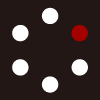
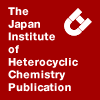
HETEROCYCLES
An International Journal for Reviews and Communications in Heterocyclic ChemistryWeb Edition ISSN: 1881-0942
Published online by The Japan Institute of Heterocyclic Chemistry
e-Journal
Full Text HTML
Received, 21st April, 2014, Accepted, 21st May, 2014, Published online, 4th June, 2014.
DOI: 10.3987/COM-14-13010
■ Selective Photocyclization Reactions of (Z)-N-Acetyl-α-dehydro(9-phenanthryl)alanine Alkyl Esters and N′,N′-Dialkylamides to Dibenzo[f,h]isoquinoline Derivatives
Yuto Iijima, Tetsutaro Igarashi, and Tadamitsu Sakurai*
Department of Material and Life Chemistry, Faculty of Engineering, Kanagawa University, 3-27-1 Rokkakubashi, Kanagawa-ku, Yokohama 221-8686, Japan
Abstract
(Z)-N-Acetyl-α-dehydro(9-phenanthryl)alanine alkyl esters and N′,N′-dialkylamides [(Z)-1] were synthesized, and their photocyclization behavior in methanol was explored. The irradiation of (Z)-1 in a protic polar solvent at wavelengths greater than 280 nm caused selective cyclization reactions, producing the corresponding dibenzo[f,h]isoquinoline derivatives. Moreover, the formation efficiency of these derivatives showed strong dependence on the steric bulk and electronic property of the alkoxycarbonyl and dialkylaminocarbonyl groups.Organic photochemistry has contributed to the development of efficient synthetic methods toward various heterocyclic compounds.1,2 In particular, because some of these compounds exhibit potent pharmacological activities, recent photochemical research has focused on diverse photocyclization and photocycloaddition reactions involving heteroatom-containing organic compounds.2 Our systematic study of the photocyclization reactions of α-dehydroamino acid derivatives has revealed that N-acyl-α-dehydro(4-substituted phenyl)alaninamides in their excited states undergo novel cyclization reactions in methanol to afford the corresponding isoquinolines and 1-azetines despite a low formation efficiency.3 Moreover, the introduction of 1-naphthyl group instead of phenyl as the aryl chromophore in N-acetyl-α-dehydroarylalaninamides significantly decreased the relative compositions of the photoproducts bearing isoquinoline and 1-azetine chromophores because of the preferential formation of the corresponding benzo[f]quinolinone derivatives, affecting the photocyclization process.4 Interestingly, the replacement of the secondary amide chromophore in N-acetyl-α-dehydro(4-substituted phenyl)alanine N′-alkylamides by an alkyl ester chromophore selectively produced 3-alkoxycarbonyl-1-methylisoquinoline derivatives, albeit still at low formation efficiency.5 Because the isoquinoline ring is an important heterocyclic skeleton contained in various pharmacologically active natural and synthetic products,6 its synthesis through selective and efficient transformation of N-acetyl-α-dehydroarylalanine alkyl esters and N′-alkylamides is necessary but remains significantly challenging.
Recently, the introduction of a 9-phenanthryl group into the N-acetyl-α-dehydroarylalaninamide aryl moiety has unexpectedly been found to greatly enhance the efficiency of photocyclization to the corresponding dihydrodibenzoquinolinones, confirming the usefulness of the phenanthryl chromophore as a photofunctional group for increasing the cyclization performance of a given N-acyl-α-dehydroamino acid derivative.7 Moreover, it is likely that the presence of a tertiary amide or an alkyl ester group in the amide moiety of this derivative suppresses the quinolinone ring construction. In addition, naphthalene and phenanthrene rings, which are bulkier than benzene, may exert their electronic effects that inhibit 1-azetine derivative formation. Using these molecular design considerations for the selective and efficient production of substituted isoquinolines from N-acyl-α-dehydroarylalanine derivatives, (Z)-N-acetyl-α-dehydro(9-phenanthryl)alanine methyl ester [(Z)-1a], (Z)-N-acetyl-α-dehydro(9-phenanthryl)alanine tert-butyl ester [(Z)-1b], (Z)-N-acetyl-α-dehydro(9-phenanthryl)alanine N′,N′-dimethylamide [(Z)-1c], and (Z)-N-acetyl-α-dehydro(9-phenanthryl)alanine N′,N′-(pentan-1,5-diyl)amide [(Z)-1d] were designed and synthesized (Scheme 1). In the present study, the photoreactivity of (Z)-1a–d was investigated along with the composition of the (Z)-1-derived photoproducts.
