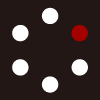
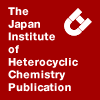
HETEROCYCLES
An International Journal for Reviews and Communications in Heterocyclic ChemistryWeb Edition ISSN: 1881-0942
Published online by The Japan Institute of Heterocyclic Chemistry
e-Journal
Full Text HTML
Received, 15th May, 2014, Accepted, 23rd June, 2014, Published online, 3rd July, 2014.
DOI: 10.3987/COM-14-13029
■ Synthesis and Characterization of N-Substituted (S)-1,2,3,4-Tetrahydroisoquinoline-3-carboxamides and Thioamides as Organocatalysts for Asymmetric Aldol Reaction
Ladislav Androvič, Pavel Drabina,* Illia Panov, Lydie Harmand, Zdeňka Padělková, and Miloš Sedlák
Institute of Organic Chemistry and Technology, University of Pardubice, Studentská 573, Pardubice, 532 10, Czech Republic
Abstract
In this paper, the preparation and characterization of eight optically pure N-functionalized (S)-1,2,3,4-tetrahydroisoquinoline-3-carboxamides and thioamides is described. The prepared amides and thioamides were tested as organocatalysts of aldol reaction of 4-nitrobenzaldehyde and acetone. The highest ee of formation of 4-hydroxy-4-(4-nitrophenyl)butan-2-one was obtained with (S)-N-[(1R)-1-phenylethyl]-1,2,3,4-tetrahydroisoquinoline-3-thiocarboxamide (77% ee). The observed deceleration of the aldol reaction catalyzed in this way, as compared with that catalyzed with (S)-proline, was attributed to the formation of little reactive cyclic intermediate, which was isolated and characterized.INTRODUCTION
(S)-1,2,3,4-Tetrahydroisoquinoline-3-carboxylic acid belongs among the chiral synthetic α-amino acids that are not found in nature. The acid can be synthesized by Pictet–Spengler reaction of phenylalanine with formaldehyde involving bridge formation between amino group and aromatic nucleus of phenylalanine by means of methylene group.1 The compounds whose molecules contain an incorporated 1,2,3,4-tetrahydroisoquinoline skeleton exhibit a number of effects on biological systems; e.g., they possess high affinity to opioid receptors,2,3 or act as inhibitors of some enzymatic reactions.4–6 In asymmetric synthesis, the derivatives of (S)-1,2,3,4-tetrahydroisoquinoline-3-carboxylic acid were used as ligands of ruthenium complexes in hydrogenations of ketones (up to 94% ee).7 The complexes of copper(II) acetate with the oxazolines derived from (S)-1,2,3,4-tetrahydroisoquinoline-3-carboxylic acid were also successfully used as highly enantioselective catalysts for the Henry reaction (up to 77% ee).8 Moreover, other derivatives of (S)-1,2,3,4-tetrahydroisoquinoline-3-carboxylic acid were proved as useful enantioselective catalysts in the addition reaction of diethylzinc to aldehydes (more than 99% ee).9 Furthermore, functionalized esters of this acid catalyze the asymmetric Diels–Alder reaction of cinnamic aldehyde with cyclopentadiene (43% ee for the exo-product, 50% ee for the endo-product, in the ratio of 2:1 for the exo:endo-product).10 It is known, that in analogy to the molecule of (S)-proline, also the molecule of (S)-1,2,3,4-tetrahydroisoquinoline-3-carboxylic acid possesses a rigid structure, and incorporation of this α-amino acid into a peptide chain allows preparation of peptides with well-defined tertiary structures.11 With regard to the similarity with the molecule of (S)-proline, it is possible to anticipate analogous properties also for corresponding derivatives of (S)-1,2,3,4-tetrahydroisoquinoline-3-carboxylic acid. This presumption reveals their possible application in organocatalysis. Therefore, the aim of the research work described in this paper was to prepare and characterize a series of new optically pure N-functionalized amides and thioamides of (S)-1,2,3,4-tetrahydroisoquinoline-3-carboxylic and to test them as organocatalysts of aldol reactions.11–13
RESULTS AND DISCUSSION
N-Functionalized (S)-1,2,3,4-tetrahydroisoquinoline-3-carboxamides (1a-g) were prepared by reactions of amines and sulfonamide (aniline, 2-aminopyridine, benzylamine, toluene-4-sulfonamide (R)-1-phenylethylamine and (S)-1-phenylethylamine) with activated (S)-2-(tert-butoxycarbonyl)- 1,2,3,4-tetrahydroisoquinoline-3-carboxylic acid. The activation of carboxylic group was achieved by the action of ethyl chloroformate (Method A) or dicyclohexylcarbodiimide (DCC) (Method B). The yields of functionalized (S)-1,2,3,4-tetrahydroisoquinoline-3-carboxamides (1a-g) varied in the range 59–65% (Scheme 1).
