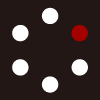
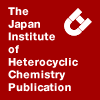
HETEROCYCLES
An International Journal for Reviews and Communications in Heterocyclic ChemistryWeb Edition ISSN: 1881-0942
Published online by The Japan Institute of Heterocyclic Chemistry
e-Journal
Full Text HTML
Received, 10th July, 2014, Accepted, 28th August, 2014, Published online, 11th September, 2014.
DOI: 10.3987/COM-14-S(K)83
■ Chiral Primary Amino Amide Alcohol Organocatalyst for the Asymmetric Michael Addition of 4-Hydroxycoumarin with α,β-Unsaturated Ketones
Jun Kumagai, Yoshihito Kohari, Chigusa Seki, Koji Uwai, Yuko Okuyama, Eunsang Kwon, and Hiroto Nakano*
Department of Applied Chemistry, Graduate School of Engineering, Muroran Institute of Technology, 27-1 Mizumoto, Muroran, Hokkaido 050-8585, Japan
Abstract
Chiral primary amino amide organocatalysts were designed and synthesized as new organocatalysts for the enantioselective Michael addition of 4-hydroxycoumarin with α,β-unsaturated ketones to produce chiral warfarin (up to 56% ee with up to 92% yield).INTRODUCTION
Asymmetric organocatalysis has emerged as an important and rapidly growing area of synthetic organic chemistry, and excellent covalent and non-covalent organocatalysts have been developed for use in a wide range of reactions.1 The asymmetric Michael addition is one of the most important and efficient
methods for C–C bond formation in organic synthesis,2 and the reaction of 4-hydroxycoumarin 1 with an α,β-unsaturated ketone, benzylideneacetone 2, using an organocatalyst is a straightforward method to access chiral warfarin 3 which is an effective and relatively safe agent for preventing thrombosis and embolism.3 Although several efficient organocatalyst4 for this reaction have been developed by several groups in recent years, it is difficult to obtain chiral warfarins with satisfactory high enantioselectivity. Therefore, it is still desirable and challenging to develop an effective catalytic system for this conversion. Chiral amine-based molecules, especially primary amine organocatalysts shows efficient asymmetric catalytic activity in this procedure. We designed a series of chiral primary amino amide alcohols 7a-n with one covalent site and two non-covalent sites in the molecule as an organocatalyst (Scheme 2). The reaction using these designed amino amide alcohol catalyst might proceed through the transition state X which is figured in Scheme 2. Since 4-hydroxycoumarins are fixed by the hydrogen bonding interactions with both the hydroxy group and the amide group on the imine intermediate, the coumarins might attack the less sterically crowded face of olefin part on the imine intermediate rather than the sterically crowded face that is masked by the combination of the bulky diphenyl group and the two substituents (R1 and R2) at the α and β positions on the imine intermediate.
We describe herein the catalytic ability of the newly designed primary amino amide alcohol organocatalyst for the asymmetric Michael addition of 4-hydroxycoumarins with α,β-unsaturated ketones affording chiral warfarins.