As shown in Scheme 1, (Z)-2-methyl-4-(9-phenanthrylmethylene)-5(4H)-oxazolone [(Z)-2] was prepared by Knoevenagel-type condensation between 9-phenanthrenecarboxaldehyde and N-acetylglycine in acetic anhydride containing sodium acetate. Ring-opening reactions of the oxazolone derivative (Z)-2 in methanol and tert-butyl alcohol containing 1,8-diazabicyclo[5.4.0]-7-undecene (DBU) afforded alkyl ester derivatives (Z)-1a and (Z)-1b, respectively. On the other hand, amide derivatives (Z)-1c and (Z)-1d were synthesized from ring-opening reactions of (Z)-2 mediated by dimethylamine and piperidine, respectively.
To isolate the (Z)-1a-derived photoproducts and determine their structures, a nitrogen-saturated methanolic solution of the α-dehydro(9-phenanthryl)alanine methyl ester (4.0 x 10–3 mol dm–3, 500 mL) was irradiated for 4 h using a 400 W high-pressure Hg lamp (λ ≥ 280 nm) at room temperature (rt). The irradiated solution was evaporated to dryness in vacuo to give a residual solid, which was subjected to preparative thin layer chromatography on silica gel using 9:1 CHCl3/hexane (v/v) as a developing solvent. Standard workup provided 3-methoxycarbonyl-1-methyldibenzo[f,h]isoquinoline (3a, 45% yield) and the (E)-isomer of 1a (23% yield), along with minor amounts of unidentified products. Irradiation of (Z)-1b–d in nitrogen-saturated methanol under the same conditions as (Z)-1a caused the expected cyclization reactions, leading to the corresponding dibenzo[f,h]isoquinoline derivatives 3b–d as major products (Scheme 2). Attempts at isolating (E)-1b–d were not made.
To compare the photoreactivity of 1a–d and the selectivity of 3a–d, nitrogen-saturated methanolic solutions of (Z)-1a–d (4.0 x 10–3 mol dm–3, 10 mL each) were irradiated in parallel for 2 h using the same light source (Hg lamp, λ ≥ 280 nm). Reaction mixtures obtained after solvent removal were analyzed by 1H NMR spectroscopy to determine the compositions of (Z)-1a–d, (E)-1a–d, and 3a–d (Table 1). 1H NMR spectral data obtained after 2 h irradiation confirmed that the methyl ester (Z)-1a and the dialkylamides (Z)-1c and (Z)-1d generated minor amounts of byproducts through undesired side reactions, whereas no (Z)-1b-derived byproducts were detected, providing an accurate estimate of the conversion of 1 as a photoreactivity index and the 1H NMR composition of each compound.
