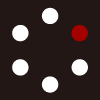
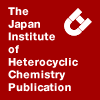
HETEROCYCLES
An International Journal for Reviews and Communications in Heterocyclic ChemistryWeb Edition ISSN: 1881-0942
Published online by The Japan Institute of Heterocyclic Chemistry
e-Journal
Full Text HTML
Received, 31st July, 2014, Accepted, 8th September, 2014, Published online, 11th September, 2014.
DOI: 10.3987/COM-14-S(K)99
■ Solution-Phase-Peptide Synthesis without Purification of Column Chromatography and Recrystallization by Protecting Amino Acid Esters with Phosphinyl Chloride
Guanghui An, Wei Zhou, Xiaokang Xu, Yi Pan, and Guigen Li*
Department of Chemistry & Biochemistry, Texas Tech University, Lubbock, TX 79409-1061, U.S.A.
Abstract
Biphenyl phosphinyl chloride (Bpp-Cl) has been successfully applied for the amino acid GAP (Group-Assisted Purification) protection. The resulting N-protected amino acid esters have been readily converted into the corresponding amino acids and peptides through GAP operation. Biphalin, enkephalin derivatives and the fragments of surfaxin have also been synthesized via GAP work-up by avoiding column chromatography. The GAP protecting group (Bpp) can be recovered and can implement the former phosphonyl groups in peptide synthesis.The market of pharmaceuticals of peptides and peptidomimetics has been growing rapidly in the past several decades. 1,2 So far, there have been approximately 60 peptide-based pharmaceutical products and over 600 more in different phases of clinical and preclinical trials.3 To accelerate the peptide drug discovery process, Merrifield established the pioneering work on solid-phase-peptide synthesis (SPPS),4 which has solved the tedious purification encountered at each step in solution phase peptide synthesis, although it still faces challenges such as scale-up, steric hindrance for longer peptides and resin expenses.5-7 For many cases, solution-phase-peptide synthesis cannot be completely replaced due to its faster rates, the use of less coupling reagents, easier production of longer peptides, less racemization and side-chain reactions, etc.6-9 To benefit from both SPPS and solution phase peptide synthesis, an alternative method was developed by using soluble polymers as the templates for peptide synthesis.10,11 This method enables the peptide synthesis to be performed in the solution phase but the work-up in a solid-phase manner, or, through convenient extractions. However, the latter method needs soluble polymers of high molecular weights, which makes it inconvenient to produce large amounts of peptides of much lower molecular weights than polymeric templates. In addition, it often needs long periods to generate solid or crystalline products by carefully controlling solidification/crystallization conditions. Obviously, the integration of these known methods would be anticipated to facilitate highly practical peptide synthesis.
Recently, we have developed a new concept called Group-Assisted Purification (GAP) chemistry12-15 which is to introduce well engineered functional groups onto reaction substrates for synthetic reactions; these functional group can result in solid products that can be purified simply by washing with common solvents or co-solvents without using column chromatography and recrystallization. The GAP chemistry has also been employed as a new strategy to for biphalin peptide synthesis successfully.15,16 This synthesis was started from O-phosphonyl protected tyrosine and followed a stepwise protocol in the N ? C direction. To extend the GAP synthesis of a larger scope of peptides, further study of converting N-phosphonyl amino acid esters to acids were thus carried out. Herein, we would like to report an N-phosphinyl protection group for peptide synthesis via stepwise protocol in the N ? C direction in GAP chemistry operation.
During the N ? C peptide elongation, the α-amino moiety of the initial amino acid was protected by N-phosphinyl group. More functionalized amino acids such as lysine and ornithine usually require additional protection. We envisioned that phosphinyl protecting group, as a GAP protection group,14 would show greater stability under cleavage conditions of methyl esters and benzyl esters as compared to its phosphonyl counterpart. Biphenyl phosphinyl group 1 (Bpp-Cl)14 was chosen to replace traditional N-protecting groups such as Fmoc, Boc and Cbz for the general use (Scheme 1).
The protection of phenylalanine benzyl ester was chosen for the initial experiment. The slurry of phenylalanine benzyl ester hydrochloride in dichloromethane (DCM) was added by N-methylmorpholine (NMM, 2.5 equiv.) and 4 Ǻ MS. By cooling the mixture to 0 oC, the Bpp-Cl (1) was added dropwise under inert gas to the resulting solution. The resulting mixture was warmed to room temperature and stirred overnight for complete consumption of the starting materials. The pure protected amino acid esters 2a was obtained in 95% yields by extraction and trituration with ether or dichloromethane/hexane (Scheme 2). It was found that either decreasing or increasing the temperature of Bpp-Cl addition resulted in a lower yield of 2a. The 4 Ǻ molecular sieves were also found to be important for the reaction.
With the optimized condition in hand, different amino acid esters were protected with phosphinyl chloride 1 (Scheme 3). Employing the hindrance amino acid esters slightly decreased the yields (2d, 2e, 2f, 2g and 2k in Scheme 3). Lysine and ornithine also afforded branched-protected products in good yields (2h and 2i in Scheme 3). All the N-phosphinyl amino acid esters were purified with standard manner as above.
These N-biphenylphosphinyl amino acid esters were readily converted into corresponding acid by either hydrolysis (methyl ester) or hydrogenation (benzyl ester), which overcome the sluggish cleavage reaction of N-phosphonyl amino acid esters.13 To study the peptide coupling efficiency, Bpp-Phe-OH (3a), Bpp-Ala-OH (3b) and more steric hindrance amino acid, Bpp-Val-OH (3c), were subjected to the standard coupling conditions for dipeptide synthesis. All the dipeptide was obtained via the GAP work-up in good yields (Scheme 4). Meanwhile, the known diphenylphosphinyl group 1’(Ph2PO-)17 was also tested in this synthesis; the coupling of Dpp-Phe-OH 3a’ with alanine benzyl ester hydrochloride resulted in racemization (Scheme 4). The bulkiness of the Bpp group may prevent the deprotonation of amino acid’s α-H, which provided the configuration maintenance.
Encouraged by the above results, we started to investigate the peptide chain extension. Symmetric linear biphalin18 was synthesized via the GAP protocol (Scheme 5).