RESULTS AND DISCUSSION
Catalysts 7a-o bearing two substituents, aliphatic and/or aromatic group, at the α and β positions in the molecule were easily prepared by the condensation of N-Cbz-amino acids 4 with the corresponding amino alcohols 5a or amino silyl ether 5b in the presence of stoichiometric amounts of HOBt and EDC followed by the debenzyloxycarbonylation of the N-Cbz amino amide alcohols 6a-o using H2 and Pd-C (5%) at up to 98% yields, respectively (Scheme 3). We first examined the Michael addition of common
4-hydroxycoumarin 1 with benzylideneacetone 2 using the obtained amino amide alcohols 7a-n as organocatalysts (Table 1). The reaction of 1 (1 equiv) with 2 (1.2 equiv) was carried out at room temperature in THF (8 equiv) in the presence of 20 mol% of catalysts 7a-n and 20 mol% of additives to afford the chiral warfarin 3, respectively (entries 1-14). As a result of these reactions, all catalysts showed a catalytic activity and afforded warfarin 3 in moderate to fairly good chemical yields (56-91%, entries 1-14). However, satisfactory enantioselectivity was not observed under these reaction conditions. The best enantioselectivity was 43 % ee with 85% yield in the case of using the catalyst 7l which was bearing two iso-butyl moieties at α and β positions on the molecule (entry 12). The use of stereoisomer 7n (α: [S], β: [R]) of catalyst 7l (α: [S], β: [S]) caused a drop in the enantioselectivity (31% ee, entry 14), although the highest chemical yield (91%) was observed. The same reaction using amino amide silyl ether catalyst 7o in which the hydroxyl group was protected by a trimethylsilyloxy (TMS) group brought about a great decrease in the chemical yield and enantioselectivity (65%, 15% ee, entry 15) in comparison with those of the corresponding amino amide alcohol catalyst 7l with free hydroxy group (86%, 43% ee, entry 15). This difference may be due to the loss of the ability for hydrogen bonding to 4-hydroxycoumarin 1 or the steric influence of the bulkier trimethylsilyloxy group on the molecule, although the reasons are not clear. To further improve the enantioselectivity, the reactions using 7l were examined at lower temperatures of both 0 °C and -30 °C (entries 17 and 18). However, satisfactorily results were not observed under each temperatures for enantioselectivity and chemical yield. Next, to optimize the reaction conditions using the best catalyst 7l, we examined the effect of reducing the molar ratio of 7l. However, the decrease of catalytic loading of 7l to 10 mol% resulted in a substantial decrease in the chemical yield (46%) with a slight decrease in enantioselectivity (33% ee, entry 16). Furthermore, the effects of additives such as CF3CO2H, CCl3CO2H, and hexafluoroisopropyl alcohol (HFIP) in this reaction were investigated, respectively (entries 19-21). An additive has generally used for the improvement of the chemical yield and enantioselectivity in the Michael reaction for warfarin.4b,c,f,g,i,j,m Unfortunately, these additives did not work more effectively than MeCO2H and the chemical yields were significantly decreased under these reaction conditions. We examined the reactions of 1 (1 equiv) with 2 (1.2 equiv) carried out at room temperature in THF (8 equiv) in the presence of 20 mol% of catalyst 7l without any additive (entries 22-24). An increase in enantioselectivity was observed with good chemical yield (53%, 50% ee, entry 22). Furthermore, extending the reaction time from 24 to 72 h led to a great increase in the chemical yield (86%) with 52% ee (entry 26). After 7l was identified as effective
organocatalyst, we also examined solvent effects on this reaction. Commonly used both aprotic (Et2O, CH2Cl2, MeCN, benzene, toluene) and protic (EtOH, 2-PrOH) solvents were screened, respectively (entries 25-31). Only Et2O afforded a fairly good chemical yield (92%, entry 25), but other solvents gave chiral warfarin 3 in moderate to good yields (49-75%, entries 26-31). Unfortunately, no improvements in enantioselectivity were obtained when other catalysts 7a-k, m-o were used.
Under the optimized reaction conditions, a wide range of the Michael additions with 4-hydroxy- coumarins 1, 8a-d5 and α,βunsaturated ketones 2, 9a-h6 were investigated and the results are shown in Table 2. In all the Michael additions, catalyst 7l was performed to afford the corresponding chiral warfarins (up to 87%, up to 56% ee, entries 1-12). In particular, the reaction of 1 with bulkier aromatic 2-naphthylated α,β-unsaturated ketone 9g afforded the corresponding chiral warfarin 10g in moderate enantioselectivity and good chemical yield (56%, 56% ee, entry 7).
Based on the observed enantiopurity (52% ee, entry 24, Table 1) of chiral warfarin 3 that was obtained from the reaction of 1 with 2, a model of the enantioselective reaction under the condition without additives was proposed as follows (Scheme 4). The reaction might be go through the transition state A which is fixed by the two hydrogen bonding interactions of the hydroxy group on the imine intermediate and the carbonyl group on the 4-hydroxycoumarin 1 and of the carbonyl group on the imine intermediate and the hydroxy group on 1. Then 4-hydroxycoumarin might attack the si-face of the olefin part on the imine intermediate rather than the sterically crowded re-face that was masked by the combination of the bulky diphenyl group and the two iso-butyl groups on the imine intermediate in the transition state A.