These results showed that the alkyl ester and dialkylamide derivatives (Z)-1 underwent selective transformations into the corresponding dibenzo[f,h]isoquinolines 3 upon photoirradiation but their photoreactivity strongly depended on the steric bulk and electronic property of the alkoxycarbonyl and dialkylaminocarbonyl groups. Specifically, replacing the methyl group in the ester moiety of (Z)-1a by the bulkier tert-butyl group [(Z)-1b] increased the conversion of 1a by a factor of ca. 2. A similar steric effect on photoreactivity was observed when the piperidinocarbonyl group, which is bulkier than the dimethylaminocarbonyl group, was introduced into the acid amide moiety of (Z)-1c. In contrast, substituting the dialkylaminocarbonyl chromophore [(Z)-1c,d] for the alkoxycarbonyl group in (Z)-1a and (Z)-1b lowered the photoreactivity of these α-dehydroamino acid ester derivatives to a considerable extent. Compared to (Z)-1a, (Z)-1b presented stronger steric repulsion between the alkoxy and acetyl carbonyl groups, which may further shorten the average distance between the acetyl carbonyl carbon and the phenanthrene ring carbon at the 10-position, enhancing the photoreactivity of this tert-butyl ester derivative (Figure 1). Molecular modeling based on the MM2 calculations of energy-minimized conformations for (Z)-1a and (Z)-1b provided a reasonable rationale for this interpretation.
In addition, the alkoxycarbonyl group is known to have a higher electron-withdrawing ability than the dialkylaminocarbonyl group,8 providing an interpretation of the additional photoreactivity increase for acid ester derivatives (Z)-1a and (Z)-1b compared with acid amide derivatives (Z)-1c and (Z)-1d in terms of inductive effects. In particular, the strong electron-withdrawing effect of the alkoxycarbonyl group contributed to a reactivity enhancement of the N-acetylcarbonyl carbon toward cyclization at the 10-position of the phenanthrene ring.
As previously proposed,3,4b the (Z)-isomer of 1 in the excited state undergoes a cyclization reaction producing the biradical intermediate I in competition with the deactivation of this (Z)-isomer toward the ground-state (Z)- and (E)-isomers (Scheme 3). Hydrogen shift in intermediate I affords tetrahedral intermediate II, which is readily converted into dibenzo[f,h]isoquinoline derivative 3 by subsequent dehydration.
In conclusion, (Z)-N-acetyl-α-dehydro(9-phenanthryl)alanine alkyl esters and N′,N′-dialkylamides (Z)-1a–d gave their corresponding dibenzo[f,h]isoquinoline derivatives 3a–d through selective photocyclization reactions, which significantly depended on the steric bulk and electronic property of the alkoxycarbonyl and dialkylaminocarbonyl groups in the starting material. The analysis of substituent effects on the photoreactivity of (Z)-1 demonstrated that (Z)-N-acetyl-α-dehydro(9-phenanthryl)alanine tert-butyl ester produced an N-acyl-α-dehydroarylalanine derivative that enabled the selective and efficient construction of the isoquinoline ring without any byproduct formation.
EXPERIMENTAL
1H and 13C NMR spectra were obtained using a JEOL JNM-ECA500 spectrometer. Chemical shifts were determined using tetramethylsilane as an internal standard. Matrix assisted laser desorption/ionization time-of-flight (MALDI-TOF) mass spectra were recorded on a Shimadzu/Kratos TOF mass spectrometer. MM2 calculations were performed using the CAChe 5.0 for Windows software (Fujitsu Ltd, 2002). Methanol was purified according to the standard procedure and freshly distilled prior to use.9 All other chemicals were obtained from commercial sources at the highest grade available.
Synthesis of (Z)-4-(9-phenanthrylmethylene)-2-methyl-5(4H)-oxazolone [(Z)-2]
Compound 9-phenanthrenecarboxaldehyde (44 mmol) was slowly added to a solution of N-acetylglycine (54 mmol) and sodium acetate (36 mmol) in acetic anhydride (40 mL) at rt and the resulting mixture was stirred for 6 h at 80–85 °C. The mixture was cooled overnight in an ice bath and the formed solid was collected by suction filtration and rinsed with water, a small amount of cold ethanol, and hexane. The crude product was air-dried at rt and purified by reprecipitation from hexane–CHCl3 to give the oxazolone derivative (Z)-2 as a yellow solid in a 55% yield: mp 213.0–215.0 °C; 1H NMR (500 MHz, CDCl3) δ = 2.48 (3H, s), 7.62 (1H, dd, J = 6.8, 8.5 Hz), 7.69–7.75 (3H, m), 8.03 (1H, s), 8.04 (1H, d, J = 8.5 Hz), 8.28 (1H, d, J = 8.5 Hz), 8.67 (1H, d, J = 8.5 Hz), 8.76 (1H, d, J = 8.5 Hz), 8.99 (1H, s); 13C NMR (125 MHz, CDCl3) δ = 15.8, 122.6, 123.3, 123.7, 126.9, 127.0, 127.3, 127.5, 127.6, 128.6, 130.2, 130.3, 130.5, 131.1, 131.4, 133.6, 133.8, 167.7, 170.0.
