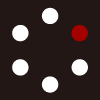
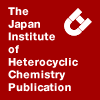
HETEROCYCLES
An International Journal for Reviews and Communications in Heterocyclic ChemistryWeb Edition ISSN: 1881-0942
Published online by The Japan Institute of Heterocyclic Chemistry
e-Journal
Full Text HTML
Received, 9th July, 2014, Accepted, 16th September, 2014, Published online, 26th September, 2014.
DOI: 10.3987/COM-14-S(K)81
■ Synthesis of 1,2-Dialkynyldisilanes Incorporated in 10-Membered-Ring System
Yoshiyuki Mizuhata, Yasunobu Egawa, Takahiro Sasamori, and Norihiro Tokitoh*
Division of Synthetic Chemistry, Organoelement Chemistry, Institute for Chemical Research, Kyoto University, Uji, Kyoto 611-0011, Japan
Abstract
The reduction of a bis(dichloroethynylsilane) bridged by o-xylylene by lithium naphthalenide afforded trans- and cis-isomers of novel 10-membered-cyclic disilanes. Their structures were characterized by X-ray crystallographic analysis, showing the strain around the C≡C bonds and the close distances between two ethynylene moieties.INTRODUCTION
Disilenes are silicon–silicon double-bond compounds, the first example of which was reported by West et al. in 1981.1 Since then, a number of disilenes that are kinetically stabilized by bulky substituents have been reported.2 Recently, several π-conjugated systems between the Si=Si unit and the connected aryl groups have been reported, which exhibited optically and electrochemically unique properties.3 From the viewpoint of elucidation of the π-conjugation between the Si=Si and C≡C units, we have succeeded in the synthesis and isolation of the first stable silicon analogues of (E)-enediyne, (E)-1,2-dialkynyldisilenes 1, by the reductive coupling of the corresponding dihalosilanes 2 (Scheme 1).4 The experimental and theoretical results indicated that the Si=Si unit conjugated with two alkynyl groups. On the other hand, (Z)-isomers are expected to show through-space interaction between two alkynyl groups in addition to the through-bonds interaction observed similarly in the (E)-isomers, resulting in the different electronic properties from those of (E)-isomers. However, the generation of the (Z)-1,2-dialkynyldisilenes could not be observed under those synthetic conditions. In order to synthesize the (Z)-isomers selectively, we attempted the introduction of o-xylylene bridge between two ethynyl units leading to the synthesis of (Z)-1,2-dialkynyldisilene 3 (Scheme 2).
RESULTS AND DISCUSSION
For the synthesis of disilenes, the reduction of dihalosilanes is known as one of the efficient methods.2 Actually, those methods were applicable toward the synthesis of 1, where the reduction of dichloro- or dibromosilanes 2 by lithium naphthalenide gave 1 in moderated yields. Accordingly, the bis(dichlorosilane) 4 was designed as the precursor of (Z)-dialkynyldisilene 3 (Scheme 2).
Bis(dichlorosilane) 4 was synthesized as shown in Scheme 3. At first, we attempted the reaction of dilithio compound 5,5 which was prepared by the treatment of 66 with n-butyllithium, with TipSiCl3 7.7 Although the formation of 4 was indicated by the results of 1H NMR measurement, the isolation of 4 from this reaction mixture was difficult due to its high sensitivity toward moisture. The attempted reaction of TipSi(OMe)3 8, which was prepared by the treatment of 7 with methanol in the presence of triethylamine, with 5 was unsuccessful leading to the recovery of the starting material. Partial chlorination of 8 by boron trichloride was found to give TipSiCl(OMe)2 9 as a main product along with ca. 10% of starting material 8 and TipSiCl2(OMe). The reaction of this mixture with 5 afforded bis(dimethoxysilane) 10 in 54% yield. Compound 10 had enough stability to be handled in the air and was purified by HPLC and recrystallization to be isolated. Following chlorination of 10 proceeded almost quantitatively to give 4 in 58% isolated yield (after recrystallization). Compound 4 was characterized by NMR spectroscopy together with elemental analysis, and the structure of 4 was finally determined by X-ray crystallographic analysis (Figure 1). The two TipSi(Cl2)C≡C– moieties were situated at opposite sides each other in the crystalline state.
