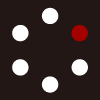
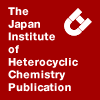
HETEROCYCLES
An International Journal for Reviews and Communications in Heterocyclic ChemistryWeb Edition ISSN: 1881-0942
Published online by The Japan Institute of Heterocyclic Chemistry
e-Journal
Full Text HTML
Received, 9th April, 2014, Accepted, 2nd May, 2014, Published online, 27th May, 2014.
DOI: 10.3987/COM-14-13003
■ Stereochemical Assignment of Four Diastereoisomers of 3,4-Dimethylpyroglutamic Acid, a Moiety of Callipeltin B
Mari Kikuchi and Hiroyuki Konno*
Graduate School of Science and Engineering, Yamagata University, 3-16 Jonan 4-Chome, Yonezawa 992-8510, Japan
Abstract
The synthesis and stereochemical assignment of four diastereoisomers of 3,4-dimethylpyroglutamic acid (pDME) (1) are described. Stereo-divergent synthesis of four pDMEs (1) was achieved starting from Thottathil’s synthon and Garner’s aldehyde for comparison of synthetic pDMEs (1) by 1H NMR analysis and CD spectra. The stereochemistry of pDME (1) in cyclic depsipeptide callipeltin B (2) with cytotoxic and anti-HIV activities was confirmed to be 2S,3S,4R. Coupling constant between H2 and H3 of synthetic (2S,3S,4R)-pDME (1a) showed a smaller value than those of other isomers. In addition, synthetic pDMEs (1) hardly showed cytotoxicity against HeLa cells.INTRODUCTION
3,4-Dimethylpyroglutamic acid (pDME) (1) is an unusual amino acid, including callipeltin B (2) isolated from marine sponge Callipelta sp.1 3,4-Dimethylglutamine (DMQ) (3), an acyclic unusual amino acid as a precursor of pDME (1), is a common component of callipeltin A (4),2 papuamide A,3 neamphamides,4 theopapuamides,5 mirabamides,6 homophymines7 and pipecolidepsin A.8 The stereochemistry of DMQ (3) was determined to be 2S,3S,4R on the basis of a positive Cotton effect on the CD spectrum and NMR analysis of the hydrolysate, which was pDME (1) derived from callipeltin A (4). Therefore, the stereochemistry of pDME (1) in callipeltin B (2) was also concluded to be 2S,3S,4R. Synthetic studies to construct unusual amino acids in the above-mentioned cyclic depsipeptides have been reported by several groups.9-14 In particular, synthesis of pDME (1a) is important research to achieve the total synthesis of complexed cyclic depsipeptides since DMQ (3) could be converted by a ring-opening reaction for pDME (1a).12 Preparation of DMQ and pDME derivatives has been reported independently by Lipton’s and Hamada’s groups to date. Lipton et al. obtained pDME (1a) in 9 steps via cuprate addition and enolate alkylation, followed by kinetic epimerization of the methyl substituent starting from L-pyroglutamic acid (L-pGlu) (5).12 On the other hand, Hamada’s group chose a Thottathil’s synthon15 as the starting material for pDME (1a) and produced in 6 steps.13 For our research program of the synthesis of callipeltins and homophymines, we reported the preparation of L-N-methylalanine, L-N-methylglutamine, (2S,3R)-D-β- methoxytyrosine (βMOY), D-allothreonine and pDME (1a) for the solid phase synthesis of callipeltin B (2) in our previous works.16-19 However, 1H NMR spectral data of pDME (1), which was obtained on large-scale synthesis based on previous synthetic route starting from Garner’s aldehyde, and those of natural product1 were slightly different. Synthetic pDME (1a) derived from Garner’s aldehyde was simultaneously recognized as the equilibrium mixture. Because of the formal synthesis of pDME (1a) derived from Garner’s aldehyde in our previous report,19 the stereochemistry of pDME (1a) has been hardly investigated in detail. In addition, spectral data of 3,4-dimethylpyroglutaminol (7a) reported by us,19,20 which was the substrate of the final oxidation reaction for pDME (1a), were similar to those of Hamada’s report.13 This result might suggest that the stereochemistry of pDME (1) needed reassignment by comparison of all diastereoisomers since Lipton’s and Hamada’s groups synthesized only the natural form and spectral data for comparison of other diastereoisomers were not indicated. In contrast, Lipton’s group reported that callipeltin B (2) showed cytotoxicity against HeLa cells with IC50 = 98 μM and desmethoxycallipeltin B, in which substitution of D-Tyr for βMOY indicated IC50 = 128 μM.21 The results showed that a methoxy group of βMOY was not needed to show cytotoxicity. Furthermore, we supposed that dimethyl groups of pDME (1a) were essential for cytotoxicity in our previous study22 using callipeltin B analogues; however, evaluation of pDMEs (1) against the cell lines has never been reported. For this reason, we herein disclose the preparation of four possible diastereoisomers, reconfirmation of
the stereochemistry of the natural form, and evaluation of cytotoxicity against HeLa cells for synthetic pDMEs (1) (Figure 1).