Synthesis of (Z)-N-acetyl-α-dehydro(9-phenanthryl)alanine alkyl esters [(Z)-1a,b]
The 9-phenanthrylmethylene-substituted oxazolone (10 mmol) was dissolved in MeOH or tert-BuOH (60 mL) in the presence of DBU (10 mmol), and the resulting reaction mixture was heated under reflux for 2–4 h. The solution was evaporated to dryness in vacuo and the obtained residue was dissolved in CHCl3 (100 mL). The CHCl3 solution was rinsed with hydrochloric acid (3.0 mol dm–3, 50 mL) and water (50 mL ´ 2), dried over anhydrous sodium sulfate, and evaporated to dryness under reduced pressure. The crude product was purified by column chromatography on silica gel (70–230 mesh) using 2:1 hexane/EtOAc (v/v) as an eluent. The α-dehydro(9-phenanthryl)alanine methyl ester derivative (Z)-1a and tert-butyl ester derivative (Z)-1b were isolated in 87% and 40% yields, respectively.
Methyl (Z)-2-acetylamino-3-(9-phenanthryl)propenoate [(Z)-1a]: white solid; mp 223.5–225.0 °C; 1H NMR (500 MHz, DMSO-d6) δ = 1.85 (3H, s), 3.79 (3H, s), 7.59 (1H, s), 7.66–7.77 (4H, m), 7.94 (1H, s), 8.00 (1H, d, J = 7.4 Hz), 8.00 (1H, d, J = 7.4 Hz), 8.84 (1H, d, J = 7.4 Hz), 8.89 (1H, d, J = 7.4 Hz), 9.61 (1H, s); 13C NMR (125 MHz, DMSO-d6) δ = 22.2, 52.2, 122.8, 123.4, 124.9, 126.8, 127.1 (2C), 127.2, 127.6, 127.7, 129.0, 129.1, 129.7, 129.8, 129.9 (2C), 130.8, 165.4, 169.4. MALDI-TOF-MS m/z calcd for C20H17NaNO3: 342.12 [M + Na]+. Found: 342.36.
tert-Butyl (Z)-2-acetylamino-3-(9-phenanthryl)propenoate [(Z)-1b]: colorless oil; 1H NMR (500 MHz, DMSO-d6) δ = 1.52 (9H, s), 1.85 (3H, s), 7.50 (1H, s), 7.65–7.77 (4H, m), 7.93 (1H, s), 7.98 (1H, d, J = 8.0 Hz), 7.98 (1H, d, J = 8.0 Hz), 8.84 (1H, d, J = 8.5 Hz), 8.90 (1H, d, J = 8.5 Hz), 9.47 (1H, s); 13C NMR (125 MHz, DMSO-d6) δ = 22.2, 27.7 (3C), 80.7, 122.8, 123.4, 124.9, 125.8, 127.0, 127.05, 127.14, 127.5, 127.6, 128.9, 129.3, 129.7, 129.89, 129.90, 130.9, 131.4, 163.9, 169.2. MALDI-TOF-MS m/z calcd for C23H23NaNO3: 384.17 [M + Na]+. Found: 384.29.