In conclusion, new chiral amino amide alcohol organocatalysts 7a-o were explored. The catalysts were easily prepared from the condensation of cheaply and commercially available chiral amino acids with amino alcohols in two steps. The Michael addition of 4-hydroxycoumarins with α,β-unsaturated ketones using the explored catalyst 7l provided the corresponding Michael adduct 3 in moderate enantioselectivity (up to 56% ee) and fairly good chemical yield (up to 92%). Further optimizations of the structure of the catalyst 7 to increase enantioselectivity in this reaction are now in progress.
EXPERIMENTAL
IR spectra were measured with a JASCO FT/IR-400 spectrophotometer. 1H-NMR spectra were recorded on a JEOL JNM-ECA 500 spectrometer with TMS as an internal standard. MS were taken on Hitachi RMG-6MG and JEOL-JNM-DX 303 spectrometers. Optical rotations were measured with JASCO DIP-360 digital polarimeter.
General procedure for the preparations of amino amide alcohol organocatalysts 7a-n
A solution of N-Cbz amino acid 4 (1 mmol) and amino alcohol 5a (1 mmol) were dissolved in CH2Cl2 (10 mL). To the solution, EDC (1.4 mmol) and HOBT (1 mmol) was added and the solution was stirred at 0 oC for 1 h and room temperature for another 20 h. The reaction mixture was quenched with aqueous HCl (0.1 N) and extracted with AcOEt. The combined organic layer was washed with a saturated aqueous NaHCO3, brine, dried over anhydrous Na2SO4, and filterated. The filtrate was concentrated under reduced pressure to give the crude product which was used for the next step without further purification. To a solution of crude N-Cbz amino amide alcohols 6a-n in MeOH (10 mL) was added 5 % Pd/C (10 wt%) under hydrogen atmosphere and the mixture stirred at 50 oC for 24 h. The reaction mixture was filtered and the solvent was evaporated under reduced pressure to give the residue. The residue was purified by column chromatography on silica gel (AcOEt : hexane = 1 : 2) to afford the amino amide alcohol 7a-n. Compounds 7j-l7 were known compounds and were identified by spectral data which were in good agreement with those reported.
(S)-2-Amino-N-((S)-2-hydroxy-1,2,2-triphenylethyl)-2-phenylacetamide (7a)
White crystal (AcOEt), mp 188-189 oC, [α]D23 -27.3 (c 0.21, CHCl3). IR (neat) cm-1: 3304, 3058, 3029, 1636. 1H-NMR (CDCl3) δ: 7.55-6.93 (m, 20H), 6.01-5.98 (m, 1H), 4.37-4.36 (d, 1H, J = 6.6). 13C-NMR (CDCl3) δ: 172.2, 144.2, 137.4, 128.8, 128.6, 128.5, 128.4, 128.1, 127.9, 127.8, 127.5, 127.2, 127.0, 126.9, 126.0, 125.6, 80.9, 77.3, 77.1, 76.8, 59.9, 21.1, 14.2. Ms m/z: 422. HRMS (EI) calcd for (C28H26N2O2): 422.1994, Found: 422.1994. Anal. Calcd for (C28H26N2O2): C 79.59, H 6.20, N 6.63, O 7.57, Found: C 79.48, H 6.33, N 6.60, O 7.46.
(S)-2-Amino-N-((S)-1-hydroxy-3-methyl-1,1-diphenylbutan-2-yl)-2-phenylacetamide (7b)
White crystal (AcOEt), mp 153-154 oC, [α]D23 -24.5 (c 1.02, CHCl3). IR (neat) cm-1: 3309, 2960, 1644. 1H-NMR (CDCl3) δ: 7.48-7.15 (m, 15H), 4.82-4.80 (m, 1H), 4.35 (s, 1H), 0.87-0.58 (m, 6H). 13C-NMR (CDCl3) δ: 129.1, 128.9, 128.6, 128.5, 128.4, 128.2, 128.1, 127.0, 125.3, 125.2, 125.0, 81.9, 77.3, 77.1, 76.8, 67.0, 58.2, 28.8, 22.8, 17.5. Ms m/z: 388. HRMS (EI) calcd for (C25H28N2O2): 388.2151, Found: 388.2161.