RESULTS AND DISCUSSION
At first, we synthesized pDMEs (1a,b) using Thottathil’s synthon.15 Preparation of the requisite dimethyl compound 6 was already described in 7 steps starting from L-pGlu (5) by Hamada and co-workers and therefore verification of the stereochemistry of the starting material (6) was performed correctly. After the spectral data of the obtained 6 were found to be identical to those of Hamada’s group, cleavage of N, O-acetal of 6 using 9% TFA/CH2Cl2 proceeded smoothly to afford alcohol (7b) in quantitative yield. Direct epimerization of H4-proton and/or oxidation of the hydroxy group of 7b afforded the expected products in extremely low yield. Thereby, after the protection of 7b with TBS and Boc groups, treatment with NaHMDS at -80 °C gave a mixture of the desired isomerized product (8a) and substrate (8b) in a ratio of 3:1 in satisfactory yield. Separation of the mixture of 8a and 8b afforded pure 8a by column chromatography in 67% yield, which is a known product reported by Lipton’s group.12 Boc carboxylic acid (9a) derived from 8a was obtained in the Jones condition with CrO3/H2SO4 in acetone at 0 °C (deprotection of TBS group and oxidation) and subsequently, deprotection of the Boc group with 50% TFA/CH2Cl2 proceeded to give the desired (2S,3S,4R)-pDME (1a) according to Lipton’s procedures (Scheme 1).12 It was noted that treatment of 9a with neat TFA afforded an undesired product (1b) by epimerization of H4-proton. Similarly, (2S,3S,4S)-pDME (1b) as a single diastereomer was given from 8b using the Jones condition followed by cleavage of the Boc group in 68% yield over 2 steps. As a result, 1H, 13C NMR, CD, IR, mp, [α]D and MS spectral data of synthetic 1a resembled those of the natural product1 and Hamada’s group,13 and furthermore, an equilibrium mixture of synthetic 1a could hardly be observed. On the contrary, pDME (1a) described in our previous report was not identified. Therefore, we
synthesized pDME (1a) according to the previous route to reassign the spectral data of all compounds obtained from previous study.19 As a result, we focused on the reaction of diastereoselective hydrogenation of 10. Thereby, hydrogenation of olefin (10), which was obtained starting from serine derivatives in 7 steps, by H2/Pd-C in MeOH/AcOEt afforded a single product in quantitative yield. In contrast, Hanessian has demonstrated that treatment of olefin (11) with Gilman reagent gave ethyl ester (12) in 98% yield at the high diastereoselectivity by internal 1,2-asymmetric induction.23-26 1H NMR spectral data of 12 derived from 11 by Hanessian’s protocol were clearly identical to the product obtained by hydrogenation of 10 in the present study (Scheme 2). Hanessian’s 1,4-addition reaction with Me2CuLi/TMSCl for 12 was proposed via Felkin-Ahn type conformation.27 We conjectured that 13 would be obtained due to the addition of hydrogen from the bottom at hydrogenation via similar conformation. However, both spectral data of 12 by Hanessian and our synthetic 13 in the previous literature19 conformed completely. In other words, two plausible reactions in Hanessian’s and our groups proceeded via distinct mechanisms to give the same compound (12). Kim et al. reported stereoselective dihydroxylation reaction of γ-amino-α,β-unsaturated esters via N-outside or N-inside conformers.28,29 According to these results, we assumed that the hydrogenation of E-olefin (10) proceeded via N-inside conformer to give 12 (Scheme 2).
The improved synthesis of other pDME isomers (1c,d) was carried out for comparison of spectral data. 12 was treated with NaHMDS and MeI in the presence of HMPA to provide the dimethylated product (14) in 92% yield as a single isomer. In the process of the purification of 14, pyrrolidinone (7c), transformed via deprotection of N, O-acetal, was afforded in trace amount. Therefore, treatment of 14 with SiO2 in toluene at reflux proceeded pyrrolidinone formation to afford 7c and its isomer (7d) in 54% yield at a ratio of 5:1 with amino lactone (15) as a byproduct. In an attempt to separate the mixture of 7c and 7d, we found that these isomers (7c,d) easily isomerized under neutral conditions at room temperature for a few hours. Spectral data of the mixture of 7c and 7d resembled those of 7a20 and 7b, thus determination of the natural form was extremely difficult. In our previous study, we concluded that alcohol (7) existed as a rotamer in the 1H NMR spectrum. Consequently, it was not easy to determine the natural form using 7a-d, although we have reported the synthesis of pDME (1a). Since TEMPO mediated oxidation using the mixture of 7c and 7d gave 1c and 1d in 77% yield at a ratio of 3:1, their coupling constants between H2 and H3-proton were indicated as JH2, H3 = 7.8~8.7 Hz, which did not conform with those of the natural compound and synthetic 1a or 1b. These results propose that the stereochemistry of 1c and 1d is 2S,3R,4R and 2S,3R,4S (Scheme 3).30 By measurement of the CD spectrum, 1a and the mixture of 1c and 1d showed a positive Cotton effect at 211 and 217 nm, respectively. These CD spectra were similar to those of the natural product having a positive Cotton effect at 208 nm. Because of the negative Cotton effect at 219 nm, 1b could be rejected completely.