In the next reaction step, the amides 1f and 1g were transformed by the reaction with Lawesson reagent into the corresponding thioamides 1h (52%) and 1i (50%). In the last step, the protecting tert-butoxycarbonyl group was removed from the prepared amides 1a-g and thioamides 1h,i by the reaction with trifluoroacetic acid (TFA). In this way, we prepared (S)-1,2,3,4-tetrahydroisoquinoline-3-carboxamide (2a)14 and its N-functionalized amides 2b-g and thioamides 2h,i in the yields of 68–77%. The structure of derivative 2h was confirmed by means of X-ray diffraction (Figure 1).
Consequently, the catalytic activities of the prepared amides 2a-g and thioamides 2h,i were tested and compared with the earlier-described analogous derivatives of (S)-proline.11–13 Enantioselectivities of prepared organocatalysts were studied in the reactions of 4-nitrobenzaldehyde with cyclohexanone and acetone. The aldol reaction was performed in the presence of 20 mol% of organocatalyst at two temperatures (25 °C, –10 °C), the media used being acetone and water/acetone mixtures (Table 1). In order to attain a measurable conversion, it was necessary to evaluate the reactions as late as after 7 days. It turned out, that the reaction of 4-nitrobenzaldehyde with cyclohexanone, in contrast to that with acetone, does not take place even with the application of an equivalent amount of the tested organocatalysts 2a-i.
Table 1 presents the values of conversion and optical yields of aldol reaction obtained under different reaction conditions. The aldol formed was isolated from the reaction mixture by means of column chromatography (SiO2, EtOAc/hexane (1/1)). The evaluation showed that a more suitable reaction medium is the acetone/water mixture 80/20 by volume. It was also found that in the presence of trifluoroacetic acid the aldol reaction catalyzed by derivatives 2a-i did not proceed, although in the case of analogous derivatives of (S)-proline, an addition of catalytic amount of trifluoroacetic acid resulted in an increase from 86% ee to 99% ee.13 The unsubstituted amide 2a catalyzed the aldol reaction with the conversion of 98%, but the ee value attained was only 13% (entry 1). The best enantiomeric excess was achieved with the application of thioamide 2h (77% ee); however, the conversion was only 17% (25 °C) (entry 7). Lowering of the reaction temperature from 25 °C to –10 °C with catalyst 2b led to stopping of the reaction (entries 2 and 10). With catalysts 2c,d,f, the temperature decrease caused an only negligible increase in enantiomeric excess, namely from 16% to 19%, from 17% to 29%, and from 36% to 44% (entries 3 and 11, entries 4 and 12, entries 6 and 13, respectively). A significant lowering of conversion was only observed with catalyst 2d and 2g, namely from 92% to 56% (entries 4 and 12) resp. from 86% to 56% (entries 7 and 14). A higher content of water in the reaction medium (80% by vol.) in the case of catalyst 2f resulted in considerable deceleration of reaction, and hence also of conversion (25%) and in lowering of enantioselectivity, as compared with the reaction performed in the mixture acetone/water (20%) (entry 23).
The next group of our organocatalysts (2h,i) comprises an isolobal exchange of the amide oxygen atom for sulfur. This structural modification caused a distinct increase in enantioselectivity of the aldol reaction, i.e. 77% ee in the case of thioamide 2h as compared with 36% ee of amide 2f. The increase in enantioselectivity can be ascribed to the higher acidity of thioamide functional group as compared with that of amide group (e.g., pKa MeCSNH2 is 18.5, but that of MeCONH2 is 25.5).15 Higher acidity contributes to the formation of the stronger hydrogen bond with the oxygen atom of 4-nitrobenzaldehyde in the transition state of aldol reaction, which leads to increase in stereoselectivity of aldol reaction.11 For the same reason, a further increase in acidity at the amide nitrogen atom and consequently enantioselectivity (39% ee) was observed in the case of amide 2e bearing tosyl group. Although the acidity of the derivative 2e is the highest in this serie, its enantioselectivity is lowered probably due to steric demands of the toluenesulfonic group.
