.
References
1. A. Fürstner, Angew. Chem. Int. Ed., 2003, 42, 3582; CrossRef D. O’Hagan, Nat. Prod. Rep., 2000, 17, 435; CrossRef F. Bellina and R. Rossi, Tetrahedron, 2006, 62, 7213; CrossRef S. Thirumalairajan, B. M. Pearce, and A. Thompson, Chem. Commun., 2010, 46, 179; I. S. Young, P. D. Thorntonb, and A. Thompson, Nat. Prod. Rep., 2010, 27, 1801. CrossRef
2. B. H. Lipshutz, Chem. Rev., 1986, 86, 795. CrossRef
3. H. Maeda, Eur. J. Org. Chem., 2007, 5313; CrossRef P. A. Gale, Acc. Chem. Res., 2011, 44, 216; CrossRef S. Fukuzumi, K. Ohkubo, F. D’Souza, and J. L. Sessler, Chem. Commun., 2012, 48, 9801. CrossRef
4. L.-X. Wang, X.-G. Li, and Y.-L. Yang, Reactive & Functional Polymers, 2001, 47, 125; CrossRef P.-L. T. Boudreault, A. Najari, and M. Leclerc, Chem. Mater., 2011, 23, 456; CrossRef N. Yanai, T. Uemura, M. Ohba, Y. Kadowaki, M. Maesato, M. Takenaka, S. Nishitsuji, H. Hasegawa, and S. Kitagawa, Angew. Chem. Int. Ed., 2008, 47, 9883. CrossRef
5. Benzodifurans: H. Tsuji, C. Mitsui, L. Ilies, Y. Sato, and E. Nakamura, J. Am. Chem. Soc., 2007, 129, 11902; CrossRef H. Tsuji, C. Mitsui, Y. Sato, and E. Nakamura, Adv. Mater., 2009, 21, 3776; CrossRef H. Tsuji, C. Mitsui, Y. Sato, and E. Nakamura, Heteroat. Chem., 2011, 22, 316; CrossRef C. Mitsui, H. Tanaka, H. Tsuji, and E. Nakamura, Chem. Asian J., 2011, 6, 2296; CrossRef C. Mitsui, H. Tsuji, Y. Sato, and E. Nakamura, Chem. Asian J., 2012, 7, 1443. CrossRef
6. Naphthodifurans: C. Mitsui, J. Soeda, K. Miwa, H. Tsuji, J. Takeya, and E. Nakamura, J. Am. Chem. Soc., 2012, 134, 5448. CrossRef
7. Benzodipyrroles: H. Tsuji, Y. Yokoi, C. Mitsui, L. Ilies, Y. Sato, and E. Nakamura, Chem. Asian J., 2009, 4, 655. CrossRef
8. Cinnolines: H. Tsuji, Y. Yokoi, Y. Sato, H. Tanaka, and E. Nakamura, Chem. Asian J., 2011, 6, 2005. CrossRef
9. H. Tsuji, G. Cantagrel, Y. Ueda, T. Chen, L.-J. Wan, and E. Nakamura, Chem. Asian J., 2013, 8, 2377; CrossRef H. Tsuji, K.-i. Yamagata, Y. Ueda, and E. Nakamura, Synlett, 2011, 1015; CrossRef M. Nakamura, L. Ilies, S. Otsubo, and E. Nakamura, Org. Lett., 2006, 8, 2803; CrossRef M. Nakamura, L. Ilies, S. Otsubo, and E. Nakamura, Angew. Chem. Int. Ed., 2006, 45, 944. CrossRef
10. L. Ilies, H. Tsuji, Y. Sato, and E. Nakamura, J. Am. Chem. Soc., 2008, 130, 4240; CrossRef L. Ilies, Y. Sato, C. Mitsui, H. Tsuji, and E. Nakamura, Chem. Asian J., 2010, 5, 1376.
11. H. Tsuji, K. Sato, L. Ilies, Y. Itoh, Y. Sato, and E. Nakamura, Org. Lett., 2008, 10, 2263; CrossRef H. Tsuji, K. Sato, Y. Sato, and E. Nakamura, J. Mater. Chem., 2009, 19, 3364; CrossRef H. Tsuji, K. Sato, Y. Sato, and E. Nakamura, Chem. Asian J., 2010, 5, 1294; T. Kojima, S. Furukawa, H. Tsuji, and E. Nakamura, Chem. Lett., 2014, 43, 676. CrossRef
12. T. Watanabe, A. Kobayashi, M. Nishiura, H. Takahashi, T. Usui, I. Kamiyama, N. Mochizuki, K. Noritake, Y. Yokoyama, and Y. Murakami, Chem. Pharm. Bull., 1991, 39, 1152. CrossRef
13. A. S. Guram, R. A. Rennels, and S. L. Buchwald, Angew. Chem., Int. Ed. Engl., 1995, 34, 1348; CrossRef J. P. Wolfe and S. L. Buchwald, J. Org. Chem., 1996, 61, 1133; CrossRef D. W. Old, M. C. Harris, and S. L. Buchwald, Org. Lett., 2000, 2, 1403. CrossRef
14. S. A. Van Slyke, C. H. Chen, and C. W. Tang, Appl. Phys. Lett., 1996, 69, 2160. CrossRef
15. A. Chaskar, H.-F. Chen, and K.-T. Wong, Adv. Mater., 2011, 23, 3876. CrossRef
16. M. H. Tsai, Y. H. Hong, C. H. Chang, H. C. Su, C. C. Wu, A. Matoliukstyte, J. Simokaitiene, S. Grigalevicius, J. V. Grazulevicius, and C. P. Hsu, Adv. Mater., 2007, 19, 862. CrossRef
17. The transient currents of both hole and electron were dispersive.
18. Y. Shirota, ‘Organic light emitting devices. Synthesis, properties and applications,’ ed. by K. Müllen and U. Scherf, Wiley-VCH, Weinheim, 2006; Y. Sato ‘Electroluminescence I,’ Vol. 64, ed. by G. Müller, Academic Press, San Diego, 2000, pp. 209–254; CrossRef Y. Sato, S. Ichinosawa, and H. Kanai IEEE J Select Top Quantum Electron, 1998, 4, 40.
19. C. W. Tang and S. A. VanSlyke, Appl. Phys. Lett., 1987, 51, 913. CrossRef
20. Y. Shirota and H. Kageyama, Chem. Rev., 2007, 107, 953; CrossRef Y. Shirota, ‘Organic Light Emitting Devices. Synthesis, Properties and Applications,’ ed. by K. Müllen and U. Scherf, Wiley-VCH, Weinheim, 2006, pp. 245–263; CrossRef C. Adachi and T. Tsutsui, ‘Organic Light-Emitting Devices. A Survey,’ ed. by J. Shinar, Springer, New York, 2004, pp. 43–69; CrossRef Y. Shirota, J. Mater. Chem., 2000, 10, 1. CrossRef
21. L. Groenendaal, F. Jonas, D. Freitag, H. Pielartzik, and J. R. Reynolds, Adv. Mater., 2000, 12, 481. CrossRef