Next, the molecular force field for four diastereoisomers of pDME (1) was calculated by SPARTAN. Four stable conformers were consequently indicated for each diastereoisomer and the energies of most stable conformations were calculated as -29.16, -30.09, -21.13 and -20.44 kJ/mol for 1a-d, respectively. On account of these results, the dihedrals of H3C3C2H2 of 1a-d were 93.5°, 153.6°, 30.8°and 35.7°. These coupling constants calculated by the Karplus equation confirmed that the observed value of the H2-proton of 1a was much smaller than other forms (Figure 2). We concluded that the stereochemistry of natural pDME (1) was 2S,3S,4R through the synthesis of four diastereoisomers and its simulation. (2S,3S,4R)-1a only indicated a small coupling constant between H2 and H3-protons at JH2, H3 = 3.2 Hz, and the other three isomers (1b-d) had similar coupling constants of JH2, H3 = 7.8~8.7 Hz. These results were confirmed by the comparison of 1H NMR spectral data of natural callipeltin B (2) (JH2, H3 = 3.0 Hz). In addition, 1a and 1b have never been observed in the equilibrium state in spite of the isomerized mixture of 1c and 1d with H4-proton in neutral conditions.
In our previous works on the cytotoxicity of callipeltin B analogues, we suggested that dimethyl groups of pDME (1) might be important for potent activities.22 A cytotoxicity assay was performed against HeLa cells using dimethyl and normal pyroglutamic acids, 1a-d, L-pGlu (5), D-pGlu (ent-5) for cytotoxicity alongside rotenone, which served as the control. The cytotoxicities of these compounds were determined by measuring live-cell succinate-tetrazolium reductase activity (MTT assay). The normal L- and D-pGlus (5, ent-5), pDMEs (1a, b), and the mixture of 1c and 1d showed practically no cytotoxicity. The results suggested that dimethyl groups of pDME (1) needed to be incorporated into the peptide, callipeltin B (2), for cytotoxicity against HeLa cells.
In conclusion, we prepared four diastereoisomers of pDMEs (1a-d) for the first time, and reconfirmation the stereochemistry of natural pDME (1) in callipeltin B (2) to be 2S,3S,4R. We supposed that 10 gave 12 by hydrogenation in high diastereoselectivity via N-inside conformer. Furthermore, 1H NMR spectral data of 7a-d were similar and consequently it was difficult to identify the stereochemistry of natural form pDME (1). However the comparison of all diastereomers of pDME (1) was efficient, especially using 1H NMR analysis and CD spectra. We revise the assignment of the stereochemistry of synthetic compounds in our previous research.19 In addition, cytotoxicity against HeLa cells was shown by the peptide with pDME (1). A synthetic study of callipeltin B (2) containing synthetic (2S,3S,4R)-pDME (1a) on a solid support is now underway.
EXPERIMENTAL
General. All solvents were reagent grade. CH2Cl2 was distilled from CaH2. All commercial reagents were of the highest purity available. Analytical TLC was performed on silica gel (Merck Silica gel 60 F254). Column chromatography was carried out on Silica Gel 60 N [Kanto, particle size, (spherical, neutral) 40-100 μm or 63-210 μm]. 1H (400 MHz) and 13C NMR (100, 75 MHz) spectra were recorded on either a JEOL JNM-ECX400 or a BRUKER AM-300. Chemical shifts are expressed in ppm relative to TMS (0 ppm), CHCl3 (7.26 ppm for 1H and 77.1 ppm for 13C) or H2O (4.79 ppm for 1H). IR spectra were obtained on a HORIBA FT-720 spectrometer. Optical rotations were recorded on a JASCO DIP-371 polarimeter at the sodium D line. High-resolution mass spectra (HRMS) were obtained using a JEOL AccuTOF JMS-T100LC (ESIMS) or a JMS-T100GC-EI (EIMS). Melting points were determined on an AS ONE ATM-02. Conformational searches and stable energy calculations were performed with Spartan’14 Parallel Suite for Windows (Wavefunction, Inc). Absorbances for cytotoxicity assay were measured with the CORONA MTP-310Lab. The CD spectra were recorded on a JASCO J-820.