The reduction of 4 was examined by using lithium naphthalenide under several conditions (various concentrations and ways of adding substrates). However, each reaction afforded a complicated mixture, the 29Si NMR spectra of which showed no signal characteristic of a disilene in low field region.2 HPLC analysis of the mixture suggested that the main component should be oligomeric materials. Finally, the 10-membered-cyclic products, trans- and cis-11, were isolated from the mixture in low yields. The yield of 11 was up to 11% by the method of simultaneous addition of 4 and lithium naphthalenide. On the contrary to our expectation, the chlorine atoms on the silicon atoms were fully replaced by the hydrogen atoms, which were probably derived from solvent and/or benzylic protons of 4.
The structures of trans- and cis-11 were shown in Figure 2, and the selected bond lengths and angles were summarized in Figure 3 together with the theoretically optimized structures for real molecules 11 and model compound 12 (Chart 1) bearing hydrogen atoms instead of Tip groups for comparison. The optimized structures calculated at M06-2X/6-31G(d) level showed good agreement with the observed ones, and their details were described later. In both cases of trans- and cis-11, the bridging o-xylylene units were found to flip toward C≡CSiSiC≡C cores. This trend was reproduced by the optimized structures of 11 and 12. In cis-isomer 11, interestingly, the o-xylylene moiety flipped toward the same side from C≡CSiSiC≡C core as two Tip groups probably due to the more condensed packing in the crystalline state. In the gas-phase calculations at M06-2X/6-31G(d) level, the energy difference between two configurations, cis-11a and cis-11b (Chart 1) was 0.0 kcal/mol. In the 1H NMR of trans-11, the signal assignable to the Si–H was observed as a singlet, suggesting their free flipping in solution at room temperature.
The structural parameters of 12 were similar to those of trans-11, suggesting the negligible steric effect in trans-11. On the other hand, those of cis-11, especially the distances between two ethynylene moieties, were different from those of trans-isomer, e.g., C1⋅⋅⋅C8: 3.345(4) (trans)/3.202(5) (cis) and C2⋅⋅⋅C7: 3.281(4) (trans)/3.182(5) (cis) Å. This is likely interpreted in terms of the steric repulsion between two Tip groups in cis-11 as follows. The C13–Si1–Si2/C28–Si2–Si1 angles in cis-11 [118.34(8)/121.37(9)º] were much larger than those in trans-11 [114.63(9)/108.06(9)º], these changes [Figure 4, arrow (a)] induced the movement (b) in Figure 4. As a result, Si2–Si1–C1 angle [99.99(9)º] in cis-11 was smaller than that in trans-11. While those distances were larger than the smallest values in cyclic 1,2-diethynyldisilanes structurally characterized so far [13 (Scheme 4),8 C1⋅⋅⋅C8: 3.155, C2⋅⋅⋅C7: 2.672 Å], those were one of the closest distances. The ethynylene moieties in trans- and cis-11 were highly strained, and the angles of Si–C1–C2/Si2–C8–C7 were 160.7(3)/164.6(3)˚ (trans) and 163.0(2)/168.7(3)˚ (cis), respectively. These values were within the range of the Si–C≡C angles of cyclic 1,2-diethynyldisilanes (smallest one is 155.8(1)/156.3(1)º observed in 13).
In order to estimate the ring strains in compounds 11 and 3, the calculations on isodesmic reactions of model compounds 12 and 14 were performed together with prop-2-yn-1-ylbenzene (Scheme 5) at M06-2X/6-31G(d) level. In both cases, the energy differences were 6-7 kcal/mol, which values were not so large and close to the strain energies for cyclopentane and cycloheptane.9 Judging from these results, the ring strains did not affect the low yield of the product very much.