Bpp-Tyr (tert-Bu)-OMe (2j) was hydrolyzed to Bpp-Tyr (tert-Bu)-OH (3d) in almost quantitative yield. Temperature was found to be important to maintain the L configure in tyrosine during the hydrolysis. The following peptide elongation and benzyl ester deprotection proceeded efficiently to afford the Bpp- Tyr (tert-Bu)-D-Ala-Gly-OH 4d in 90% yield. The coupling of tripeptide with H2N-Phe-NHNH-Phe-NH2 afforded the full protected biphalin 5a in 95% yields. Final deprotection with concentrate hydrochloride acid gave the biphalin in 89% yields. The Bpp-OH precipitated out during the reaction process, and can be recovered simply by filtration. All the reaction products were purified by simple extraction or filtration after washing. Similarly, two neuro linear peptides, full protected Leu-enkephalin and Met-enkephalin19 were synthesized via the above GAP protocol (Scheme 5). The iterative ester cleavage and peptide elongation gave Leu-enkephalin and Met-enkephalin in 84% and 76% yields, respectively.
We then study the GAP synthesis of subunit peptide KLLLL (5d) of sufaxin, which is a FDA approved drug in prevention of RDS in premature infants and meconium aspiration syndrome.2 The peptide contains four leucines and one lysine; and it is a hydrophobic peptide with the tendency of gelation during the synthesis. The branch–protected Lysine 2h was utilized as the first amino acid in GAP synthesis routine (Scheme 6). Through our GAP synthesis process, the iterative ester cleavage and peptide elongation afforded the final pentapeptide in 72% yield. The present GAP protocol using the phosphinyl group in the synthesis afforded high solubility of protecting peptide in the dichloromethane. The better solubility gave no racemization or difficulty occurring for purification. Because of the homogeneous property, the reaction was rapid and occurred to completion well as monitored by 31P NMR. These results demonstrated that both phosphinyl protection on α-amino and branched-amino groups can resulted in a GAP peptide synthesis.
In summary, we have demonstrated an efficient linear peptide synthesis via Group-Assisted Purification protocol by using biphenyl phosphinyl group 1(Bpp). Both α- and β- branch protected peptides have been synthesized in good chemical yields. The recyclization of diphenyl phosphinyl group during the final cleavage and high purity of resulting unprotected peptides have been achieved. Elongation difficulties, such as hindrance amino acid, gelation, hydrophobic peptide and sulfur containing peptide, can be overcome by this GAP protocol. Further applications to synthesize other bioactive peptides and peptide drugs will be under investigation in our laboratories.
EXPERIMENTAL
The 1H NMR, 13C NMR and 31P NMR spectra were recorded on a Jeol eclipse 400 SS (operating at 400 MHz for proton) at ambient temperature. The ESI-MS and ESI-HRMS were recorded with an Exactive (Thermo Scientific, San Jose, CA) mass spectrometer.
Starting Materials and Reagents:
Dry dichloromethane (DCM) was bought from Acros Organics and used directly. N, N’-Dimethylformamide (DMF) was dried over 4 Å molecule sieves powder. 4 Ǻ Molecular sieves powder was activated by heating in oven at 120 oC for 24 h. Triethylamine was stand over KOH solid overnight and distilled before use. Tetrahedron furan (THF) was distilled from Na metal before use. All the other chemicals were bought from commercial source and used directly after arrival.
General Procedure for Protection of amino acid ester with Bpp-Cl:
Bpp-OH (1 g) was suspended in DCM (10 mL) and was cooled to 0 oC. Oxalyl chloride was added to the solution via slow addition. After stirring at 0 oC for 1 h, the reaction solution was allowed to warm to room temperature and stirred for overnight. After the reaction completed, the reaction solution was evaporated and the residue was washed with hexane (3 times) and dried under vacuum to afford the Bpp-Cl as a white solid.
To a mixture of NMM (3 mmol), amino acid ester (2 mmol) and DCM (10 mL) was added Bpp-Cl (1 mmol) in DCM (5 mL) solution at 0 oC. The resulting mixture was stirred at room temperature for 24 h. The mixture was then filtered through celite, washed with by 5% citric acid aqueous solution, sat. aqueous NaHCO3 solution, H2O and then brine, dried over Na2SO4, filtered, and evaporated to give a white solid.
Compound 2a: a white solid, 484.1 mg, 95% yield. 1H NMR (400 MHz, DMSO-d6) δ 7.42 – 7.30 (m, 4H), 7.29 – 7.15 (m, 9H), 7.13 – 7.06 (m, 3H), 6.96 – 6.86 (m, 2H), 6.67 – 6.58 (m,2H), 5.19 (dd, J = 15.1, 10.5 Hz, 1H), 4.72 (q, J = 12.6 Hz, 2H), 3.40 – 3.30 (m, 2H), 3.29 – 3.21 (m, 1H), 2.39 – 1.89 (m, 6H). 13C NMR (100 MHz, DMSO-d6): δ 173.27, 138.22 (d, J = 5.1 Hz), 138.03 (d, J = 5.9 Hz), 136.84, 135.98, 129.38, 129.20, 129.15, 129.05, 128.70, 128.59 (d, J = 1.8 Hz), 128.36, 128.32, 128.28, 126.99, 126.61, 99.99, 65.97, 54.84, 48.88, 45.53, 29.85, 26.86, 26.75. 31P NMR (162 MHz, DMSO-d6) δ 54.98. ESI-HRMS calcd. for C32H32NO3PNa ([M+Na]+): 532.2018; found 532.2020.
Compound 2b: a white solid, 333 mg, 97% yield. 1H NMR (400 MHz, DMSO-d6) δ 7.33 – 7.13 (m, 10H), 5.12 – 4.89 (m, 1H), 3.43 (d, J = 2.4 Hz, 3H), 3.30 – 3.16 (m, 1H), 3.10 – 2.90 (m, 1H), 2.62 – 2.49 (m, 1H), 2.34 – 1.92 (m, 1H). 13C NMR (100 MHz, DMSO-d6): δ 171.79 (d, J = 6.0 Hz), 138.19 (d, J = 5.1 Hz), 137.65 (d, J = 5.8 Hz), 129.33 (d, J = 5.3 Hz), 128.82 (d, J = 2.2 Hz), 128.58 (d, J = 1.6 Hz), 128.28 (d, J = 4.7 Hz), 126.77 (d, J = 2.7 Hz), 126.66 (d, J = 2.2 Hz), 52.01, 48.44 (d, J = 77.2 Hz), 44.77 (d, J = 77.2 Hz), 40.87, 30.16 (d, J = 10.3 Hz), 27.21 (d, J = 11.2 Hz). 31P NMR (162 MHz, DMSO-d6) δ 55.65. ESI-HRMS calcd. for C19H22NO3PNa ([M+Na]+): 366.1235; found 366.1232.