(3S,4S,5S)-5-Hydroxymethyl-3,4-dimethylpyrrolidin-2-one (7b). To a solution of 6 (235 mg, 1.02 mmol) in CH2Cl2 (6 mL) was added TFA (600 μL). After the stirring for 1.5 h at room temperature, the mixture was evaporated under reduced pressure. The product was purified with silica gel column chromatography (CHCl3/MeOH=95:5) to give 7b (146 mg, 1.02 mmol, quant.) as a brown oil. [α]27D 45.8 (c 1.0, CHCl3); 1H NMR (CDCl3, 400 MHz) δ: 1.13 (3H, d, J=6.4 Hz), 1.14 (3H, d, J=7.2 Hz), 1.63 (1H, ddq, J=10.4, 8.4, 6.8 Hz), 2.08 (1H, dq, J=10.4, 7.2 Hz), 3.31 (1H, td, J=7.6, 2.4 Hz), 3.46 (1H, dd, J=11.6, 7.6 Hz), 3.76 (1H, d, J=11.6 Hz); 13C NMR (CDCl3, 100 MHz) δ: 13.9, 16.5, 40.0, 44.6, 62.4, 64.2, 180.6; IR νmax (film) cm-1: 3338, 2962, 2931, 2875, 1685; EIHRMS m/z [M]+: 143.0909, Calcd. for C7H13NO2: 143.0946.
(3S,4S,5S)-N-(tert-Butoxycarbonyl)-5-(tert-butyldimethylsilyloxy)methyl-3,4-dimethylpyrrolidin-2- one (8b). To a solution of 7b (288 mg, 2.01 mmol) in DMF (6 mL) was added TBSCl (603 mg, 4.02 mmol) and imidazole (545 mg, 8.04 mmol) and then the mixture was stirred at ~50 °C. After being stirred for 3.5 h, H2O and AcOEt were added to the solution. The organic layer was washed with brine, dried over MgSO4, and evaporated under reduced pressure. The residue was purified with silica gel column chromatography (CHCl3/MeOH=99:1) to give TBS ether (453 mg, 1.76 mmol, 88%) as a pale yellow oil. [α]28D 31.8 (c 0.4, CHCl3); 1H NMR (CDCl3, 400 MHz) δ: 0.05 (5H, s), 0.08 (1H, s), 0.87 (8H, s), 0.90 (1H, s), 1.13 (3H, d, J=7.2 Hz), 1.16 (3H, d, J=6.8 Hz), 1.55 (1H, m), 2.05 (1H, m), 3.27 (1H, td, J=8.2, 2.8 Hz), 3.38 (1H, dd, J=9.6, 8.7 Hz), 3.74 (1H, dd, J=10.1, 2.8 Hz), 5.87 (1H, brs); 13C NMR (CDCl3, 100 MHz) δ: -5.4, 14.2, 16.9, 18.2, 25.8, 40.6, 44.4, 61.4, 66.0, 179.2; IR νmax (film) cm-1: 3218, 3106, 2956, 2929, 2857, 1704, 1122, 839; ESIHRMS m/z [M+Na]+: 280.1708, Calcd. for C13H27NNaO2Si: 280.1709. To a solution of TBS ether (43.8 mg, 170 μmol) in MeCN (1 mL) was added (Boc)2O (371 mg, 1.70 mmol) and DMAP (cat.). After the stirring for 1.5 h at room temperature, H2O and AcOEt were added to the solution. The organic layer was washed with brine, dried over MgSO4, and evaporated under reduced pressure. The residue was purified with silica gel column chromatography (hexane/AcOEt=6:1) to give 8b (58.9 mg, 165 μmol, 97%) as a pale yellow solid. mp 50-52 °C; [α]26D -55.5 (c 2.7, CHCl3); 1H NMR (CDCl3, 400 MHz) δ: 0.03 (3H, s), 0.04 (3H, s), 0.87 (9H, s), 1.15 (3H, d, J=6.9 Hz), 1.22 (3H, d, J=7.4 Hz), 1.53 (9H, s), 1.96 (1H, m), 2.09 (1H, m), 3.54 (1H, td, J=4.1, 2.3 Hz), 3.69 (1H, dd, J=10.5, 1.8 Hz), 4.03 (1H, dd, J=10.6, 4.1 Hz); 13C NMR (CDCl3, 100 MHz) δ: -5.4, 14.8, 18.3, 18.6, 25.8, 28.1, 35.0, 45.4, 61.3, 64.4, 82.6, 150.5, 176.6; IR νmax (film) cm-1: 2958, 2931, 2857, 1789, 1754, 1716, 1340, 1201, 837; ESIHRMS m/z [M+H]+: 358.2451, Calcd. for C18H36NO4Si: 358.2414.