(S)-2-Amino-N-((S)-1-hydroxy-1,1-diphenylpropane-2-yl)-2-phenylacetamide (7c)
White crystal (AcOEt), mp 150-151 oC, [α]D23 -15.0 (c 1.00, CHCl3). IR (neat) cm-1: 3297, 2942, 1652. 1H-NMR (CDCl3) δ: 7.48-7.02 (m, 15H), 4.99-4.93 (m, 1H), 4.31 (s, 1H), 1.16-1.15 (d, 3H, J = 6.6 Hz). 13C-NMR (CDCl3) δ: 144.4, 136.2, 128.9, 128.5, 128.3, 128.1, 127.2, 127.0, 125.5, 125.3, 80.3, 77.3, 77.1, 76.8, 67.0, 51.1, 16.1. Ms m/z: 360. HRMS (EI) calcd for (C23H24N2O2): 360.1838, Found: 360.1837.
(S)-2-Amino-N-((S)-2-hydroxy-1,2,2-triphenylethane)-3-phenylpropanamide (7d)
White crystal (AcOEt), mp 194-195 oC, [α]D22 -201.2 (c 0.83, CHCl3). IR (neat) cm-1: 3311, 2956, 1644. 1H-NMR (CDCl3) δ: 8.29-8.27 (d, 1H, J = 8.4 Hz), 7.71-6.01 (m, 20H), 6.03-6.01 (d, 1H, J = 9.2 Hz), 3.47-3.41 (m, 1H), 3.04-3.00 (m, 1H), 2.54-2.50 (m, 1H), 1.09 (brs, 2H). 13C-NMR (CDCl3) δ: 173.6, 144.5, 144.4, 137.8, 137.7, 129.3, 128.7, 128.3, 128.1, 127.9, 127.5, 127.2, 127.0, 126.7, 126.1, 125.7, 81.0, 77.3, 77.1, 76.8, 59.1, 56.3, 40.5. Ms m/z: 437 [M+H]+. HRMS (EI) calcd for (C29H28N2O2+H)+: 437.2229, Found: 437.2216. Anal. Calcd for (C29H28N2O2): C 79.79, H 6.46, N 6.42, O 7.33, Found: C 79.56, H 6.41, N 6.50, O 7.39.
(S)-2-Amino-N-((S)-1-hydroxy-3-methyl-1,1-diphenylbutan-2-yl)-3-phenylpropanamide (7e)
White crystal (AcOEt), mp 165-166 oC, [α]D21 -95.6 (c 0.93, CHCl3). IR (neat) cm-1: 3313, 2953, 1637. 1H-NMR (CDCl3) δ: 7.71-7.69 (d, 1H, J = 9.7 Hz), 7.69-7.04 (m, 5H), 4.82-4.80 (m, 1H), 3.43-3.41 (m, 1H), 3.07-3.04 (m, 1H), 2.48-2.39 (m, 1H), 0.89-0.85 (m, 6H). 13C-NMR (CDCl3) δ: 146.6, 145.6, 129.3, 128.8, 128.4, 128.2, 128.0, 126.9, 126.8, 125.6, 125.4, 81.9, 77.3, 77.1, 76.8, 56.6, 40.8, 28.8, 23.0, 18.2. Ms m/z: 403 [M+H]+. HRMS (EI) calcd for (C26H30N2O2+H)+: 403.2386, Found: 403.2397.
(S)-2-Amino-N-((S)-1-hydroxy-1,1-diphenylpropane-2-yl)-3-phenylpropanamide (7f)
White crystal (AcOEt), mp 167-168 oC; [α]D20 -85.8 (c 0.99, CHCl3). IR (neat) cm-1: 3325, 2970, 1644. 1H-NMR (CDCl3) δ: 7.60-7.59 (d, 1H, J = 8.9 Hz), 7.51-7.09 (m, 15H), 4.99-4.93 (m 1H), 3.38-3.36 (m, 1H), 3.04-3.00 (m, 1H), 2.38-2.32 (m, 1H), 1.15-1.13 (d, 3H, J =6.6 Hz). 13C-NMR (CDCl3) δ: 174.2, 145.5, 144.9, 137.9, 129.3, 128.7, 128.4, 128.2, 127.0, 126.9, 126.8, 125.8, 125.7, 80.6, 77.3, 77.1, 76.8, 56.3, 51.7, 40.7, 15.8. Ms m/z: 375 [M+H]+. HRMS (EI) calcd for (C24H26N2O2+H)+: 375.2073, Found: 375.2072.