Molecular orbitals of trans-11, cis-11a, and 12 were shown in Figure 5. In the model compound 12, the HOMO and LUMO apparently expanded to whole of the 10-membered ring and o-phenylene moiety. The HOMO showed the effective σ-π conjugation between the Si–Si bond and C≡C bonds, which corresponded to the HOMO–4’s in trans-11 and cis-11a. The dominant component of HOMOs of 11 was σ(Si–Si)-π(aryl) conjugation along with small contribution of ethynyl moieties.
As a result, novel 10-membered-cyclic disilanes 11 were found to have unique orbitals expanded to the whole rings. The introduction of Si=Si instead of Si–Si in 11 is expected to exhibit more effective π-electronic conjugation, and the transformation of compounds 11 into cyclic disilenes is now in progress.
EXPERIMENTAL
General procedures. All experiments were performed under an argon atmosphere unless otherwise noted. Solvents used for the reactions were purified by The Ultimate Solvent System (GlassContour Company).10 1H NMR (300 MHz), 13C NMR (76 MHz), and 29Si NMR (59 MHz) spectra were measured in C6D6 with a JEOL JNM-AL300 spectrometer. In 1H NMR, signal due to C6D5H (7.15 ppm) was used as references, and that due to C6D6 (128 ppm) was used in 13C NMR. Multiplicity of signals in 13C NMR spectra was determined by DEPT technique. 29Si NMR was measured with NNE and INEPT techniques using SiMe4 as an external standard. GPLC (gel permeation liquid chromatography) was performed on an LC-908, LC-918, or LC-908-C60 (Japan Analytical Industry Co., Ltd.) equipped with JAIGEL 1H and 2H columns (eluent: chloroform). All melting points were determined on a Yanaco micro melting point apparatus and were uncorrected. Elemental analyses were carried out at the Microanalytical Laboratory of the Institute for Chemical Research, Kyoto University. 1,2-Di(prop-2-yn-1-yl)benzene (6)6 and trichloro(2,4,6-triisopropylphenyl)silane (7)7 were prepared by following the procedures reported previously. All theoretical calculations were carried out using the Gaussian 0911 programs.
Synthesis of trimethoxy(2,4,6-triisopropylphenyl)silane (8). To mixture of MeOH (200 mL) and triethylamine (30 mL) was added a n-hexane solution (100 mL) of trichloro(2,4,6-triisopropylphenyl)silane 7 (10.7 g, 31.8 mmol) at 0 ºC. After stirring at 0 ºC for 1.5 h, the solvents were removed under reduced pressure. n-Hexane was added to the residue, and the resulting suspension was filtered through Celite® to remove inorganic salts to give 8 (9.94 g, 30.6 mmol, 96%). 8: viscous liquid; 1H NMR (300 MHz, C6D6, δ in ppm) 1.21 (d, J = 7.0 Hz, 6H), 1.36 (d, J = 6.7 Hz, 12H), 2.79 (sept, J = 7.0 Hz, 1H), 3.48 (s, 9H), 3.88 (sept, J = 7.0 Hz, 2H), 7.21 (s, 2H); 13C NMR (75 MHz, C6D6, δ in ppm) 24.0 (CH3), 25.6 (CH3), 33.0 (CH), 34.8 (CH), 50.3 (CH3), 121.6 (CH), 123.7 (C), 151.3 (C), 157.7 (C); 29Si NMR (59 MHz, C6D6, δ in ppm) –59.3; HRMS(FAB), m/z calcd for ([M–H]+): 323.2037, found: 323.2042.