(3R,4S,5S)-N-(tert-Butoxycarbonyl)-5-(tert-butyldimethylsilyloxy)methyl-3,4-dimethylpyrrolidin-2- one (8a). To a solution of 8b (1.17 g, 3.28 mmol) in THF (15 mL) at -80 °C was added NaHMDS in THF (2.30 mL, 1.9 mol/L, 4.26 mmol) and then the mixture was stirred at -80 °C. After being stirred for 1 h, AcOH (377 μL) was added. The mixture was quenched by saturated NH4Cl aq. and diluted with Et2O. The solution was allowed to warm to room temperature and then extracted with Et2O. The organic layer was washed with brine, dried over MgSO4, and evaporated under reduced pressure. The residue was purified with silica gel column chromatography (hexane/AcOEt=8:1) to give 8a (786 mg, 2.20 mmol, 67%) as a pale yellow oil. [α]28D -43.5 (c 1.7, CHCl3); 1H NMR (CDCl3, 400 MHz) δ: 0.03 (3H, s), 0.05 (3H, s), 0.87 (9H, s), 0.99 (3H, d, J=7.2 Hz), 1.08 (3H, d, J=8.0 Hz), 1.54 (9H, s), 2.44 (1H, m), 2.97 (1H, m), 3.67 (1H, td, J=5.2, 2.8 Hz), 3.75 (1H, dd, J=10.8, 2.8 Hz), 3.85 (1H, dd, J=10.8, 5.2 Hz); 13C NMR (CDCl3, 100 MHz) δ: -5.6, 10.1, 15.7, 18.1, 25.8, 28.0, 32.9, 40.6, 63.0, 64.7, 82.6, 150.5, 176.7; IR νmax (film) cm-1: 2956, 2931, 2857, 1789, 1754, 1712, 1309, 1159, 837; ESIHRMS m/z [M+Na]+: 380.2212, Calcd. for C18H35NNaO4Si: 380.2233.
(2S,3S,4R)-N-(tert-Butoxycarbonyl)-3,4-dimethylpyroglutamic acid (9a). To a solution of 8a (317 mg, 887 μmol) in acetone (5 mL) at 0 °C was added Jones reagent (30% CrO3/H2SO4, 225 μL). After being stirred for 6.5 h at 0 °C, the reaction was quenched by addition of 2-propanol (225 μL) at 0 °C and saturated NaHCO3 aq. (125 μL) was added. The mixture was diluted with H2O and extracted with AcOEt. The aqueous layers were combined and acidified with 0.1 M HCl. The solution was extracted with AcOEt. The combined organic layers were washed with brine, dried over MgSO4, and evaporated under reduced pressure. The residue was purified with silica gel column chromatography (CHCl3/MeOH=98:2 to 95:5) to give 9a (199 mg, 773 μmol, 87%) as a colorless amorphous. mp 112-113 °C; [α]29D -8.0 (c 0.6, CHCl3); 1H NMR (CDCl3, 400 MHz) δ: 1.12 (3H, d, J=7.3 Hz), 1.13 (3H, d, J=7.3 Hz), 1.52 (9H, s), 2.58 (1H, m), 2.82 (1H, m), 4.24 (1H, d, J=1.8 Hz); 13C NMR (CDCl3, 100 MHz) δ: 9.9, 15.3, 27.9, 34.3, 40.7, 64.2, 83.9, 149.9, 175.7; IR νmax (film) cm-1: 3218, 2979, 2937, 2879, 1781, 1751, 1720, 1311, 1155; ESIHRMS m/z [M+Na]+: 280.1137, Calcd. for C12H19NNaO5: 280.1161.
(2S,3S,4R)-3,4-Dimethylpyroglutamic acid (1a). To a solution of 9a (49.5 mg, 192 μmol) in CH2Cl2
(2 mL) was added TFA (2 mL). After the stirring for 1.5 h at room temperature, the mixture was evaporated under reduced pressure to give 1a (30.2 mg, 192 μmol, quant.) as a white powder. mp 177-180 °C; [α]29D 48.3 (c 0.3, MeOH); CD [θ]211 nm +29728 (c 0.001 M, H2O); 1H NMR (D2O, 400 MHz) δ: 1.05 (3H, d, J=6.8 Hz), 1.13 (3H, d, J=6.8 Hz), 2.68 (2H, m), 3.96 (1H, d, J=3.2 Hz); 13C NMR (D2O, 100 MHz) δ: 9.5, 13.7, 38.0, 39.2, 61.4, 176.3, 183.8; IR νmax (film) cm-1: 3259, 2971, 1735, 1720, 1155; EIHRMS m/z [M]+: 157.0749, Calcd. for C7H11NO3: 157.0739.