(S)-2-Amino-N-((S)-1-hydroxy-1,1,2-triphenylethane)-3,3-dimethylbutanamide (7g)
White crystal (AcOEt), mp 210-211 oC, [α]D21 -215.5 (c 0.90, CHCl3). IR (neat) cm-1: 3325, 2969, 1645. 1H-NMR (CDCl3) δ: 7.75-7.73 (m, 1H), 7.62-6.61 (m, 2H), 7.37-7.06 (m, 13H), 6.09-6.04 (m, 1H), 2.96-2.95 (m, 1H), 0.99 (brs, 2H), 0.81 (s, 9H). 13C-NMR (CDCl3) δ: 172.9, 144.3, 128.8, 128.4, 128.1, 127.9, 127.5, 127.2, 127.1, 125.9, 125.5, 81.0, 77.3, 77.1, 76.8, 64.5, 59.1, 34.1, 26.6. Ms m/z: 402. HRMS (EI) calcd for (C26H30N2O2): 402.2307, Found: 402.2301.
(S)-2-Amino-N-((S)-1-hydroxy-3-methyl-1,1-diphenylbutan-2-yl)-3,3-dimethylbutanamide (7h)
White crystal (AcOEt), mp 240-241 oC, [α]D21 -70.7 (c 0.41, CHCl3). IR (neat) cm-1: 3422, 2969, 1647. 1H-NMR (CDCl3) δ: 7.55-7.04 (m, 10H), 5.08-5.00 (m, 1H), 2.80 (s, 1H), 0.87 (s, 9H), 0.83 (s, 6H). 13C-NMR (CDCl3) δ: 129.0, 128.2, 128.1, 126.7, 126.4, 126.1, 125.1, 124.7, 77.3, 77.1, 76.8, 65.0, 33.7, 29.9, 26.8. Ms m/z: 368. HRMS (EI) calcd for (C23H32N2O2): 368.2464, Found: 368.2448.
(S)-2-Amino-N-((S)-1-hydroxy-1,1-diphenylpropane-2-yl)-3,3-dimethylbutanamide (7i)
White crystal (AcOEt), mp 111-112 oC, [α]D22 -39.0 (c 0.87, CHCl3). IR (neat) cm-1: 3253, 2969, 1652. 1H-NMR (CDCl3) δ: 7.52-7.04(m, 10H), 5.06-5.00 (m, 1H), 2.92 (s, 1H), 1.52 (brs, 2H), 1.15-1.14 (d, 3H, J = 6.6 Hz), 0.78 (s, 9H). 13C-NMR (CDCl3) δ: 173.2, 153.3, 145.5, 145.0, 128.5, 128.3, 127.0, 126.9, 125.6, 125.5, 80.5, 77.3, 77.1, 76.8, 64.5, 51.6, 33.8, 26.5, 15.8. Ms m/z: 340. HRMS (EI) calcd for (C21H28N2O2): 340.2151, Found: 340.2162.
(S)-2-Amino-N-((S)-1-hydroxy-1,1-diphenylpropane-2-yl)-4-methylpentanamide (7m)
White crystal (AcOEt), mp 101-102oC, [α]D22 -34.6 (c 0.52, CHCl3). IR (neat) cm-1: 3308, 2969, 1644. 1H-NMR (CDCl3) δ: 7.51-7.13 (m, 10H), 4.99-4.92 (m, 1H), 3.16-3.14 (m, 1H), 1.16-1.15 (d, 3H, J = 6.6), 0.83-0.79 (m, 6H). 13C-NMR (CDCl3) δ: 175.6, 145.6, 145.0, 128.4, 128.2, 127.0, 126.8, 125.7, 125.6, 80.6, 77.3, 77.1, 76.8, 53.5, 51.8, 43.8, 24.7, 23.3, 21.4, 15.7. Ms m/z: 340. HRMS (EI) calcd for (C21H28N2O2): 340.2151, Found: 340.2136. Anal. Calcd for (C21H28N2O2): C 74.08, H 8.29, N 8.23, O 9.40, Found: C 74.01, H 8.34, N 8.17, O 9.31.