Compound 2c: a white solid, 411 mg, 98% yield. 1H NMR (400 MHz, DMSO-d6) δ 7.37 – 7.13 (m, 15H), 5.10 – 4.98 (m, 1H), 4.94 (s, 2H), 3.30 – 3.19 (m, 2H), 3.12 – 2.97 (m, 1H), 2.60 (ddd, J = 17.9, 9.0, 5.1 Hz, 1H), 2.36 – 1.91 (m, 4H). 13C NMR (100 MHz, DMSO-d6): δ 171.22 (d, J = 6.0 Hz), 138.15 (d, J = 5.1 Hz), 137.64 (d, J = 5.8 Hz), 136.31, 129.30 (d, J = 5.3 Hz), 128.92, 128.83 (d, J = 2.3 Hz), 128.59, 128.57, 128.50, 128.28 (d, J = 4.7 Hz), 126.79 (d, J = 2.7 Hz), 126.65 (d, J = 2.3 Hz), 66.26, 48.43 (d, J = 77.2 Hz), 44.79 (d, J = 77.3 Hz), 41.09, 30.10 (d, J = 9.5 Hz), 27.24 (d, J = 10.9 Hz). 31P NMR (162 MHz, DMSO-d6) δ 55.66. ESI-HRMS calcd. for C25H26NO3PNa ([M+Na]+): 442.1548; found 442.1545.
Compound 2d: a white solid, 341.2mg, 96% yield. 1H NMR (400 MHz, DMSO-d6) δ 7.57 – 6.95 (m, 10H), 5.04 (dd, J = 14.5, 10.3 Hz, 1H), 3.33 – 2.97 (m, 6H), 2.35 – 1.79 (m, 4H), 0.91 (d, J = 6.9 Hz, 3H). 13C NMR (100 MHz, DMSO-d6): δ 174.01 (d, J = 6.7 Hz), 138.31 (d, J = 5.2 Hz), 137.54 (d, J = 5.8 Hz), 129.26 (d, J = 5.2 Hz), 128.67 (d, J = 2.1 Hz), 128.57 (d, J = 1.8 Hz), 128.16 (d, J = 4.6 Hz), 126.60 (d, J = 2.0 Hz), 126.53 (d, J = 2.4 Hz), 51.92, 48.31 (d, J = 76.5 Hz), 48.22, 44.86 (d, J = 76.6 Hz), 29.81 (d, J = 10.1 Hz), 27.45 (d, J = 10.9 Hz), 22.89. 31P NMR (162 MHz, DMSO-d6) δ 54.62. ESI-HRMS calcd. for C20H24NO3PNa ([M+Na]+): 380.1391; found 380.1388.
Compound 2e: a white solid, 411.8 mg, 95% yield. 1H NMR (400 MHz, DMSO-d6) δ 7.41 – 7.06 (m, 15H), 5.06 (dd, J = 15.9, 10.3 Hz, 1H), 4.94 (q, J = 12.6 Hz, 2H), 3.27 – 3.03 (m, 3H), 2.37 – 1.82 (m, 4H), 0.49 (d, J = 7.2 Hz, 3H). 13C NMR (100 MHz, DMSO-d6): δ 174.53, 138.13, 138.07 (d, J = 2.6 Hz), 136.50, 129.11 (d, J = 5.2 Hz), 128.89, 128.77 (d, J = 2.0 Hz), 128.54 (d, J = 1.7 Hz), 128.45, 128.34 (d, J = 4.6 Hz), 128.22, 126.79, 126.56, 66.16, 48.56 (d, J = 76.0 Hz), 48.48, 45.39 (d, J = 76.1 Hz), 29.48 (d, J = 9.4 Hz), 27.07 (d, J = 12.3 Hz), 19.91 (d, J = 5.9 Hz). 31P NMR (162 MHz, DMSO-d6) δ 55.29. ESI-HRMS calcd. for C26H28NO3PNa ([M+Na]+): 456.1704; found 456.1708.
Compound 2f: a white solid, 420 mg, 91% yield. 1H NMR (400 MHz, DMSO-d6) δ 7.36 – 7.08 (m, 15H), 4.95 (s, 2H), 4.60 (dd, J = 14.3, 10.8 Hz, 1H), 3.47 – 3.33 (m, 1H), 3.24 (dd, J = 13.5, 7.3 Hz, 1H), 3.11 (ddd, J = 10.8, 8.3, 5.0 Hz, 1H), 2.33 – 1.93 (m, 4H), 1.26 – 1.16 (m, 1H), 0.37 (d, J = 6.9 Hz, 3H), 0.29 (d, J = 6.8 Hz, 3H). 13C NMR (100 MHz, DMSO-d6): δ 173.56 (d, J = 1.6 Hz), 138.48 (d, J = 5.1 Hz), 137.94 (d, J = 5.9 Hz), 136.46, 129.18 (d, J = 5.2 Hz), 128.88, 128.70 (d, J = 2.3 Hz), 128.63, 128.54, 128.48 (d, J = 1.8 Hz), 126.64 (d, J = 2.7 Hz), 126.44 (d, J = 2.3 Hz), 100.00, 66.14, 58.18, 48.63 (d, J = 79.9 Hz), 45.87 (d, J = 73.5 Hz), 32.03 (d, J = 5.8 Hz), 29.72 (d, J = 9.7 Hz), 27.09 (d, J = 11.1 Hz), 18.63, 18.30. 31P NMR (162 MHz, DMSO-d6) δ 55.22. ESI-HRMS calcd. for C28H32NO3PNa ([M+Na]+): 484.2018; found 484.2020.
Compound 2g: a white solid, 351.5 mg, 88% yield. 1H NMR (400 MHz, DMSO-d6) δ 7.43 – 7.01 (m, 10H), 4.90 (dd, J = 16.2, 10.7 Hz, 1H), 3.33 – 3.13 (m, 6H), 2.35 – 1.81 (m, 4H), 1.40 (dt, J = 13.1, 6.5 Hz, 1H), 1.13 (t, J = 7.0 Hz, 2H), 0.64 (dd, J = 6.6, 1.8 Hz, 3H), 0.57 (dd, J = 6.5, 1.8 Hz, 3H). 13C NMR (100 MHz, DMSO-d6): δ 173.92 (d, J = 4.7 Hz), 138.34 (d, J = 5.4 Hz), 137.62 (d, J = 5.4 Hz), 129.25 (d, J = 5.1 Hz), 128.66 (d, J = 2.1 Hz), 128.48 (d, J = 1.8 Hz), 128.09 (d, J = 4.7 Hz), 126.56, 126.39, 51.70, 50.67, 48.22 (d, J = 78.2 Hz), 45.55 (d, J = 62.6 Hz), 45.16 (d, J = 12.1 Hz), 29.66 (d, J = 10.5 Hz), 27.43 (d, J = 10.8 Hz), 24.00, 23.08, 22.37. 31P NMR (162 MHz, DMSO-d6) δ 54.27. ESI-HRMS calcd. for C23H30NO3PNa ([M+Na]+): 422.1861; found 422.1859.