(2S,3S,4S)-N-(tert-Butoxycarbonyl)-3,4-dimethylpyroglutamic acid (9b). To a solution of 8b (137 mg, 530 μmol) in acetone (2.5 mL) at 0 °C was added Jones reagent (30% CrO3/H2SO4, 100 μL). After being stirred for 3.5 h at 0 °C, the reaction was quenched by addition of 2-propanol (100 μL) at 0 °C and saturated NaHCO3 aq. (50 μL) was added. The mixture was diluted with H2O and extracted with AcOEt. The aqueous layers were combined and acidified with 0.1 M HCl. The solution was extracted with AcOEt. The combined organic layers were washed with brine, dried over MgSO4, and evaporated under reduced pressure. The residue was purified with silica gel column chromatography (CHCl3/MeOH=99:1 to 80:20) to give 9b (73.9 mg, 287 μmol, 76%) as a pale yellow oil. [α]23D -29.9 (c 0.7, CHCl3); 1H NMR (CDCl3, 400 MHz) δ: 1.24 (3H, d, J=7.3 Hz), 1.30 (3H, d, J=6.9 Hz), 1.50 (9H, s), 1.94 (1H, m), 2.21 (1H, m), 4.07 (1H, d, J=8.2 Hz); 13C NMR (CDCl3, 100 MHz) δ: 13.9, 17.2, 27.9, 38.8, 45.6, 64.4, 84.1, 149.4, 175.1; IR νmax (film) cm-1: 3219, 2978, 2937, 1778, 1753, 1724, 1155; ESIHRMS m/z [M+Na]+: 280.1175, Calcd. for C12H19NNaO5: 280.1161.
(2S,3S,4S)-3,4-Dimethylpyroglutamic acid (1b). To a solution of 9b (65.9 mg, 256 μmol) in CH2Cl2 (800 μL) was added TFA (800 μL). After the stirring for 2 h at room temperature, the mixture was evaporated under reduced pressure to give 1b (36.1 mg, 230 μmol, 90%) as a colorless amorphous. mp 129-130 °C; [α]24D -13.3 (c 0.4, MeOH); CD [θ]219 nm -26424 (c 0.001 M, H2O); 1H NMR (D2O, 400 MHz) δ: 1.06 (3H, d, J=7.3 Hz), 1.22 (3H, d, J=6.9 Hz), 2.03 (1H, m), 2.19 (1H, m), 3.88 (1H, d, J=8.2 Hz); 13C NMR (D2O, 100 MHz) δ: 13.5, 16.6, 42.7, 43.9, 61.2, 175.9, 182.9; IR νmax (film) cm-1: 3276, 2969, 2929, 1739, 1722, 1660; EIHRMS m/z [M]+: 157.0752, Calcd. for C7H11NO3: 157.0739.
(3R,4R,5S)-5-Hydroxymethyl-3,4-dimethylpyrrolidin-2-one (7c) and (3S,4R,5S)-5-hydroxymethyl- 3,4-dimethylpyrrolidin-2-one (7d). To a solution of 14 (195 mg, 647 μmol) in toluene (10 mL) was added SiO2 (2.0 g) and heated to reflux for 8 h. The mixture was filtrated, washed with 30% MeOH/CHCl3 and evaporated under reduced pressure. The residue was purified with silica gel column chromatography (CHCl3/MeOH=97:3 to 95:5) to give mixture of 7c and 7d (5:1, 50.4 mg, 352 μmol, 54%) as a brown oil. 13C NMR (CDCl3, 75 MHz) δ: 13.1, 13.8, 13.9, 16.4, 39.8, 40.0, 42.6, 44.4, 57.3, 58.4, 62.0, 62.1, 63.9, 180.4, 181.6; IR νmax (film) cm-1: 3385, 2962, 2931, 2877, 1685; EIHRMS m/z [M]+: 143.0944, Calcd. for C7H13NO2: 143.0946; 7c: 1H NMR (CDCl3, 400 MHz) δ: 1.11-1.14 (6H, m), 2.16 (2H, m), 3.55-3.76 (3H, m). 7d: 1H NMR (CDCl3, 400 MHz) δ: 1.13-1.15 (6H, m), 1.69-2.04 (2H, m), 3.31 (1H, m), 3.45 (1H, m), 3.79 (1H, m).