(S)-2-Amino-N-((R)-1-hydroxy-4-methyl-1,1-diphenylpentan-2-yl)-4-methylpentanamide (7n)
White crystal (AcOEt), mp 162-163 oC, [α]D23 -4.5 (c 0.22, CHCl3). IR (neat) cm-1: 3290, 2969, 1645. 1H-NMR (CDCl3) δ: 7.54-7.50 (m, 4H), 7.44-7.7.29 (m, 3H), 7.24-7.11 (m, 3H), 4.94-4.90 (m, 1H), 3.20-3.18 (m, 1H), 0.99-0.79 (m, 12H). 13C-NMR (CDCl3) δ: 175.9, 145.7, 145.1, 128.4, 128.1, 127.0, 126.6, 125.6, 81.3, 77.3, 77.1, 76.8, 54.3, 53.4, 43.6, 38.8, 25.2, 24.7, 24.0, 23.3, 21.6, 21.4. Ms m/z: 382. HRMS (EI) calcd for (C24H34N2O2): 382.2620, Found: 382.2630.
General procedure for the preparation of amino amide alcohol catalyst 7o
A solution of amino alcohol 5a (1 mmol) in CH2Cl2 (15 mL) was cooled down to -30 oC. To the solution, Et3N (1.2 mmol) and hexamethyldisilazane (1.2 mmol) were added and the reaction mixture was stirred at room temperature for 16 h. The solution was quenched with water and extracted with CHCl3. The combined organic layer was washed with a brine, dried over anhydrous Na2SO4, and filtrated. The filtrate was concentrated under reduced pressure to give the residue. The residue was purified by column chromatography on silica gel (AcOEt : hexane = 1 : 4) to afford (S)-4-methyl-1,1-diphenyl-1- trimethylsilyloxypentan-2-amine 5b. N-Cbz amino acid 4 (1 mmol) and (S)-4-methyl-1,1-diphenyl-1- trimethylsilyloxypentan-2-amine 5b (1 mmol) were dissolved in CH2Cl2 (10 mL) and the solution was cooled down to 0 oC. EDC (1.4 mmol) and HOBT (1 mmol) was added to the solution and the solution was stirred at 0 oC for 1 h and room temperature for another 20 h. The solution was quenched with aqueous HCl (0.1 N) and extracted with AcOEt. The combined organic layer was washed with a saturated aqueous NaHCO3, brine, dried over anhydrous Na2SO4, and concentrated under reduced pressure to give the crude product 6o which was used for the next step without further purification. The solution of 6o in MeOH (10 mL) was added 5 % Pd/C (10 wt%) under hydrogen atmosphere and the mixture stirred at 50 oC for 24 h. The reaction mixture was filtered and the solvents were evaporated under reduced pressure to give the residue. The crude residue was purified by column chromatography on silica gel (AcOEt : hexane = 1 : 2) to afford the amino amide silyl ether 7o.
(S)-4-Methyl-1,1-diphenyl-1- trimethylsilyloxypentan-2-amine (5b)
Colorless oil, [α]D21 -63.5 (c 0.21, CHCl3). IR (neat) cm-1: 1249, 2953. 1H-NMR (CDCl3) δ: 7.39-7.23 (m, 10H), 3.76-3.73 (m, 1H), 1.88-1.78 (m, 1H), 1.44-1.38 (m, 1H), 1.01-1.00 (d, 3H, J = 6.6 Hz), 0.84-0.82 (d, 3H, J = 6.6 Hz), -0.17 (s, 9H). 13C-NMR (CDCl3) δ: 128.7, 128.2, 127.6, 127.5, 127.4, 127.2, 83.5, 50.7, 40.8, 24.5, 24.1, 22.8, 22.1, 21.4, 1.8. Ms m/z: 341. HRMS (EI) calcd for (C24H34N2O2): 341.2175, Found: 341.2173.