Compound 2h: a white solid, 504.7 mg, 92% yield. 1H NMR (400 MHz, DMSO-d6) δ 7.62 (d, J = 7.7 Hz, 1H), 7.44 – 6.98 (m, 15H), 4.99 (s, 2H), 4.39 (dd, J = 14.2, 6.7 Hz, 1H), 3.84 (td, J = 8.4, 5.4 Hz, 1H), 3.62 – 3.43 (m, 3H), 3.26 – 3.00 (m, 2H), 2.35 – 1.71 (m, 6H), 1.47 – 1.16 (m, 2H), 1.07 – 0.75 (m, 4H). 13C NMR (100 MHz, DMSO-d6): δ 173.45, 156.64, 138.43 (d, J = 3.3 Hz), 138.37 (d, J = 3.6 Hz), 137.46, 129.25 (d, J = 5.0 Hz), 128.89, 128.69 (d, J = 2.2 Hz), 128.55 (d, J = 1.7 Hz), 128.38, 128.33, 128.26, 126.56, 66.00, 54.31, 52.31, 48.67 (d, J = 76.6 Hz), 45.36 (d, J = 77.1 Hz), 39.19, 31.52 (d, J = 5.5 Hz), 30.81, 29.99 (d, J = 10.2 Hz), 27.87 (d, J = 10.4 Hz, 23.08. 31P NMR (162 MHz, DMSO-d6) δ 54.48. ESI-HRMS calcd. for C31H37N2O5PNa ([M+Na]+): 571.2338; found 571.2336.
Compound 2i: a white solid, 481.1 mg, 90% yield. 1H NMR (400 MHz, DMSO-d6) δ 7.60 (d, J = 7.7 Hz, 1H), 7.35 – 7.15 (m, 15H), 4.98 (s, 2H), 4.53 – 4.38 (m, 1H), 3.84 – 3.66 (m, 1H), 3.64 – 3.46 (m, 3H), 3.25 – 3.05 (m, 2H), 2.28 – 1.84 (m, 6H), 1.48 – 1.14 (m, 2H), 1.06 – 0.78 (m, 2H). 13C NMR (100 MHz, DMSO-d6): δ 173.36, 156.58, 138.34 (t, J = 5.2 Hz), 137.45, 129.22 (d, J = 5.0 Hz), 129.12 (d, J = 5.1 Hz), 128.89, 128.72 (d, J = 2.2 Hz), 128.60, 128.56 (d, J = 1.7 Hz), 128.55, 128.36 (d, J = 2.1 Hz), 128.29 (d, J = 2.9 Hz), 126.57 (d, J = 2.3 Hz), 66.00, 54.34, 52.31, 48.64 (d, J = 76.5 Hz), 45.33 (d, J = 77.2 Hz), 38.94, 29.90 (d, J = 9.9 Hz), 28.68 (d, J = 5.0 Hz), 28.38, 27.81 (d, J = 10.6 Hz). 31P NMR (162 MHz, DMSO-d6) δ 54.72. ESI-HRMS calcd. for C30H35N2O5PNa ([M+Na]+): 557.2181; found 557.2180.
Compound 2j: a white solid, 480.2 mg, 95% yield. 1H NMR (400 MHz, DMSO-d6) δ 7.41 – 7.07 (m, 10H), 6.89 – 6.56 (m, 4H), 4.79 (dd, J = 15.3, 9.9 Hz, 1H), 3.41 – 3.31 (m, 2H), 3.27 – 3.23 (m, 1H), 3.20 (s, 3H), 2.61 – 2.49 (m, 1H), 2.31 – 1.86 (m, 1H), 1.32 – 1.04 (m, 9H). 13C NMR (100 MHz, DMSO-d6): δ 174.10 (d, J = 1.9 Hz), 154.05, 138.28 (d, J = 4.9 Hz), 137.99 (d, J = 6.0 Hz), 131.57, 129.84, 129.18 (d, J = 5.2 Hz), 129.01 (d, J = 2.3 Hz), 128.58 (d, J = 1.8 Hz), 128.34 (d, J = 4.7 Hz), 126.89, 126.62, 123.94, 78.19, 54.85, 51.45, 48.46 (d, J = 79.4 Hz), 45.04 (d, J = 75.3 Hz), 40.71, 29.81 (d, J = 9.5 Hz), 29.04, 26.83 (d, J = 10.3 Hz). 31P NMR (162 MHz, DMSO-d6) δ 55.35. ESI-HRMS calcd. for C30H36NO4PNa ([M+Na]+): 528.2280; found 528.2283.
Compound 2k: a white solid, 618.3 mg, 89% yield. 1H NMR (400 MHz, DMSO-d6) δ 7.32 – 7.13 (m, 10H), 7.11 (br, 1H), 6.35 (br, 2H), 4.88 (dd, J = 15.2, 10.7 Hz, 1H), 3.42 (s, 3H), 3.39 – 3.30 (m, 1H), 3.27 – 3.18 (m, 1H), 3.08 (s, 1H), 2.93 (s, 2H), 2.69 – 2.57 (m, 2H), 2.45 (s, 3H), 2.40 (s, 3H), 2.33 – 1.96 (m, 7H), 1.36 (s, 6H), 0.83 – 0.73 (m, 4H). 13C NMR (100 MHz, DMSO-d6): δ 174.63 (d, J = 2.0 Hz), 157.99, 138.30, 131.94, 129.18, 129.12, 128.74, 128.54, 128.28, 126.73, 126.57, 124.86, 123.14, 116.82, 100.00, 99.54, 86.84, 52.50, 51.97, 45.19, 43.03, 39.04, 34.72, 34.46, 31.49, 28.82, 25.31, 22.59, 19.50, 18.13, 14.50, 12.84. 31P NMR (162 MHz, DMSO-d6) δ 55.36. ESI-HRMS calcd. for C36H47N4O6PSNa ([M+Na]+): 717.2852; found 717.2849.
General procedure for deprotection of benzyl esters:
To a flask, Bpp-attached Amino Acid benzyl ester or Bpp-attached peptide benzyl ester ( 1.0 mmol) was charged with Pd/C (10%, 0.2 g) in absolute EtOH solution. The reaction underwent under hydrogen for 6 h and the reaction mixture was filter through the Celite and the filtrate cake was washed by EtOH for 3 times. Collect all filtrates. Evaporate to get the corresponding acid.
