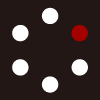
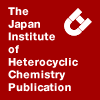
HETEROCYCLES
An International Journal for Reviews and Communications in Heterocyclic ChemistryWeb Edition ISSN: 1881-0942
Published online by The Japan Institute of Heterocyclic Chemistry
e-Journal
Full Text HTML
Received, 27th June, 2014, Accepted, 14th July, 2014, Published online, 16th July, 2014.
DOI: 10.3987/COM-14-S(K)48
■ Tandem [4+2] Cycloaddition/Aromatization Sequence of Allenyl 2-Bromo-3-vinylcyclohex-2-enyl Thioether to Naphtho[1,8-bc]thiophene
Noriyuki Hatae,* Aiichirou Kaji, Chiaki Okada, and Eiko Toyota
Faculty of Pharmaceutical Sciences, Health Sciences University of Hokkaido, Ishikari-Tobetsu, Hokkaido 061-0293, Japan
Abstract
A concise synthetic route of tetrahydro-3H-naphtho[1,8-bc]thiophene was developed. Allenyl 2-bromo-3-vinylcyclohex-2-enyl thioether underwent a tandem [4+2] cycloaddition/aromatization sequence to produce the naphthothiophene with high yield. A density functional study for the transition and reaction enthalpies was performed to elucidate the details of the reaction.Intramolecular pericyclic reactions of diene with dienophile provide an extraordinarily versatile method for the constructing polycyclic ring systems.1 In particular, using allenes as the dienophile in cycloaddition reactions leads to complex carbocyclic and heterocyclic frameworks, including natural products.2,3 Most recently, we have reported a switching of reaction pathway by C(2) substituent effects in the intramolecular cycloaddition reactions of allenyl 3-vinylcyclohex-2-enyl ethers generated in situ by base-catalyzed rearrangement of the corresponding propargyl ethers (Scheme 1).4 Steric and enthalpic effects of the C(2) substituent play an essential role in the switching of different cycloaddition processes; alkylated derivatives at the C(2) position provided [2+2] cycloaddition/[3,3]-sigmatropic rearranged products 2,5 whereas halogenated derivatives at the C(2) position yielded a [4+2] cycloaddition/aromatized product 3.4 It has also been reported that allenyl thioethers with or without alkyls at the C(2) position underwent intramolecular [2+2]/[3,3] or [4+2] cycloaddition reactions switched by their C(2) substituents; however, C(5) substituents were required for the reactions to progress owing to the increased stability and reactivity of the allenyl thioethers.6 The intramolecular cycloaddition reaction of alleny thioether with C(2)-halogen has encouraged more detailed studies of these types of cycloaddition reactions.
Potent bioactive furanosteroids, such as wortmannin, viridine, halenaquinone, and xestoquinone7 with a naphtho[1,8-bc]furan scaffold and the ergoline alkaloids, such as ergotamine and lysergic acid,8 containing benzo[cd]indole have been reported. Furans and pyrroles have been reported to be less aromatic and higher reactivity than thiophene in five-membered heterocycles.9 Replacement of the oxygen in the naphthofuran and the nitrogen in the benzoindole with sulfur could change the pharmacological actions of the furanosteroids and ergoline alkaloids and, in some cases, produce isosters with useful biological properties.10 However, a synthetic route of naphtho[1,8-bc]thiophene has been reported only by Clark and co-workers.11 A concise synthetic route of the naphtho[1,8-bc]thiophene ring system is required for the development of furanosteroid analogs and ergoline alkaloid analogs with good bioactivities. The aim of this work is to synthesize 4,6,7,8-tetrahydro-3H- naphtho[1,8-bc]thiophene (9) employing a tandem [4+2] cycloaddition/aromatization sequence of allenyl 2-bromo-3-vinylcyclohex-2-enyl thioether and to analyze the reaction mechanism in detail.