Synthesis of (Z)-N-acetyl-α-dehydro(9-phenanthryl)alanine N′,N′-dialkylamides [(Z)-1c,d]
The 9-phenanthrylmethylene-substituted oxazolone (10 mmol) was dissolved in CHCl3 (50 mL) containing dimethylamine (50 wt% aqueous solution, 12 mmol) or piperidine (12 mmol), and the resulting reaction mixture was heated under reflux for 2 h. The CHCl3 solution was rinsed with water (25 mL x 2), dried over anhydrous sodium sulfate, and evaporated to dryness under reduced pressure. The residual solid was subjected to column chromatography on silica gel (70–230 mesh) using EtOAc as an eluent. The α-dehydro(9-phenanthryl)alanine N′,N′-dimethylamide derivative (Z)-1c and N′,N′-(pentan-1,5-diyl)amide derivative (Z)-1d were isolated in 50% and 67% yields, respectively.
(Z)-2-Acetylamino-N,N-dimethyl-3-(9-phenanthryl)propenamide [(Z)-1c]: white solid; mp 237.0–238.0 °C; 1H NMR (500 MHz, DMSO-d6) δ = 1.85 (3H, s), 2.93 (3H, s), 3.17 (3H, s), 6.51 (1H, s), 7.64–7.75 (4H, m), 7.94 (1H, s), 7.98–8.03 (2H, m), 8.83 (1H, d, J = 8.0 Hz), 8.89 (1H, d, J = 8.0 Hz), 9.54 (1H, s); 13C NMR (125 MHz, DMSO-d6) δ = 22.2, 34.7, 38.9, 115.0, 122.7, 123.3, 125.1, 126.8 (2C), 126.9, 127.0, 127.4, 128.8, 129.5, 129.6, 130.0, 130.2, 131.2, 133.2, 167.7, 168.5. MALDI-TOF-MS m/z calcd for C21H20NaN2O2: 355.15 [M + Na]+. Found: 355.34.
(Z)-2-Acetylamino-N,N-(pentan-1,5-diyl)-3-(9-phenanthryl)propenamide [(Z)-1d]: white solid; mp 228.0–229.0 °C; 1H NMR (500 MHz, DMSO-d6) δ = 1.51–1.59 (2H, m), 1.60–1.68 (4H, m), 1.86 (3H, s), 3.47–3.59 (2H, m), 3.60–3.74 (2H, m), 6.47 (1H, s), 7.64–7.75 (4H, m), 7.95 (1H, s), 7.98 (1H, d, J = 6.8 Hz), 8.02 (1H, d, J = 6.8 Hz), 8.83 (1H, d, J = 8.5 Hz), 8.89 (1H, d, J = 8.5 Hz), 9.53 (1H, s); 13C NMR (125 MHz, DMSO-d6) δ = 22.3, 24.3, 25.0 (2C), 42.3, 48.2, 114.2, 122.7, 123.3, 125.0, 126.85, 126.89, 127.0, 127.1, 127.5, 128.8, 129.5, 129.6, 130.0, 130.2, 131.2, 133.3, 166.4, 168.4. MALDI-TOF-MS m/z calcd for C24H24NaN2O2: 395.18 [M + Na]+. Found: 395.39.
Photoirradiation of (Z)-1a–d
To isolate the (Z)-1a–d-derived photoproducts and estimate their yields, a nitrogen-saturated methanolic solution of each α-dehydro(9-phenanthryl)alanine derivative (4.0 x 10–3 mol dm–3, 500 mL) was placed in a Pyrex vessel and irradiated for 4 h using a 400 W high-pressure Hg lamp (λ ≥ 280 nm) set in a Pyrex cooling jacket at rt (internal irradiation, Pyrex glass filter). The irradiated solution was evaporated to dryness under reduced pressure to give a residual solid, which was subjected to preparative thin layer chromatography on silica gel to isolate dibenzo[f,h]isoquinoline derivatives 3a–d and the (E)-isomer of 1a. A mixture of CHCl3/hexane or EtOAc/hexane was used as a developing solvent. Yields and spectroscopic data are shown below for isolated 3a–d and (E)-1a. Attempts at isolating (E)-1b–d were not made.