General procedure for deprotection of methyl esters:
To a flask, Bpp-attached Amino Acid methyl ester or Bpp-attached peptide methyl ester ( 1.0 mmol) was suspended in a 10 mL MeOH/H2O (v:v, 9:1) solution. The mixture was cooled to 0 oC followed by addition of LiOH·H2O (5 mmol). The reaction was allowed to warm to room temperature and stirred overnight. HCl (1.0 M aqueous solution) was added to neutralize the reaction. The water phase was extracted by DCM for 3 times. Combine all organic phase. Dry over Na2SO4 and evaporate to get the corresponding acid.
Compound 3a: a white solid. 1H NMR (400 MHz, DMSO-d6) δ 12.87 (br, 1H), 7.51 – 6.97 (m, 15H), 6.72 – 6.66 (m, 2H), 4.74 – 4.49 (m, 1H), 2.35 – 1.84 (m, 7H). 13C NMR (100 MHz, DMSO-d6): δ 174.53 (d, J = 2.7 Hz), 138.72 (d, J = 5.2 Hz), 138.05 (d, J = 5.7 Hz), 137.94, 129.76, 129.25 (d, J = 5.1 Hz), 128.96 (d, J = 2.1 Hz), 128.96 (d, J = 2.1 Hz), 128.50 (d, J = 1.8 Hz), 128.33 (d, J = 4.5 Hz), 128.23, 126.79 (d, J = 2.7 Hz), 126.45 (d, J = 2.2 Hz), 126.41, 55.46, 54.91, 48.34 (d, J = 79.3 Hz), 45.41 (d, J = 74.9 Hz), 29.99 (d, J = 9.9 Hz), 26.93 (d, J = 10.6 Hz). 31P NMR (162 MHz, DMSO-d6) δ 54.29. ESI-HRMS calcd. for C25H26NO3PNa ([M+Na]+): 442.1548; found 442.1551.
Compound 3b: a white solid. 1H NMR (400 MHz, DMSO-d6) δ 12.37 (s, 1H), 7.43 – 7.08 (m, 10H), 4.86 (s, 1H), 3.23 (dd, J = 12.8, 7.1 Hz, 1H), 3.12 (d, J = 3.3 Hz, 1H), 3.02 (d, J = 6.4 Hz, 1H), 2.35 – 1.87 (m, 4H), 0.46 (d, J = 7.1 Hz, 3H). 13C NMR (100 MHz, DMSO-d6): δ 175.94, 138.24, 138.09, 129.16 (d, J = 5.1 Hz), 128.74, 128.53, 128.45, 126.77, 126.55, 55.46, 48.69, 48.61 (d, J = 76.0 Hz), 45.30 (d, J = 76.0 Hz), 29.55 (d, J = 10.2 Hz), 27.42 (d, J = 10.6 Hz), 20.17. 31P NMR (162 MHz, DMSO-d6) δ 55.47. ESI-HRMS calcd. for C19H22NO3PNa ([M+Na]+): 366.1235; found 366.1232.
Compound 3c: a white solid. 1H NMR (400 MHz, CDCl3) δ 7.35 – 7.17 (m, 10H), 3.58 (ddd, J = 22.8, 12.2, 7.4 Hz, 1H), 3.41 (td, J = 10.5, 4.3 Hz, 1H), 3.12 (td, J = 12.9, 7.5 Hz, 1H), 2.68 (t, J = 11.6 Hz, 1H), 2.57 – 2.02 (m, 4H), 1.59 (td, J = 13.0, 6.4 Hz, 1H), 0.51 (d, J = 6.8 Hz, 3H), 0.44 (d, J = 6.8 Hz, 3H). 13C NMR (100 MHz, CDCl3): δ 176.77, 136.64, 136.38, 128.96, 128.87, 128.59, 127.95, 127.09, 126.74, 58.50, 47.51 (d, J = 63.5 Hz), 46.73 (d, J = 60.1 Hz), 31.76, 30.45 (d, J = 10.4 Hz), 27.25 (d, J = 10.4 Hz), 18.51, 17.26. 31P NMR (162 MHz, CDCl3) δ 55.87. ESI-HRMS calcd. for C21H26NO3PNa ([M+Na]+): 394.1548; found 394.1552.
Compound 3a’: a white solid. 1H NMR (400 MHz, CDCl3) δ 13.20 (br, 1H), 8.00 – 7.64 (m, 2H), 7.52 – 7.09 (m, 13H), 3.99 – 3.56 (m, 2H), 3.18 (d, J = 13.5 Hz, 1H), 2.99 – 2.66 (m, 1H).13C NMR (100 MHz, CDCl3): δ 174.33, 137.24, 132.78 (d, J = 10.0 Hz), 132.47, 132.14, 131.88 (d, J = 9.8 Hz), 130.83, 130.11, 129.77, 128.76, 128.57 (d, J = 8.6 Hz), 128.41, 126.97, 55.83, 40.79 (d, J = 9.9 Hz). 31P NMR (162 MHz, CDCl3) δ 26.77.
General Procedure for Peptide Coupling Reactions:
Diisopropylethylamine (DIPEA) (2.4 mmol), Bpp-attached Amino Acid I (1 mmol) and Amino Acid II (1.2 mmol) were dissolved in 5 mL of dry DCM. The mixture was cooled to 0 oC. TBTU (1.2 mmol) was added in portion at 0 oC. The resulting mixture was stirred at 0 oC for 2 h and room temperature for 10 h. The mixture was then washed by 5% citric acid aqueous solution, sat. aqueous NaHCO3 solution, H2O and then brine. The organic phase was dried over Na2SO4, filtered and evaporated to give corresponding protected peptide as a white solid.
Compound 4a: a white solid. 1H NMR (400 MHz, DMSO-d6) δ 8.05 (d, J = 7.1 Hz, 1H), 7.39 – 7.10 (m, 17H), 7.02 – 6.98 (m, 3H), 6.63 (dd, J = 6.6, 2.9 Hz, 2H), 4.98 (s, 2H), 4.64 (dd, J = 15.4, 10.7 Hz, 1H), 4.11 (t, J = 7.2 Hz, 1H), 3.48 – 3.41 (m, 1H), 2.31 – 1.89 (m, 6H), 0.92 (d, J = 7.2 Hz, 3H). 13C NMR (100 MHz, DMSO-d6): δ 172.57, 172.16 (d, J = 2.0 Hz), 138.06 (d, J = 5.4 Hz), 137.88, 137.83, 136.46, 129.77, 129.21 (d, J = 5.0 Hz), 129.02 (d, J = 2.1 Hz), 128.90, 128.48, 128.46, 128.41, 128.18, 128.16, 126.88, 126.55, 126.34, 66.30, 53.85, 48.22 (d, J = 78.7 Hz), 47.99, 45.68 (d, J = 74.8 Hz), 29.58 (d, J = 10.4 Hz), 27.15 (d, J = 10.4 Hz), 17.33. 31P NMR (162 MHz, DMSO-d6) δ 56.44. ESI-HRMS calcd. for C35H37N2O4PNa ([M+Na]+): 603.2389; found 603.2392.