Introduction of another chiral centre into the organocatalyst molecule also increased the enantioselectivity, which was observed in the case of amide 2f (36% ee) in comparison with amides 2a-e (max. 17% ee). On the other hand, the presence of the center with opposite configuration (S) did not affect enantioselectivity significantly (2g, 14% ee). However, a much more significant increase was observed in the analogous case of thioamide 2h (77% ee) compared with thioamide 2i (40% ee).
On the basis of the presented results, (S)-1,2,3,4-tetrahydroisoquinoline-3-N-[(1R)- 1-phenylethyl]thiocarboxamide (2h) represents the best organocatalyst in the given series from the point of enantioselectivity (25 °C, 17%, 77% ee). In the catalysis using the analogous thioamide derived from (S)-proline, ((S)-N-[(1R)-1-phenylethyl]pyrrolidine-2-thiocarboxamide), the yield of isolated product was 62% and the enantiomeric excess was 84% at the temperature of 4 °C.13 These results are comparable from the standpoint of enantioselective effectiveness of the two analogous thioamide catalysts. The chemical yield obtained with 2h is significantly lower, as can be explained by general reactivity of corresponding enamines - reactivity of them follows the order pyrrolidine-derived > acyclic-derived > piperidine-derived enamines.16 On the other hand, in comparison with catalysts 2, the pipecolinic acid afforded very low catalytic activity (˂ 10%) and no enantioselectivity for this aldol reaction.17 Therefore, we consider that the presence of the benzene ring in the structure of the catalysts 2 positive influence their catalytic activity.
Hence, the aldol reaction catalyzed by thioamide 2h was further studied in more detail (TLC). The reaction of 2h with 4-nitrobenzaldehyde was used to prepare the cyclic product 3a, and the reaction with acetone was used to prepare the analogous cyclic product 3b (Scheme 2). The monitoring of the aldol reaction course showed that, under the conditions of aldol reaction, the reaction mixture contained both cyclic products (3a and 3b). Therefore, the cyclic product 3a alone was submitted to the reaction with equivalent amount of acetone. In this experiment, the presence of 3b was observed during the reaction; however, it was subsequently transformed into the product of aldol reaction, i.e. 4-hydroxy-4-(4-nitrophenyl)butan-2-one. From this finding it follows that 3a has to be decomposed back to the starting 2h and 4-nitrobenzaldehyde. In analogous way, the cyclic product 3b alone was submitted to the reaction with equivalent amount of 4-nitrobenzaldehyde.
It was found that in this reaction, 3b is only transformed to the product of aldol reaction – most probably via enamine.18 From these findings, summarized in Scheme 2, it is obvious that under the conditions of aldol reaction the operating mechanism is the reversible formation of little reactive intermediate 3a. This reversible formation of 3a makes itself felt kinetically as a reaction “blind alley”, which leads to general retardation of the aldol reaction course. A similar occurrence of such mentioned blind reaction pathways was described in the case of S-proline derivatives and called “parasitic dead dens”.18
EXPERIMENTAL
All starting substances were purchased from Sigma-Aldrich or Acros. 1H NMR and 13C NMR spectra were recorded on a Bruker Avance 400 instrument at the temperatures of 25 °C or 75 °C. Chemical shifts δ are referenced to standard Me4Si (CDCl3 and DMSO-d6, δ = 0.00 ppm). The solvent residual peak was used as inner standard for 13C NMR spectra (77.00 ppm for CDCl3, 39.60 ppm for DMSO-d6). The elemental analysis was carried out using an apparatus FISONS Instruments EA 1108 CHN. The optical rotatory power was measured on a Perkin-Elmer 341 instrument at λ = 589 nm at the temperature of 20 °C, the concentration c was given in g/100 mL.