(2S,3R,4R)-3,4-Dimethylpyroglutamic acid (1c) and (2S,3R,4S)-3,4-dimethylpyroglutamic acid (1d). To a solution of mixture 7c and 7d (105 mg, 732 μmol) in MeCN (500 μL) and 0.5 M phosphate buffer (pH 6.6) (500 μL) was added NaClO (2 mL), NaClO2 (132 mg, 1.46 mmol) and TEMPO (16.3 mg, 105 μmol). After the stirring for 4.5 h at room temperature, 1 M HCl and AcOEt were added to the solution. The organic layer was washed with brine, dried over MgSO4, and evaporated under reduced pressure. The residue was purified with silica gel column chromatography (CHCl3/MeOH=95:5) to give mixture of 1c and 1d (3:1, 88.8 mg, 565 μmol, 77%) as a colorless oil. CD [θ]217 nm +21097 (c 0.001 M, H2O); 13C NMR (D2O, 100 MHz) δ: 14.3, 14.4, 14.6, 15.0, 41.2, 41.6, 43.0, 43.2, 69.2, 71.0, 173.9, 179.8, 180.0, 181.3; IR νmax (film) cm-1: 3417, 2972, 2939, 1732, 1716, 1213; EIHRMS m/z [M]+: 157.0730, Calcd. for C7H11NO3: 157.0739; 1c: 1H NMR (D2O, 400 MHz) δ: 1.07 (3H, d, J=7.7 Hz), 1.11 (3H, d, J=7.2 Hz), 2.27 (1H, m), 2.47 (1H, m), 4.37 (1H, d, J=8.7 Hz). 1d: 1H NMR (D2O, 400 MHz) δ: 1.14 (3H, d, J=6.9 Hz), 1.22 (3H, d, J=6.9 Hz), 2.11 (1H, m), 2.28 (1H, m), 4.04 (1H, d, J=7.8 Hz).
Chemical shift calculations. Synthetic pDMEs (1a-d) were submitted to a conformational search employing Molecular Mechanics to afford four stable conformers, respectively. The most stable conformer about each diastereomer was calculated the energies, dihedrals of H3C3C2H2 and chemical shifts.
Evaluation of the cytotoxicity by MTT assay. HeLa cells were maintained in a suspension culture of DMEM supplemented with 5% FBS (Fetal Bovine Serum) containing 1% of a penicillin-streptomycin mixture. A 100 μL aliquot of HeLa cells (5000 cells/mL) was added to a 96 well plate and incubated for 24 h at 37 °C in a humidified incubator containing 5% CO2 in air. After 24 h, a 10 μL aliquot of compound 1a, 1b, mixture of 1c and 1d, L-pGlu (5), D-pGlu (ent-5) (concentrations varying in the range of 5, 4, 3, 2, 1, 0.1 mM) and rotenone as a control was added to each of the 96 wells and incubated for 24 h. A 10 μL WST-8 solution (mixture of WST-8 and 1-Methoxy PMS) was added to each well and the incubation continued for 2 h. The visible absorbance at 450 nm and 630 nm as the reference wavelength of each well was quantified using a microplate reader.
ACKNOWLEDGEMENTS
This work was supported in part by a Grant-in aid from the Japan Society for the Promotion of the
Science (25450145) to H. K. and M. K. is grateful to the Japan Society for the Promotion of Science Fellowships for young scientists (8696).
SUPPORTING INFORMATION AVAILABLE
1H, 13C NMR, IR and MS spectra of 1a-d, and 7a-d and CD spectral data for 1a-d. This material associated with this article can be found in the online version.
References
1. M. V. D’Auria, A. Zampella, L. Gomez-Paloma, and L. Minale, Tetrahedron, 1996, 52, 9589. CrossRef
2. A. Zampella, M. V. D’Auria, L. Gomez-Paloma, A. Casapullo, L. Minale, C. Debitus, and Y. Henin, J. Am. Chem. Soc., 1996, 118, 6202. CrossRef
3. P. W. Ford, K. R. Gustafson, T. C. McKee, N. Shigematsu, L. K. Maurizi, L. K. Pannell, D. E. Williams, E. D. de Silva, P. Lassota, T. M. Allen, R. Van Soest, R. J. Andersen, and M. R. Boyd, J. Am. Chem. Soc., 1999, 121, 5899. CrossRef
4. N. Oku, K. R. Gustafson, L. K. Cartner, J. A. Wilson, N. Shigematsu, S. Hess, L. K. Pannell, M. R. Boyd, and J. B. McMahon, J. Nat. Prod., 2004, 67, 1407. CrossRef
5. A. S. Ratnayake, T. S. Bugni, X. Feng, M. K. Harper, J. J. Skalicky, K. A. Mohammed, C. D. Andjelic, L. R. Barrows, and C. M. Ireland, J. Nat. Prod., 2006, 69, 1582. CrossRef
6. A. Plaza, E. Gustchina, H. L. Baker, M. Kelly, and C. A. Bewley, J. Nat. Prod., 2007, 70, 1753. CrossRef
7. A. Zampella, V. Sepe, P. Luciano, F. Bellotta, M. C. Monti, M. V. D’Auria, T. Jepsen, S. Petek, M.-T. Adeline, O. Laprevote, A.-M. Aubertin, C. Debitus, C. Poupat, and A. Ahond, J. Org. Chem., 2008, 73, 5319. CrossRef
8. L. Coello, R. Fernandez, J. F. Reyes, A. Francesch, and M. D. C. Cuevas, Int. Appl. Pat., WO 2010/070078 A1, (24 June 2010).