Compound 4b: a white solid. 1H NMR (400 MHz, DMSO-d6) δ 8.16 (d, J = 7.1 Hz, 1H), 7.37 – 7.06 (m, 20H), 6.97 (dd, J = 6.2, 3.2 Hz, 2H), 4.98 – 4.76 (m, 3H), 4.29 (q, J = 7.1 Hz, 1H), 3.11 (dd, J = 16.4, 9.0 Hz, 1H), 2.66 (d, J = 7.1 Hz, 2H), 2.31 – 1.93 (m, 4H), 0.33 (d, J = 6.9 Hz, 3H). 13C NMR (100 MHz, DMSO-d6): δ 174.02 (d, J = 3.1 Hz), 171.51, 138.00 (d, J = 5.8 Hz), 137.90 (d, J = 5.3 Hz), 137.00, 136.12, 129.94, 129.56, 129.21 (d, J = 5.2 Hz), 129.15, 128.96, 128.86, 128.84 (d, J = 0.8 Hz), 128.82, 128.77, 128.56 (d, J = 1.8 Hz), 128.50, 128.41 (d, J = 4.7 Hz), 128.33, 127.07, 126.84 (d, J = 2.9 Hz), 126.64, 66.34, 54.25, 48.75, 48.45 (d, J = 77.6 Hz), 45.41 (d, J = 76.1 Hz), 37.23, 29.49 (d, J = 10.3 Hz), 27.36 (d, J = 10.3 Hz), 19.52 (d, J = 4.9 Hz). 31P NMR (162 MHz, DMSO-d6) δ 56.59. ESI-HRMS calcd. for C35H37N2O4PNa ([M+Na]+): 603.2389; found 603.2391.
Compound 4c: a white solid. 1H NMR (400 MHz, MeOH-d4) δ 8.05 (d, J = 7.4 Hz, 1H), 7.40 – 7.10 (m, 20H), 7.10 – 7.01 (m, 2H), 5.04 – 4.93 (m, 2H), 4.63 – 4.55 (m, 1H), 3.62 (dd, J = 15.1, 10.5 Hz, 1H), 3.49 – 3.34 (m, 1H), 3.24 – 3.14 (m, 2H), 2.95 – 2.82 (m, 2H), 2.47 – 2.08 (m, 4H), 1.38 (tt, J = 9.0, 4.6 Hz, 1H), 0.44 (d, J = 6.8 Hz, 3H), 0.29 (d, J = 6.8 Hz, 3H). 13C NMR (100 MHz, MeOH-d4): δ 173.73 (d, J = 2.3 Hz), 171.32, 136.77 (d, J = 6.0 Hz), 136.45 (d, J = 5.9 Hz), 136.34, 135.54, 134.84, 133.88, 129.14, 128.93, 128.82, 128.71, 128.64 (d, J = 4.0 Hz), 128.59 (d, J = 2.5 Hz), 128.50, 128.37, 128.28, 128.20 (t, J = 3.0 Hz), 128.14 (d, J = 2.0 Hz), 128.02, 127.91 (d, J = 4.8 Hz), 127.58, 126.71 (d, J = 2.8 Hz), 126.62, 126.33 (d, J = 2.5 Hz), 67.83, 66.62, 58.22 (d, J = 2.1 Hz), 53.97 (d, J = 18.2 Hz), 46.26, 36.68 (d, J = 90.4 Hz), 31.98 (d, J = 4.5 Hz), 29.82 (d, J = 10.8 Hz), 26.97 (d, J = 11.4 Hz), 17.81, 16.40. 31P NMR (162 MHz, MeOH-d4) δ 57.34. ESI-HRMS calcd. for C37H41N2O4P Na ([M+Na]+): 631.2702; found 631.2799.
Compound 5a: a white solid. 1H NMR (400 MHz, DMSO-d6) δ 10.14 (s, 2H), 8.14 (s, 2H), 7.72 (t, J = 8.7 Hz, 4H), 7.37 – 7.14 (m, 30H), 6.67 (d, J = 8.3 Hz, 4H), 6.58 (d, J = 8.2 Hz, 4H), 5.32 – 5.13 (m, 2H), 4.63 – 4.44 (m, 2H), 3.95 – 3.84 (m, 2H), 3.23 (dd, J = 16.9, 6.4 Hz, 4H), 3.05 – 2.96 (m, 4H), 2.86 – 2.73 (m, 4H), 2.65 (s, 2H), 2.35 – 1.90 (m, 12H), 1.16 (s, 18H), 0.82 – 0.77 (m, 6H). 13C NMR (100 MHz, DMSO-d6): δ 172.77, 172.70, 170.63, 169.00, 153.80, 138.11, 137.94 (d, J = 5.4 Hz), 137.75 (d, J = 5.6 Hz), 132.15, 130.11, 129.79, 129.14, 129.09, 128.89, 128.78, 128.62, 128.54, 126.85, 126.61, 123.79, 78.07, 56.03, 52.78, 48.27, 48.24 (d, J = 82.0 Hz), 44.44 (d, J = 75.5 Hz), 42.37, 38.77, 38.47, 29.70 (d, J = 7.7 Hz), 29.01, 27.84, 17.67. 31P NMR (162 MHz, DMSO-d6) δ 56.36. ESI-HRMS calcd. for C86H103N10O12P2 ([M+H]+): 1529.7232; found 1529.7226.