4-Toluenesulfonic acid benzyl (S)-1,2,3,4-tetrahydroisoquinoline-3-carboxylate salt. To a solution of L-phenylalanine (20 g; 0.12 mol) in conc. HCl (155 mL; 35%; 1.77 mol) was slowly added a solution of formaldehyde in water (37%; 0.60 mol; 45 mL). The mixture was heated to the temperature of 100 °C for 4 h. The solid phase was isolated by filtration after standing for 24 h at the temperature of 25 °C and then washed with water (100 mL) and cold acetone (50 mL) and dried. The crude hydrochloride of (S)-1,2,3,4-tetrahydroisoquinoline-3-carboxylic acid (ca 20 g) was then heated with benzyl alcohol (11 g; 0.1 mol) and 4-toluenesulfonic acid (17.2 g; 0.1 mol) in benzene (180 mL) for 5 h. The pure product (22.4 g; mp 148–150 °C) was obtained after 4 fold recrystallization from aqueous MeOH (80%); [α]D20 –61.0 (c 1.0, MeOH). 1H NMR (DMSO-d6, ppm): δ 2.27 (s, 3H, CH3), 3.16 (dd, 1H, H(4a), 2J = 17.1 Hz, 3J = 11.6 Hz), 3.32 (dd, 1H, H(4b), 2J = 17.1 Hz, 3J = 5.0 Hz), 4.34–4.44 (m, 2H, H(1a) + H(1b)), 4.67 (dd, 1H, H(3), 3J = 11.6 Hz, 3J = 5.0 Hz), 5.30 (s, 2H, CH2), 7.09–7.11 (m, 2H, Ar), 7.25–7.27 (m, 4H, Ar), 7.35–7.48 (m, 7H, Ar), 9.78 (s, 2H, NH2+). 13C NMR (DMSO-d6, ppm): δ 20.9, 28.01, 44.2, 53.4, 67.5, 125.6, 126.7, 127.01, 127.8, 128.3, 128.2, 128.6, 128.7, 128.9, 130.4, 135.2, 138.0, 145.4, 168.5. Anal. Calcd for C25H25NO5S (439.5): C, 65.58; H, 5.73; N, 3.19; S, 7.30. Found: C, 65.29; H, 5.59; N, 3.12; S, 6.99.
Benzyl 2-tert-butyl (S)-1,2,3,4-tetrahydroisoquinoline-2,3(1H)-dicarboxylate. 4-Toluenesulfonic acid benzyl (S)-1,2,3,4-tetrahydroisoquinoline-3-carboxylate salt (18 g; 0.04 mol) was neutralized with aqueous solution of NaHCO3 (8%; 0.14 mol) and extracted with EtOAc (3 × 50 mL). The organic extract was washed with saturated solution of NH4Cl (1 × 50 mL), with water (3 × 50 mL), dried (Na2SO4) and evaporated. The obtained free base of benzyl ester was acylated with di-tert-butyl dicarbonate (10.5 g; 0.048 mol) in CH2Cl2 (80 mL) at the temperature of 25 °C for 2 h. The reaction mixture was then washed with aqueous solution of citric acid (10%; 50 mL), water (2 × 50 mL), dried (Na2SO4) and evaporated. Yield 13.9 g (95%) as oil. [α]D20 –2.2 (c 1.1, MeOH). 1H NMR (DMSO-d6, ppm, 75 °C): δ 1.44–1.56 (s, 9H, C(CH3)3), 3.16–3.20 (m, 1H, H(4a)), 3.29–3.34 (m, 1H, H(4b)), 4.54–4.58 (m, 1H, H(1a)), 4.69–4.74 (m, 1H, H(1b)), 4.86–5.25 (m, 3H, CH2 a H(3)), 7.10–7.25 (m, 6H, Ar), 7.29–7.32 (m, 3H, Ar). 13C NMR (DMSO-d6, ppm, 75 °C): δ 27.5, 30.4, 43.5, 54.6, 65.5, 79.3, 125.5, 126.1, 126.2, 126.9, 127.3, 127.7, 131.5, 135.5, 170.6. Anal. Calcd for C22H25NO4 (367.4): C, 71.91; H, 6.86; N, 3.81. Found: C, 71.85; H, 6.79; N, 3.90.