(S)-2-Amino-N-((S)-4-methyl-1,1-dipheny-1-trimethylsilyloxypentan-2-yl)-4-methylpentanamide (7o)
White crystal (AcOEt), mp 75-76 oC; [α]D22 -83.3 (c 0.24, CHCl3). IR (neat) cm-1: 2969, 1669. 1H-NMR (CDCl3) δ: 7.41-7.20 (m, 10H), 5.09-5.00 (m, 1H), 3.24-3.19 (m, 1H), 1.03-0.72 (m, 12H), -0.12 (s, 9H). 13C-NMR (CDCl3) δ: 174.7, 144.5, 128.8, 128.2, 127.8, 127.5, 127.4, 127.1, 83.8, 77.3, 77.1, 76.8, 53.7, 51.7, 44.3, 41.0, 24.7, 24.3, 23.5, 22.0, 21.4, 1.9, 0.9. Ms m/z: 455 [M+H]+. HRMS (EI) calcd for (C24H34N2O2+H)+: 455.3094, Found: 455.3099.
General procedure for the asymmetric Michael reaction of 4-hydroxycoumarines 1, 8a-d to α,β-unsaturated ketones 2, 9a-h
4-Hydroxycoumarines 1, 8a-d (0.1 mmol), α,β-unsaturated ketones 2, 9a-h (0.12 mmol), amino amide alcohol catalysts 7a-o (0.02 mmol) with/without additives (0.02 mmol) were stirred in THF (0.8 mL) at room temperature for the times shown in the Table 1. The reaction mixture was directly purified by preparative TLC on silica gel (hexane : AcOEt = 3 : 1) to afford warfarin 3 and its analogs 10a-l. All adducts were known compounds and were identified by spectral data (1H and 13C-NMR) which were in good agreement with those reported.4 The enantiomeric excess (ee) were determined by HPLC (DAICEL CHIRALPAK AD-H, 1.0 mL/min, n-hexane : 2-propanol = 80 : 20).4
References
1. (a) B. List, Synlett., 2001, 1675; CrossRef (b) P. I. Dalko and L. Moisan, Angew. Chem. Int. Ed., 2004, 37, 580; (c) W. Notz, F. Tanaka, and C. F. Barbas III, Acc. Chem. Res., 2004, 37, 580; CrossRef (d) J. Seayad and B. List, Org. Biomol. Chem., 2005, 3, 719; CrossRef (e) H. Yang and R. G. Carter, Synlett, 2010, 2827.
2. (a) J. Christoffers, Eur. J. Org. Chem., 1998, 7, 1259; CrossRef (b) ‘Comprehensive Asymmetric Catalysis,’ Vol. I-III, ed. by E. N. Jacobsen, A. Pfaltz, and H. Yamamoto, Springer, Berlin, 1999; CrossRef (c) M. P. Sibi and S. Manyem, Tetrahedron, 2000, 56, 8033; CrossRef (d) N. Krause and A. Hoffmann-Roder, Synthesis, 2001, 171; CrossRef (e) O. M. Berner, L. Tedeschi, and D. Enders, Eur. J. Org. Chem., 2002, 1877; (f) J. Christoffers and A. Baro, Angew. Chem. Int. Ed., 2003, 42, 1688; CrossRef (g) R. Ballini, G. Bosica, D. Fiorini, A. Palmieri, and M. Petrini, Chem. Rev., 2005, 105, 933; CrossRef (h) L. W. Xu and C. G. Xia, Eur. J. Org. Chem., 2005, 633; CrossRef (i) D. Almasi, D. A. Alonso, and C. Najera, Tetrahedron: Asymmetry, 2007, 18, 299; CrossRef (j) J. L. Vicario, D. Badia, and L. Carrillo, Synthesis, 2007, 2065; CrossRef (k) S. Sulzer-Mossé and A. Alexakis, Chem. Commun., 2007, 3123; CrossRef (l) S. B. Tsogoeva, Eur. J. Org. Chem., 2007, 1701; CrossRef (m) C. F. Nising and S. Bräse, Chem. Soc. Rev., 2008, 37, 1218; CrossRef (n) P. M. Liu, C. Chang, R. J. Reddy, Y. F. Ting, H. H. Kuan, and K. Chen, Eur. J. Org. Chem., 2010, 42. CrossRef
3. (a) R. A. O’Railly, N. Engl. J. Med., 1976, 295, 354; CrossRef (b) L. B. Wingard, R. A. O’Railly, and G. Levy, Clin. Pharmacol. Ther., 1978, 23, 212; (c) H. P. Rang, M. M. Dale, J. M. Ritter, and P. Gardner, Pharmacology 4th ed., Churchill Livingstone, Philadelphia, 2001.