Compound 5b: a white solid. 1H NMR (400 MHz, DMSO-d6) δ 8.25 – 8.16 (br, 2H), 7.82 (t, J = 5.7 Hz, 1H), 7.58 (d, J = 8.5 Hz, 1H), 7.34 – 7.13 (m, 15H), 6.68 (d, J = 8.4 Hz, 2H), 6.58 (d, J = 8.4 Hz, 2H), 5.22 (dd, J = 12.9, 10.0 Hz, 1H), 4.49 (td, J = 9.1, 4.0 Hz, 1H), 4.09 (dd, J = 13.9, 8.2 Hz, 1H), 3.48 (dd, J = 16.9, 6.3 Hz, 1H), 3.42 – 3.30 (m, 2H), 3.24 – 3.11 (m, 2H), 3.01 – 2.89 (m, 2H), 2.83 – 2.72 (m, 2H), 2.44 – 1.86 (m, 6H), 1.62 – 1.41 (m, 3H), 1.35 (d, J = 5.5 Hz, 9H), 1.18 (s, 9H), 0.84 (d, J = 6.5 Hz, 3H), 0.78 (d, J = 6.4 Hz, 3H). 13C NMR (101 MHz, DMSO-d6) δ 173.45, 171.97, 171.67, 169.69, 168.87, 153.84, 138.29, 138.01 (d, J = 4.9 Hz), 137.45 (d, J = 5.8 Hz), 132.03, 130.10, 129.76, 129.17, 129.12, 128.99, 128.97, 128.53, 126.95, 126.74, 126.65, 123.70, 80.98, 78.09, 56.25, 53.80, 51.72, 47.98 (d, J = 80.9 Hz), 44.39 (d, J = 75.4 Hz), 43.92, 43.52, 42.68, 42.31, 38.17, 29.86, 29.05, 28.13, 27.36, 24.74, 23.24 (d, J = 4.7 Hz), 21.97. 31P NMR (162 MHz, DMSO-d6) δ 56.48. ESI-HRMS calcd. for C52H69N5O8P ([M+H]+): 922.4884; found 922.4878.
Compound 5c: a white solid. 1H NMR (400 MHz, DMSO-d6) δ 8.24 – 8.17 (br, 2H), 7.84 (t, J = 5.7 Hz, 1H), 7.61 (d, J = 8.5 Hz, 1H), 7.35 – 7.16 (m, 15H), 6.71 – 6.66 (m, 2H), 6.59 (dd, J = 8.9, 2.2 Hz, 2H), 5.24 (dd, J = 13.1, 9.8 Hz, 1H), 4.46 (td, J = 9.1, 4.1 Hz, 1H), 4.28 – 4.14 (m, 1H), 3.48 (dd, J = 16.8, 6.4 Hz, 1H), 3.32 – 3.26 (m, 2H), 3.25 – 3.13 (m, 2H), 3.00 – 2.91 (m, 2H), 2.80 – 2.72 (m, 2H), 2.44 – 2.07 (m, 6H), 1.98 (s, 3H), 1.89 – 1.76 (m, 2H), 1.38 – 1.33 (m, 11H), 1.18 (d, J = 4.1 Hz, 9H). 13C NMR (100 MHz, DMSO-d6): δ 173.51, 171.75, 171.21, 169.76, 168.94, 153.85, 138.32, 137.99 (d, J = 5.0 Hz), 137.43 (d, J = 6.2 Hz), 132.03, 130.11, 129.73, 129.18, 129.13, 128.98, 128.57, 126.93, 126.77, 126.67, 123.70, 81.25, 78.09, 56.34, 53.94, 52.25, 48.02 (d, J = 80.1 Hz), 44.40 (d, J = 74.7 Hz), 42.67, 42.36, 38.06, 31.00, 30.04, 29.77 (d, J = 8.3 Hz), 29.05, 28.13, 28.00, 27.46 (d, J = 10.7 Hz), 15.03. 31P NMR (162 MHz, DMSO-d6) δ 56.59. ESI-HRMS calcd. for C51H67N5O8PS ([M+H]+): 940.4448; found 940.4442.
Compound 5d: a white solid. 1H NMR (400 MHz, DMSO-d6) δ 8.12 (d, J = 6.2 Hz, 1H), 7.93 – 7.68 (m, 3H), 7.32 – 7.13 (m, 16H), 4.97 (s, 2H), 4.52 – 4.04 (m, 6H), 3.80 (s, 1H), 3.55 (d, J = 2.3 Hz, 3H), 3.09 (d, J = 7.7 Hz, 1H), 2.32 – 1.82 (m, 7H), 1.40 (d, J = 25.1 Hz, 13H), 0.78 (dd, J = 11.9, 9.0 Hz, 28H). 13C NMR (100 MHz, DMSO-d6): δ 173.26, 172.43, 172.32, 172.21, 171.87, 138.42, 138.37, 137.56, 129.27, 129.22, 128.86, 128.69, 128.55, 128.36, 128.31, 128.14, 126.61, 65.84, 55.44, 55.19, 52.31, 51.35, 51.04, 50.59, 49.27 – 47.93 (m), 44.96, 41.38, 41.24, 41.09, 39.27, 39.22, 31.95, 31.89, 31.80, 30.04, 29.96, 28.00, 27.90, 24.62, 24.56, 24.48, 23.53, 23.31, 23.11, 22.23, 21.70. 31P NMR (162 MHz, DMSO-d6) δ 54.51. ESI-HRMS calcd. for C55H82N6O9P ([M+H]+): 1001.5881; found 1001.5875.
General Procedure for Final Cleavage of Bpp-Group and Recovery:
Bpp-protected peptide (0.130 mmol) was dissolved in dioxane (2 mL) and concentrated HCl (4 mL). The reaction mixture was stirred at room temperature until the reaction completed. After the completion, the Bpp-OH was filtered and the aqueous solution was evaporated below 30 °C to afford peptide as a solid with the recycled Bpp-OH.
Biphalin: a white solid. 1H NMR (400 MHz, DMSO-d6) δ 8.64 (dd, J = 19.5, 7.6 Hz, 2H), 8.38 – 8.22 (m, 8H), 7.26 – 7.16 (m, 10H), 6.96 (d, J = 8.4 Hz, 4H), 6.66 (d, J = 8.4 Hz, 4H), 4.56 – 4.46 (m, 1H), 4.40 – 4.30 (m, 1H), 4.19 (dt, J = 17.9, 7.2 Hz, 2H), 3.93 (d, J = 4.7 Hz, 2H), 2.90 (ddd, J = 20.5, 17.4, 4.5 Hz, 6H), 2.70 – 2.54 (m, 2H), 0.99 (t, J = 6.6 Hz, 6H). ESI-HRMS calcd. for C46H57N10O10 ([M+H]+): 909.4259; found 909.4253.
ACKNOWLEDGEMENT
We gratefully acknowledge the financial support from the NIH (R33DA031860), the Robert A. Welch Foundation (D-1361), the National Natural Science Foundation of China (21332005) and Jiangsu Educational Innovation Team Program (P.R. China) for their generous support of this research. We also thank NSF Grant CHE-1048553 and the CRIF program for supporting our NMR facility.