Synthesis of 1,2-bis{3-[dimethoxy(2,4,6-triisopropylphenyl)silyl]prop-2-yn-1-yl}benzene (10). To a n-hexane solution (60 mL) of 8 (2.08 g, 6.42 mmol) was added boron trichloride (1.0 M heptane solution, 3.2 mL, 3.2 mmol) at 0 ºC, and the mixture was stirred at 0 ºC for 40 h. The solvents were removed under reduced pressure to give the mixture of chloro(dimethoxy)silane [TipSi(OMe)2Cl] 9 mainly with ca. 10% of starting material 8 and TipSiCl2(OMe). The mixture was used for the next reaction without further purification. To a THF solution (178 mL) of 1,2-di(prop-2-yn-1-yl)benzene 6 (407 mg, 2.60 mmol) was added n-butyllithium (1.61 M in n-hexane, 3.3 mL, 5.3 mmol) at –78 ºC, and the mixture was stirred at –78 ºC for 1 h. The resulting solution was added to a THF solution (25 mL) of the mixture containing 9 at 0 ºC, and the mixture was warmed up to room temperature overnight. The solvents were removed under reduced pressure. n-Hexane was added to the residue, and the resulting suspension was filtered through Celite® to remove inorganic salts. The reaction mixture was purified by GPLC (eluent: CHCl3) to afford 10 (1.53 g, 0.207 mmol, 54%). 9: 1H-NMR (300 MHz, C6D6, δ in ppm) 1.18 (d, J = 7.0 Hz, 6H) 1.33 (d, J = 6.7 Hz, 12H), 2.74 (sept, J = 7.0 Hz, 1H), 3.48 (s, 6H), 3.89 (sept, J = 6.7 Hz, 2H), 7.18 (s, 2H). 10: pale yellow viscous liquid; 1H NMR (300 MHz, C6D6, rt, δ in ppm) 1.21 (d, J = 7.0 Hz, 12H), 1.38 (d, J = 6.7 Hz, 24H), 2.77 (sept, J = 7.0 Hz, 2H), 3.38 (s, 4H), 3.61 (s, 12H), 4.15 (sept, J = 6.7 Hz, 4H), 6.98 (dd, 2H, J = 5.6, 3.5 Hz), 7.21 (s, 4H), 7.37 (dd, 2H, J = 5.6, 3.5 Hz); 13C NMR (75 MHz, C6D6, rt, δ in ppm) 23.9 (CH2), 24.0 (CH3), 25.4 (CH3), 32.9 (CH), 34.8 (CH), 50.9 (CH3), 84.1 (C), 104.4 (C), 121.7 (CH), 125.5 (C), 127.7 (CH), 128.1 (CH), 133.9 (C), 151.6 (C), 157.5 (C); 29Si NMR (59 MHz, C6D6, rt, δ in ppm) –42.9; HRMS (FAB) m/z calcd for C46H67O4Si2 ([M+H]+): 739.4578, found: 739.4577; Anal. Calcd for C46H66O4Si2: C, 74.74; H, 9.00%. Found: C, 74.81; H, 8.95%.
Synthesis of 1,2-bis{3-[dichloro(2,4,6-triisopropylphenyl)silyl]prop-2-yn-1-yl}benzene (4). To a n-hexane solution (150 mL) of 10 (938 mg, 1.26 mmol) was added boron trichloride (1.0 M heptane solution, 5.1 mL, 5.1 mmol) at 0 ºC, and the mixture was stirred at 0 ºC for 3.5 h. The solvents were removed under reduced pressure. Toluene was added to the residue, and the resulting suspension was filtered through Celite® to remove inorganic salts. The filtrate was re-precipitated from n-hexane to afford 4 (550 mg, 0.73 mmol, 58%). 4: colorless crystals; mp 124 ºC (decomp); 1H NMR (300 MHz, C6D6, rt, δ in ppm) 1.13 (d, J = 7.0 Hz, 12H), 1.27 (d, J = 6.7 Hz, 24H), 2.67 (sept, J = 7.0 Hz, 2H), 3.23 (s, 4H), 4.25 (sept, J = 6.7 Hz, 4H), 6.95 (dd, J = 5.7, 3.4 Hz, 2H), 7.12 (s, 4H), 7.22 (dd, J = 5.7, 3.4 Hz, 2H); 13C NMR (75 MHz, C6D6, rt, δ in ppm) 23.7 (CH3), 23.9 (CH2), 25.0 (CH3), 33.6 (CH), 34.7 (CH), 85.3 (C), 108.7 (C), 122.6 (CH), 124.9 (C), 128.0 (CH), 129.3 (CH), 132.9 (C), 153.5 (C), 156.8 (C); 29Si NMR (59 MHz, C6D6, rt, δ in ppm) –42.0; Anal. Calcd for C42H54Cl4Si2: C, 66.65; H, 7.33%. Found: C, 66.77; H, 7.33%.