Compositions of reaction mixtures obtained by irradiation of (Z)-1a–d in MeOH were determined as follows. Nitrogen-saturated methanolic solutions of the (Z)-α-dehydro(9-phenanthryl)alanine derivatives (4.0 x 10–3 mol dm–3, 10 mL x 4) were placed in Pyrex test tubes and irradiated in parallel for 2 h using the same light source as above (Hg lamp, λ ≥ 280 nm). The parallel irradiation was conducted at rt using a carrousel irradiation apparatus immersed into a water bath (RIKO model RH400-10W). Irradiated solutions were evaporated to dryness in vacuo to afford residual solids, which were dissolved in DMSO-d6 for 1H NMR spectroscopy analysis.
3-Methoxycarbonyl-1-methyldibenzo[f,h]isoquinoline (3a): pale yellow solid; 52% yield; mp 156.0–157.0 °C; 1H NMR (500 MHz, DMSO-d6) δ = 3.20 (3H, s), 3.99 (3H, s), 7.74–7.86 (4H, m), 8.73 (1H, d, J = 8.0 Hz), 8.78–8.88 (3H, m), 9.07 (1H, s); 13C NMR (125 MHz, DMSO-d6) δ = 29.5, 52.4, 115.8, 123.6, 123.9, 124.6, 126.8, 127.10, 127.11, 127.7, 128.4 (2C), 128.7, 130.1, 130.8, 131.2, 136.5, 142.3, 156.8, 165.4. MALDI-TOF-MS m/z calcd for C20H15NaNO2: 324.12 [M + Na]+. Found: 324.29.
3-tert-Butoxycarbonyl-1-methyldibenzo[f,h]isoquinoline (3b): pale yellow solid; 85% yield; mp 123.0–124.0 °C; 1H NMR (500 MHz, DMSO-d6) δ = 1.67 (9H, s), 3.20 (3H, s), 7.74–7.86 (4H, m), 8.71 (1H, d, J = 8.0 Hz), 8.78–8.85 (3H, m), 8.98 (1H, s); 13C NMR (125 MHz, DMSO-d6) δ = 27.8 (3C), 29.6, 81.4, 115.3, 123.6, 123.8, 124.5, 126.1, 127.1, 127.2, 127.7, 128.3 (2C), 128.6, 130.1, 130.8, 131.1, 136.4, 143.8, 156.6, 164.1. MALDI-TOF-MS m/z calcd for C23H21NaNO2: 366.16 [M + Na]+. Found: 366.36.
3-Dimethylaminocarbonyl-1-methyldibenzo[f,h]isoquinoline (3c): pale yellow oil; 11% yield; 1H NMR (500 MHz, DMSO-d6) δ = 3.05 (3H, s), 3.11 (3H, s), 3.21 (3H, s), 7.74–7.85 (4H, m), 8.68 (1H, s), 8.73 (1H, d, J = 8.0 Hz), 8.83–8.88 (3H, m); 13C NMR (125 MHz, DMSO-d6) δ = 29.6, 34.9, 38.5, 113.3, 123.5, 123.8, 124.7, 127.0, 127.4, 127.9, 128.0, 128.08, 128.11, 128.2, 129.9, 130.7, 130.8, 136.8, 149.7, 155.4, 168.2. MALDI-TOF-MS m/z calcd for C21H18NaN2O: 337.14 [M + Na]+. Found: 337.43.
1-Methyl-3-piperidinocarbonyldibenzo[f,h]isoquinoline (3d): pale yellow oil; 15% yield; 1H NMR (500 MHz, DMSO-d6) δ = 1.50–1.59 (2H, m), 1.61–1.70 (4H, m), 3.21 (3H, s), 3.38–3.42 (2H, m), 3.68–3.72 (2H, m), 7.74–7.86 (4H, m), 8.66 (1H, s), 8.71 (1H, d, J = 6.8 Hz), 8.78–8.85 (3H, m); 13C NMR (125 MHz, DMSO-d6) δ = 24.1, 25.4, 26.0, 29.6, 42.3, 47.6, 113.1, 123.6, 123.8, 124.4, 124.8, 127.0, 127.5, 128.0, 128.08, 128.13, 128.2, 130.0, 130.7, 130.8, 136.8, 149.8, 155.5, 166.8. MALDI-TOF-MS m/z calcd for C24H22NaN2O: 377.17 [M + Na]+. Found: 377.45.