4. (a) N. Halland, T. Hansen, and K. A. Jørgensen, Angew. Chem. Int. Ed., 2003, 42, 4955; CrossRef (b) H. Kim, C. Yen, P. Preston, and J. Chin, Org. Lett., 2006, 8, 5239; CrossRef (c) J. W. Xie, L. Yue, W. Chen, W. Du, J. Zhu, J. G. Deng, and Y. C. Chen, Org. Lett., 2007, 9, 413; CrossRef (d) Z. H. Dong, L. J. Wang, X. H. Chen, X. H. Liu, L. L. Lin, and X. M. Feng, Eur. J. Org. Chem., 2009, 5192; CrossRef (e) T. E. Kristensen, K. Vestli, F. K. Hansen, and T. Hansen, Eur. J. Org. Chem., 2009, 5185; CrossRef (f) H. M. Yang, L. Li, K. Z. Jiang, J. X. Jiang, G. Q. Lai, and L. W. Xu, Tetrahedron, 2010, 66, 9708; CrossRef (g) H. M. Yang, Y. H. Gao, L. Li, Z. Y. Jiang, G. Q. Lai, C. G. Xia, and L. W. Xu, Tetrahedron Lett., 2010, 51, 3836; CrossRef (h) R. Q. Mei, X. Y. Xu, Y. C. Li, J. Y. Fu, Q. C. Huang, and L. X. Wang, Tetrahedron Lett., 2011, 14, 1566; CrossRef (i) J. Dong and D. M. Du, Org. Biomol. Chem., 2012, 10, 8125; CrossRef (j) Y. J. Lim and D. Y. Kim, Bull. Korean Chem. Soc., 2012, 33, 1825; CrossRef (k) S. K. Ray, P. K. Singh, N. Molleti, and V. K. Singh, J. Org. Chem., 2012, 77, 8802; CrossRef (l) T. Shi, Z. Guo, H. Yu, J. Xie, Y. Zhong, and W. Zhu, Adv. Synth. Catal., 2013, 355, 2538; CrossRef (m) M. Rogozińska-Szymczak and J. Mlynarski, Tetrahedron: Asymmetry, 2014, 25, 813. CrossRef
5. (a) P. R. Kym, R. Iyengar, A. J. Souers, J. K. Lynch, A. S. Judd, J. Gao, J. Freeman, M. Mulhern, G. Zhao, A. Vasudevan, D. Wodka, C. Blackburn, J. Brown, J. L. Che, C. Cullis, S. J. Lai, M. J. LaMarche, T. Marsilje, J. Roses, T. Sells, B. Geddes, E. Govek, M. Patane, D. Fry, B. D. Dayton, S. Brodjian, D. Falls, M. Brune, E. Bush, R. Shapiro, V. Knourek-Segel, T. Fey, C. McDowell, G. A. Reinhart, L. C. Preusser, K. Marsh, L. Hernandez, H. L. Sham, and C. A. Collins, J. Med. Chem., 2005, 48, 5888; CrossRef (b) K. A. Nolan, J. R. Doncaster, M. S. Dunstan, K. A. Scott, A. D. Frenkel, D. Siegel, D. Ross, J. Barnes, C. Levy, R. C. Whitehead, I. J. Stratford, and R. A. Bryce, J. Med. Chem., 2009, 52, 7142. CrossRef
6. X. Li, L. Li, Y. Tang, L. Zhong, L. Cun, J. Zhu, J. Liao, and J. Deng, J. Org. Chem., 2010, 75, 2981. CrossRef
7. (a) X. Y. Xu, Y. Z. Wang, and L. Z. Gong, Org. Lett., 2007, 9, 4247; CrossRef (b) C. S. Da, L. P. Che, Q. P. Guo, F. C. Wu, X. Ma, and Y. N. Jia, J. Org. Chem., 2009, 74, 2541. CrossRef