Reduction of 4 (concentration of 4: 13 mM). To a solution of 4 (102 mg, 0.134 mmol) in THF (10 mL) was added lithium naphthalenide (0.29 M, 1.85 mL, 0.536 mmol) at –78 ºC. The reaction mixture was allowed to warm slowly to –5 ºC, where stirring was continued for 2.5 h. The solvent was removed under reduced pressure. Hexane was added to the residue and the resulting suspension was filtered through Celite® in order to remove all inorganic salts. The reaction mixture was separated by GPLC (CHCl3) to afford oligomeric products (88 mg) and the mixture of trans- and cis-11. The following PTLC (CH2Cl2:n-hexane = 1:1) gave trans-11 (3.1 mg, 0.0050 mmol, 3%) and cis-11 (n.d., <1%).
Reduction of 4 (concentration of 4: 1.3 mM). To a solution of 4 (99.4 mg, 0.131 mmol) in THF (100 mL) was added lithium naphthalenide (0.28 M, 1.9 mL, 0.532 mmol) at –78 ºC. The reaction mixture was allowed to warm up slowly to –5 ºC, where stirring was continued for 2.5 h. The solvent was removed under reduced pressure. n-Hexane was added to the residue and the resulting suspension was filtered through Celite® in order to remove all inorganic salts. The reaction mixture was separated by GPLC (CHCl3) to afford oligomeric products (51.8 mg) and the mixture of trans- and cis-11. The following PTLC (CH2Cl2:n-hexane = 1:1) gave trans-11 (2.4 mg, 0.0039 mmol, 3%) and cis-11 (4.7 mg, 0.0076 mmol, 6%).
Reduction of 4 (adding simultaneously). A 0.30 M THF solution (1.77 mL) of lithium naphthalenide (0.531 mmol) and a solution of 4 (100 mg, 0.132 mmol) in THF (1.77 mL) were added simultaneously from separate gas-tight syringes to 60 mL of THF at –78 ºC. The reaction mixture was allowed to warm slowly to –20 ºC, where stirring was continued for 4 h. The solvent was removed under reduced pressure. n-Hexane was added to the residue and the resulting suspension was filtered through Celite® in order to remove all inorganic salts. The reaction mixture was separated by GPLC (CHCl3) to afford oligomeric products (51.8 mg) and the mixture of trans- and cis-11. The following PTLC (CH2Cl2:n-hexane = 1:1) gave trans-11 (4.5 mg, 0.0073 mol, 6%) and cis-11 (4.2 mg, 0.0068 mmol, 5%).