Methyl (E)-2-acetylamino-3-(9-phenanthryl)propenoate [(E)-1a]: white solid; 23% yield; 1H NMR (500 MHz, DMSO-d6) δ = 2.04 (3H, s), 3.41 (3H, s), 7.16 (1H, s), 7.57 (1H, s), 7.63 (1H, dd, J = 6.8, 7.5 Hz), 7.69 (1H, dd, J = 6.8, 7.5 Hz), 7.69 (1H, dd, J = 6.8, 7.5 Hz), 7.75 (1H, dd, J = 6.8, 7.5 Hz), 7.94 (1H, d, J = 8.2 Hz), 8.01 (1H, d, J = 8.2 Hz), 8.81 (1H, d, J = 8.2 Hz), 8.88 (1H, d, J = 8.2 Hz), 10.29 (1H, s); 13C NMR (125 MHz, DMSO-d6) δ = 22.6, 51.6, 117.9, 122.8, 123.3, 125.2, 126.1, 127.0, 127.06, 127.10, 127.13, 128.5, 129.5, 129.7, 130.3, 130.5, 131.0, 131.3, 165.0, 168.3. MALDI-TOF-MS m/z calcd for C20H17NaNO3: 342.12 [M + Na]+. Found: 342.41.
References
1. a) 'Photochemistry of Heterocyclic Compounds', ed. by O. Buchardt, Wiley-Interscience, New York, 1976; b) 'CRC Handbook of Organic Photochemistry and Photobiology', 3rd ed., Vol. 1, ed. by A. Griesbeck, M. Oelgemöller, and F. Ghetti, CRC Press, Boca Raton, 2012.
2. a) 'Synthetic Organic Photochemistry', ed. by W. M. Horspool, Plenum, New York, 1984; b) 'Synthetic Organic Photochemistry', ed. by A. G. Griesbeck and J. Mattay, Marcel Dekker, New York, 2005; c) A. G. Griesbeck, N. Hoffmann, and K. Warzecha, Acc. Chem. Res., 2007, 40, 128. CrossRef
3. H. Hoshina, K. Kubo, A. Morita, and T. Sakurai, Tetrahedron, 2000, 56, 2941. CrossRef
4. a) K. Maekawa, T. Igarashi, K. Kubo, and T. Sakurai, Tetrahedron, 2001, 57, 5515; CrossRef b) K. Maekawa, H. Kajiwara, Y. Iseya, T. Igarashi, and T. Sakurai, Heterocycles, 2003, 60, 637. CrossRef
5. H. Hoshina, H. Tsuru, K. Kubo, T. Igarashi, and T. Sakurai, Heterocycles, 2000, 53, 2261. CrossRef
6. a) 'Comprehensive Heterocyclic Chemistry', Vol. 1, ed. by A. R. Katritzky and C. W. Rees, Pergamon Press, Oxford, 1984; b) A. R. Katritzky and A. F. Pozharskii, 'Handbook of Heterocyclic Chemistry', 2nd ed., Pergamon Press, Oxford, 2000.
7. Y. Kawanishi, H. Yoshi-ie, T. Igarashi, and T. Sakurai, Heterocycles, 2012, 85, 1053. CrossRef
8. S. L. Murov, I. Carmichael, and G. L. Hug, 'Handbook of Photochemistry', 2nd ed., Marcel Dekker, New York, 1993, pp. 345–348.
9. J. A. Riddick, W. B. Bunger, and T. K. Sakano, 'Organic Solvents', 4th ed., Wiley, Chichester, 1986.