References
1. (a) A. M. Thayer, Chem. Eng. News, 2011, 89, 13; (b) K. Badiani, International Pharmaceutical Industry, 2012, 4, 84; (c) D. J. Craik, D. P. Fairliel, S. Liras, and D. Price, Chem. Biol. Drug Des., 2013, 81, 136. CrossRef
2. (a) J. Clardy and C. Walsh, Nature, 2004, 432, 829; CrossRef (b) H. H. Szeto and P. W. Schiller, Pharm. Res., 2011, 28, 2669; CrossRef (c) C. J. White and A. K. Yudin, Nat. Chem., 2011, 3, 509; CrossRef (d) N. Assem and A. K. Yudin, Nat. Protoc., 2012, 7, 1327; CrossRef (e) V. J. Hruby, G. Li, C. Haskell Luevano, and M. Shenderovich, Biopolymers, 1997, 43, 219. CrossRef
3. P. Vlieghe, V. Lisowski, J. Martinez, and M. Khrestchatisky, Drug Discov. Today, 2010, 15, 40. CrossRef
4. R. B. Merrifield, J. Am. Chem. Soc., 1963, 85, 2149. CrossRef
5. E. Bayer and M. Mutter, Nature, 1972, 237, 512. CrossRef
6. E. Bayer, M. Mutter, R. Uhmann, J. Polster, and H. Mauser, J. Am. Chem. Soc., 1974, 96, 7333. CrossRef
7. V. Gut and J. Rudinger, ‘Peptides,’ ed. by E. Bricas, North-Holland Publ., Co., Amsterdam, 1968, pp. 185–188.
8. M. Amblard, J. Fehrentz, J. Martinez, and G. Subra, Mol. Biotechnol., 2006, 33, 239. CrossRef
9. W. Chan and P. White, ‘Fmoc solid phase peptide synthesis: a practical approach,’ Oxford University Press, Oxford, 2000.
10. P. M. Fischer and I. Zheleva, J. Pept. Sci., 2002, 8, 529. CrossRef
11. S. Kitada, M. Takahashi, Y. Yamaguchi, Y. Okada, and K. Chiba, Org. Lett., 2012, 14, 5960. CrossRef
12. (a) A. Kattuboina and G. Li, Tetrahedron Lett., 2008, 49, 1573; CrossRef (b) P. Kaur, W. Wever, S. Pindi, R. Milles, P. Gu, M. Shi, and G. Li, Green Chem., 2011, 13, 1288; CrossRef (c) P. V. Kattamuri, T. Ai, S. Pindi, Y. Sun, P. Gu, M. Shi, and G. Li, J. Org. Chem., 2011, 76, 2792; CrossRef (d) P. Kaur, W. Wever, T. Rajale, and G. Li, Chem. Biol. Drug Des., 2010, 76, 314; CrossRef (e) P. Kaur, G. Shakya, H. Sun, Y. Pan, and G. Li, Org. Biomol. Chem., 2010, 8, 1091; CrossRef (f) P. Kaur, S. Pindi, W. Wever, T. Rajale, and G. Li, Chem. Commun., 2010, 46, 4330; CrossRef (g) P. Kaur, S. Pindi, W. Wever, T. Rajale, and G. Li, J. Org. Chem., 2010, 75, 5144. CrossRef
13. (a) H. Sun, T. Rajale, Y. Pan, and G. Li, Tetrahedron Lett., 2010, 51, 4403; CrossRef (b) S. Pindi, J. B. Wu, and G. Li, J. Org. Chem., 2013, 78, 4006; CrossRef (c) H. Sun, J. L. Han, P. V. Kattamuri, Y. Pan, and G. Li, J. Org. Chem., 2013, 78, 11711.
14. S. Pindi, P. Kaur, G. Shakya, and G. Li, Chem. Biol. Drug Des., 2011, 77, 20. CrossRef
15. J. B. Wu, G. H. An, S. Q. Lin, J. B. Xie, W. Zhou, H. Sun, Y. Pan, and G. Li, Chem. Commun., 2014, 50, 1259. CrossRef
16. (a) V. Declerck, P. Nun, J. Martinez, and F. Lamaty, Angew. Chem. Int. Ed., 2009, 48, 9318; CrossRef (b) V. R. Pattabiraman and J. W. Bode, Nature, 2011, 480, 471; CrossRef (c) E. Ko, J. Liu, L. M. Perez, G. Lu, A. Schaefer, and K. Burgess, J. Am. Chem. Soc., 2011, 133, 462; CrossRef (d) W.-K. Chan, C.-M. Ho, M.-K. Wong, and C.-M. Che, J. Am. Chem. Soc., 2006, 128, 14796; CrossRef (e) R. Hirschmann, A. B. Smith, III, C. M. Taylor, P. A. Benkovic, S. D.Taylor, K. M. Yager, P. A. Sprengeler, and S. J. Benkovic, Science, 1994, 265, 234. CrossRef
17. G. W. Kenner, G. A. Moore, and R. Ramage, Tetrahedron Lett., 1976, 17, 3623. CrossRef
18. (a) P. W. Schiller, Biopolymers, 2005, 80, 492; (b) B. Sinha, Z. Cao, T. F. Murray, and J. V. Aldrich, J. Med. Chem., 2009, 52, 7372; CrossRef (c) A. W. Lipkowski, A. M. Konecka, and I. Sroczynska, Peptides, 1982, 3, 697; CrossRef (d) Y. Shimohigashi, T. Costa, H. C. Chen, and D. Rodbard, Nature, 1982, 297, 333; CrossRef (e) M. Kawalec, J. E. Kowalczyk, M. Beresewicz, A. W. Lipkowski, and B. Zablocka, Neurochem. Res., 2011, 36, 2091; CrossRef (f) G. Li, W. Haq, L. Xiang, B. S. Lou, R. Houghes, I. A. De Leon, P. Davis, T. J. Gillespie, M. Romanowski, X. Y. Zhu, A. Misicka, A. W. Lipkowski, F. Porreca, T. P. Davis, H. I. Yamamura, D. F. O’Brien, and V. J. Hruby, Bioorg. Med. Chem. Lett., 1998, 8, 555; CrossRef (g) L. Yang, H. Wang, K. Shah, V. T. Karamyan, and T. J. Abbruscato, Brain Res., 2011, 1383, 307. CrossRef
19. (a) J. Hughes, T. W. Smith, H. W. Kosterlitz, L. A. Fothergill, B. A. Morgan, and H. R. Morris, Nature, 1975, 258, 577; CrossRef (b) M. Comb, P. H. Seeburg, J. Adelman, L. Eiden, and E. Herbert, Nature, 1982, 295, 663. CrossRef