Compound data for trans-11. colorless crystals; mp 151 ºC (decomp.); 1H-NMR (300 MHz, C6D6, rt, δ in ppm) 1.10 (d, J = 6.6 Hz, 12H), 1.16 (dd, J = 6.9, 1.3 Hz, 12 H), 1.32 (d, J = 6.6 Hz, 12 H), 2.72 (sept, J = 6.9 Hz, 2 H), 3.33 (d, J = 1.3 Hz, 4 H), 3.57 (sept, J = 6.6 Hz, 4H), 5.31 (s, 2H), 6.86-6.87 (m, 2H), 6.96-6.97 (m, 2H), 7.13 (s, 4H); 13C NMR (C6D6, rt, δ in ppm) 24.0 (CH3), 24.1 (CH3), 24.7 (CH3), 24.9 (CH3), 25.7 (CH2), 34.8 (CH), 34.9 (CH), 83.1 (C), 111.0 (C), 121.5 (CH), 125.3 (C), 127.9 (CH), 131.1 (CH), 135.8 (C), 151.2 (C), 156.7 (C); 29Si NMR (59 MHz, C6D6, rt, δ in ppm) –63.6; HRMS (FAB) m/z calcd for C42H55Si2 ([M–H]+): 615.3842, found: 615.3834.
Compound data for cis-11. colorless crystals; mp 153 ºC (decomp.); 1H NMR (300 MHz, C6D6, rt, δ in ppm) 0.99 (d, J = 6.5 Hz, 12H), 1.10 (d, J = 6.8 Hz, 12 H), 1.12 (d, J = 6.8 Hz, 12 H), 2.66 (sept, J = 6.8 Hz, 2 H), 3.15 (d, J = 17.1 Hz, 2H), 3.43 (d, J = 17.1 Hz, 2H), 3.46 (sept, J = 6.8 Hz, 4H), 5.51 (s, 2H), 6.85 (dd, 2H, J = 5.5, 3.4 Hz), 6.96 (dd, 2H, J = 5.5, 3.4 Hz), 6.99 (s, 4H). 13C NMR (75 MHz, C6D6, rt, δ in ppm) 23.9 (CH3), 24.3 (CH3), 24.7 (CH3), 26.0 (CH2), 34.5 (CH), 34.7 (CH), 84.1 (C), 112.4 (C), 121.5 (CH), 123.3 (C), 127.9 (CH), 130.9 (CH), 135.8 (C), 151.0 (C), 156.5 (C); 29Si NMR (59 MHz, C6D6, rt, δ in ppm) –65.5; HRMS (FAB) m/z calcd for C42H55Si2 ([M–H]+): 615.3842, found: 615.3846.
General procedure for X-ray crystallographic analysis of compounds 4, trans-11, and cis-11. The intensity data were collected on a Rigaku/MSC Mercury CCD diffractometer with graphite monochromated MoKα radiation (λ = 0.71070 Å). A single crystal suitable for X-ray analysis was mounted on a glass fiber. The structures were solved by a direct method (SHELXS-97)12 and refined by full-matrix least-squares procedures on F2 for all reflections (SHELXL-97).12 All hydrogen atoms except for Si–H were placed using AFIX instructions, while all other atoms were refined anisotropically. Deposition numbers CCDC-1012315 (4), 1012317 (trans-11), and 1012316 (cis-11). Free copies of the data can be obtained via http://www.ccdc.cam.ac.uk/conts/retrieving.html (or from the Cambridge Crystallographic Data Centre, 12 Union Road, Cambridge, CB2 1EZ, UK; Fax: +44 1223 336033; e-mail: deposit@ccdc.cam.ac.uk).
ACKNOWLEDGEMENTS
This work was supported by Grants-in-Aid for Scientific Research on Innovative Areas (Nos. 24109013, 23108707), Scientific Research (B) (Nos. 22350017, 25288021), Scientific Research (C) (No. 26410044), Young Scientist (B) (No. 21750042), Global COE Program (B09, “Integrated Materials Science”), Excellent Graduate Schools from the Ministry of Education, Culture, Sports, Science and Technology of Japan.