The synthetic route for allenyl 2-bromo-3-vinylcyclohex-2-enyl thioether (8) is shown in Scheme 2. The alcohol 5, which was prepared by a previously reported route,4 was treated with thioacetic acid in the presence of N,N-dimethylformamide dineopentyl acetal in toluene to give thioester 6 in 92% yield.12 Thioester 6 was subjected to ethanolysis in 0.2 mol/L KOH ethanolic solution, and then the propargylation
of crude thiol provided the requisite propargyl 2-bromo-3-vinylcyclohex-2-enyl thioether (7). Propargyl thioether 7 treated with aqueous NaOH (1 equiv.) in t-BuOH at 83 °C was isomerized to allenyl thioether 8, which was stably isolated without being hydrolyzed.
Upon treatment with t-BuOK in t-BuOH or THF, propargyl thioether 7 provided naphtho[1,8- bc]thiophene 9, which could be a cycloadduct of the corresponding allenyl thioether, with extremely low yield and was primarily recovered as unreacted propargyl thioethers (entries 1-4 in Table 1). The t-BuOK-mediated rearrangements of propargyl ethers to allenyl ethers have been reported to accelerate using THF as a solvent at room temperature,4,13 whereas propargyl thioether was hardly rearranged by t-BuOK in THF (entries 1 and 4 in Table 1). Refluxed propargyl thioether with aqueous NaOH in t-BuOH was able to rearrange quickly, corresponding to allenyl thioether 8 (Scheme 2).6,14 Using allenyl thioether 8 as starting material resulted in the synthesis of naphtho[1,8-bc]thiophene 9, whose chemical yields increased compared with the use of propargyl thioethers as starting materials (entries 5-7 in Table 1). Propargyl thioether did not undergo the cycloaddition reaction without a base (data not shown); the reaction occurred through an allenyl thioether intermediate. Apparently, the allenyl thioether under reflux conditions in toluene (110 °C) cyclized to tricyclic thiophene with high yield,15 although the chemical yield decreased significantly when refluxed in t-BuOH (83 °C). The cyclization reaction, which was conducted at 83 °C in toluene, gave only a 52% yield of thiophen (entry 6 in Table 1). Toluene might be better as a reaction solvent, but it is unclear about the effect of solvents in the reaction. Under all cyclization conditions, the reaction of allenyl 2-bromo-3-vinylcyclohex-2-enyl thioether led to a tandem [4+2] cycloaddition/aromatization reaction but not to a [2+2]/[3,3] reaction. These data were in
good agreement with Padwa and co-workers report that intramolecular [4+2] cycloaddition of halogenated furanyl amides proceeded at higher rates and increased chemical yields than the unsubstituted derivative.16 The tandem [4+2] cycloaddition/aromatization reaction of allenyl thioether required a higher temperature condition compared with allenyl ether reaction.
The potentials for compounds during cycloaddition were calculated using a density functional theory method at B3LYP/6-31G(d) level in Spartan 10.17,18 The results are shown in Table 2. Bromo-substituted analogs at the C(2) position increased the energy differences (ΔE) between s-cis and s-trans conformation and decreased the reaction enthalpies (ΔrH) for intramolecular [4+2] cycloaddition. For a Diels-Alder reaction of chlorofuran with ethylene, a decrease in reaction enthalpy by the induction of chlorine has been reported to increase reaction rates and yields due to stabilization of the resulting [4+2] cycloadducts.19 In the current cycloaddition of C(2) bromo substituted allenyl thioether, decrease of reaction enthalpy afforded the [4+2] cycloadduct but not the [2+2]/[3,3] product. A little shortening of the bond lengths between C(2) and C(2’) in bromo-substituted derivatives were observed for the [4+2] cycloadducts. One possible reason for the greater stabilization of the [4+2] cycloadducts of C(2) bromo derivatives with decreased reaction enthalpies could involve increased bond formation between bromo dienes and allenes as the dienophile. Furthermore, the higher transition state enthalpy of C(2) bromo allenyl thioether might require a higher reaction temperature relative to corresponding allenyl ethers.