9. R. Krishnamoorthy, L. D. Vazquez-Serrano, J. A. Turk, J. A. Kowalski, A. G. Benson, N. T. Breaux, and M. A. Lipton, J. Am. Chem. Soc., 2006, 128, 15392. CrossRef
10. J. P. Genet, S. Juge, and S. Mallart, Tetrahedron Lett., 1998, 29, 6765. CrossRef
11. M. Inoue, N. Shinohara, S. Tanabe, T. Takahashi, K. Okura, H. Itoh, Y. Mizoguchi, M. Iida, N. Lee, and S. Matsuoka, Nat. Chem., 2010, 2, 280. CrossRef
12. C. M. Acevedo, E. F. Kogut, and M. A. Lipton, Tetrahedron, 2001, 57, 6353. CrossRef
13. N. Okamoto, O. Hara, K. Makino, and Y. Hamada, Tetrahedron: Asymmetry, 2001, 12, 1353. CrossRef
14. S. Calimsiz and M. A. Lipton, J. Org. Chem., 2005, 70, 6218. CrossRef
15. J. K. Thottathil, J. L. Moniot, R. H. Mueller, M. K. Y. Wong, and T. P. Kissick, J. Org. Chem., 1986, 51, 3140. CrossRef
16. M. Kikuchi, K. Nosaka, K. Akaji, and H. Konno, Tetrahedron Lett., 2011, 52, 3872. CrossRef
17. H. Konno, S. Aoyama, K. Nosaka, and K. Akaji, Synthesis, 2007, 3666. CrossRef
18. M. Kikuchi and H. Konno, Tetrahedron, 2013, 69, 7098. CrossRef
19. H. Konno, Y. Takebayashi, K. Nosaka, and K. Akaji, Heterocycles, 2010, 81, 79. CrossRef
20. Treatment of 8a with TBAF followed by TFA gave 7a in moderate yield to use the comparison with other isomers. [α]23D 76.2 (c 0.2, CHCl3); 1H NMR (CDCl3, 400 MHz) δ: 1.01 (3H, d, J=6.9 Hz), 1.07 (3H, d, J=7.8 Hz), 2.25 (1H, m), 2.55 (1H, m), 3.33 (1H, brs), 3.49 (1H, td, J=10.1, 6.9 Hz), 3.74 (1H, d, J=10.5 Hz); 13C NMR (CDCl3, 100 MHz) δ: 10.7, 13.8, 34.5, 40.0, 62.4, 64.3, 181.9; IR νmax (film) cm-1: 3319, 2970, 2939, 2875, 1672; EIHRMS m/z [M]+: 143.0929, Calcd. for C7H13NO2: 143.0946.
21. R. Krishnamoorthy, B. L. Richardson, and M. A. Lipton, Bioorg. Med. Chem. Lett., 2007, 17, 5136. CrossRef
22. M. Kikuchi, Y. Watanabe, M. Tanaka, K. Akaji, and H. Konno, Bioorg. Med. Chem. Lett., 2011, 21, 4865. CrossRef
23. S. Hanessian, Y. Gai, and W. Wang, Tetrahedron Lett., 1996, 37, 7473. CrossRef
24. S. Hanessian, W. Wang, and Y. Gai, Tetrahedron Lett., 1996, 37, 7477. CrossRef
25. H. Yoda, T. Shirai, T. Katagiri, K. Takabe, K. Kimata, and K. Hosoya, Chem. Lett., 1990, 2037. CrossRef
26. C. Flamant-Robin, Q. Wang, and A. Sasaki, Tetrahedron Lett., 2001, 42, 8483. CrossRef
27. M. T. Reetz and D. Rohrig, Angew. Chem., Int. Ed. Engl., 1989, 28, 1706. CrossRef
28. J. Jeon, S.-K. Hong, J. S. Oh, and Y. G. Kim, J. Org. Chem., 2006, 71, 3310. CrossRef
29. J. S. Oh, J. Jeon, D. Y. Park, and Y. G. Kim, Chem. Commun., 2005, 770. CrossRef
30. Isomerization from γ-lactone (17) to 16 was not observed during a couple of months at room temperature. However, 7d gradually converted to 7c over several days to attain the equilibrium state at a ratio of 5:1.