References
1. R. West, M. J. Fink, and J. Michl, Science, 1981, 214, 1343. CrossRef
2. M. Kira and T. Iwamoto, Adv. Organomet. Chem., 2006, 54, 73; CrossRef T. Sasamori and N. Tokitoh, in Encyclopedia of Inorganic Chemistry, ed. by R. B. King, Wiley, Chichester, UK, 2nd edn, 2005, p. 1698; M. Weidenbruch, in The Chemistry of Organic Silicon Compounds, ed. by Z. Rappoport and Y. Apeloig, Wiley, Chichester, UK, vol. 3, 2001, p. 391. CrossRef
3. I. Bejan and D. Scheschkewitz, Angew. Chem. Int. Ed., 2007, 46, 5783; CrossRef A. Fukazawa, Y. Li, S. Yamaguchi, H. Tsuji, and K. Tamao, J. Am. Chem. Soc., 2007, 129, 14164; CrossRef R. Kinjo, M. Ichinohe, A. Sekiguchi, N. Takagi, M. Sumimoto, and S. Nagase, J. Am. Chem. Soc., 2007, 129, 7766; CrossRef T. Sasamori, A. Yuasa, Y. Hosoi, Y. Furukawa, and N. Tokitoh, Organometallics, 2008, 27, 3325; CrossRef T. Iwamoto, M. Kobayashi, K. Uchiyama, S. Sasaki, S. Nagendran, H. Isobe, and M. Kira, J. Am. Chem. Soc., 2009, 131, 3156. CrossRef
4. T. Sato, Y. Mizuhata, and N. Tokitoh, Chem. Commun., 2010, 46, 4402. CrossRef
5. M. Ramming and R. Gleiter, J. Org. Chem., 1997, 62, 5821. CrossRef
6. C. M. Bowes, D. F. Montecalvo, and F. Sondheimer, Tetrahedron Lett., 1973, 14, 3181. CrossRef
7. F. Bickelhaupt and C. N. Smit, Organometallics, 1987, 6, 1156. CrossRef
8. G. Haberhauer, F. Rominger, and R. Gleiter, J. Chem. Soc., Perkin Trans. 2, 1999, 947. CrossRef
9. K. B. Wiberg, Angew. Chem., Int. Ed. Engl., 1986, 25, 312. CrossRef
10. A. B. Pangborn, M. A. Giardello, R. H. Grubbs, R. K. Rosen, and F. J. Timmers, Organometallics, 1996, 15, 1518. CrossRef
11. Gaussian 09, Revision A.02, M. J. Frisch, G. W. Trucks, H. B. Schlegel, G. E. Scuseria, M. A. Robb, J. R. Cheeseman, G. Scalmani, V. Barone, B. Mennucci, G. A. Petersson, H. Nakatsuji, M. Caricato, X. Li, H. P. Hratchian, A. F. Izmaylov, J. Bloino, G. Zheng, J. L. Sonnenberg, M. Hada, M. Ehara, K. Toyota, R. Fukuda, J. Hasegawa, M. Ishida, T. Nakajima, Y. Honda, O. Kitao, H. Nakai, T. Vreven, J. A. Montgomery, Jr., J. E. Peralta, F. Ogliaro, M. Bearpark, J. J. Heyd, E. Brothers, K. N. Kudin, V. N. Staroverov, R. Kobayashi, J. Normand, K. Raghavachari, A. Rendell, J. C. Burant, S. S. Iyengar, J. Tomasi, M. Cossi, N. Rega, J. M. Millam, M. Klene, J. E. Knox, J. B. Cross, V. Bakken, C. Adamo, J. Jaramillo, R. Gomperts, R. E. Stratmann, O. Yazyev, A. J. Austin, R. Cammi, C. Pomelli, J. W. Ochterski, R. L. Martin, K. Morokuma, V. G. Zakrzewski, G. A. Voth, P. Salvador, J. J. Dannenberg, S. Dapprich, A. D. Daniels, O. Farkas, J. B. Foresman, J. V. Ortiz, J. Cioslowski, and D. J. Fox, Gaussian, Inc., Wallingford CT, 2009.
12. G. M. Sheldrick, Acta Cryst., 2008, A64, 112. CrossRef