In conclusion, 4,6,7,8-tetrahydro-3H-naphtho[1,8-bc]thiophene (9) was synthesized by a tandem [4+2] cycloaddition/aromatization sequence of allenyl 2-bromo-3-vinylcyclohex-2-enyl thioether (8). The bond formation between the bromo diene and allene could lead to decrease reaction enthalpy and promote the yield of the [4+2] cycloadduct.
ACKNOWLEDGEMENTS
This work was supported in part by a Grant-in Aid for Scientific Research by the Japan Society for the Promotion of Science (No. 23590143).
References
1. F. Fringuelli, A. Taticchi, and E. Wenkert, Org. Prep. Proced. Int., 1990, 22, 131. CrossRef
2. H. F. Schuster and G. M. Coppola, ‘Allenes in Organic Synthesis’, Wiley-Intersciences, New York, 1984.
3. T. Choshi, Yakugaku Zasshi, 2001, 121, 487. CrossRef
4. N. Hatae, I. Suzuki, T. Choshi, S. Hibino, C. Okada, and E. Toyota, Tetrahedron Lett., 2014, 55, 4146. CrossRef
5. K. Hayakawa, K. Aso, M. Shiro, and K. Kanematsu, J. Am. Chem. Soc., 1989, 111, 5312. CrossRef
6. S. K. Yeo, M. Shiro, and K. Kanematsu, J. Org. Chem., 1994, 59, 1621. CrossRef
7. J. R. Hanson, Nat. Prod. Rep., 1995, 12, 381. CrossRef
8. C. L. Schardl, D. G. Panaccione, and P. Tudzynski, Alkaloids Chem. Biol., 2006, 63, 45. CrossRef
9. B. S. Jursic, J. Heterocycl. Chem., 1996, 33, 1079. CrossRef
10. E. Campaigne, D. R. Knapp, E. S. Neiss, and T. R. Bosin, Adv. Drug Res., 1970, 5, 1.
11. P. D. Clark, N. M. Irvine, and P. Sarkar, Can. J. Chem., 1991, 69, 1011. CrossRef
12. The thioacetylation method was modified the procedure of acetylation of allylic alcohol: R. B. Barber and H. Rapoport, J. Med. Chem., 1975, 18, 1074. CrossRef
13. L. Brandsma and H. D. Verkruijsse, ‘Synthesis of acetylenes, allenes, and cumulenes: a laboratory manual’, Elsevier Scientific, New York, 1981.
14. K. Kanematsu and I. Kinoyama, J. Chem. Soc., Chem. Commun., 1992, 735. CrossRef
15. A typical procedure of the tandem [4+2] cycloaddition/aromatization reactions using 8 is as follows: To a solution of allenyl thioether 8 (212 mg, 0.82 mmol) in toluene (8.2 mL) was added pyridine (80 μL, 0.99 mmol) at room temperature under atmosphere of N2, and the mixture was then refluxed for 20 h. The reaction mixture was poured into water and extracted three times with t-BuOMe. The combined organic layers were washed with brine, dried over MgSO4, and concentrated under reduced pressure. The residue was then purified by column chromatography with hexane to give 4,6,7,8-tetrahydro-3H-naphtho[1,8-bc]furan (9) as a colorless oil in 94% yield.
16. K. R. Crawford, S. K. Bur, C. S. Straub, and A. Padwa, Org. Lett., 2003, 5, 3337. CrossRef
17. A. D. Becke, Phys. Rev. A: At. Mol. Opt. Phys., 1988, 38, 3098. CrossRef
18. C. T. Lee, W. Yang, and R. G. Parr, Phys. Rev. B: Condens. Matter, 1988, 37, 785. CrossRef
19. S. N. Pieniazek and K. N. Houk, Angew. Chem. Int. Ed., 2006, 45, 1442. CrossRef