(S)-2-(tert-Butoxycarbonyl)-1,2,3,4-tetrahydroisoquinoline-3-carboxylic acid. The mixture of benzyl 2-tert-butyl (S)-1,2,3,4-tetrahydroisoquinoline-2,3(1H)-dicarboxylate (10.6 g; 28.9 mmol), Pd/C (0.5 g; 5%) in EtOAc (150 mL) was stirred under hydrogen atmosphere (ca 5 kPa) at the temperature of 25 °C for 24 h. The catalyst was removed by filtration and the solvent was evaporated. White crystals, mp 122–125 °C (EtOAc/hexane; 1/1); yield: 8.3 g (71%); [α]D20 +16.6 (c 1.0, MeOH). 1H NMR (CDCl3, ppm): (two rotamers) δ 1.39–1.50 (m, 9 H, C(CH)3), 3.08–3.25 (m, 2H, H(4a) + H(4b)), 4.42–4.51 (m, 1H), 4.65–4.74 (m, 1.5H), 5.10–5.13 (m, 0.5H), 7.07–7.25 (m, 4H, Ar), 10.42 (brs, 1H, CO2H). 13C NMR (CDCl3, ppm): δ 28.2, 28.3, 30.8, 31.3, 43.8, 44.4, 52.3, 54.1, 81.0, 126.2, 126.3, 126.7, 126.9, 127.7, 128.5, 131.6, 131.8, 132.6, 133.7, 154.7, 155.6, 177.0, 177.7. Anal. Calcd for C15H19NO4 (277.3): C, 64.97; H, 6.91; N, 5.05. Found: C, 64.89; H, 6.91; N, 4.97.
General methods for preparation of Boc-protected substituted (S)-1,2,3,4-tetrahydroisoquinoline- 3-carboxamides (1a-g).
Method A. To a solution of (S)-2-(tert-butoxycarbonyl)-1,2,3,4-tetrahydroisoquinoline-3-carboxylic acid (3.1 mmol), Et3N (1 mL) in THF (25 mL) was added a solution of ethyl chloroformate (3.1 mmol) in THF (2 mL) during 10 min. After that, the appropriate amine (4 mmol) was added and the mixture was stirred at the temperature of 25 °C for 16 h. Then Et3N·HCl was filtered off and the filtrate was evaporated. The residue was dissolved in CHCl3 (30 mL) and washed with water (1 × 10 mL), aqueous solution of NaOH (5%) (1 × 10 mL), brine (1 × 10 mL), water (1 × 10 mL) and dried (Na2SO4). The organic phase was evaporated and the residue was recrystallized.
Method B. To a solution of (S)-2-(tert-butoxycarbonyl)-1,2,3,4-tetrahydroisoquinoline-3-carboxylic acid (2.8 mmol), dicyclohexylcarbodiimide (3.1 mmol) and 4-dimethylaminopyridine (5 mg) in CH2Cl2 (30 mL) was added a solution of appropriate amine (2.8 mmol) in CH2Cl2 (10 mL) at the temperature of 25 °C. The N,N’-dicyclohexylurea formed was filtered off after 5 h. The filtrate was concentrated and purified through chromatography column (SiO2, EtOAc). The crude product was recrystallized.
(S)-2-(tert-Butoxycarbonyl)-1,2,3,4-tetrahydroisoquinoline-3-carboxamide (1a): Method A; white crystals; mp 130–131 °C (EtOAc/hexane; 1/1); yield: 780 mg (78%); [α]D20 –28.5 (c 1.0, MeOH). 1H NMR (DMSO-d6, ppm, 75 °C): δ 1.42 (s, 9H, C(CH3)3), 3.05–3.08 (m, 2H, H(4a) + H(4b)), 4.40–4.61 (m, 3H, H(1a) + H(1b) + H(3)), 6.84 (brs, 2H, CONH2), 7.15 (s, 4H, Ar). 13C NMR (DMSO-d6, ppm, 25 °C): (two rotamers) δ 28.0, 28.1, 31.5, 31.8, 43.9, 44.6, 52.9, 54.7, 79.2, 79.3, 125.9, 126.3, 126.7, 127.5, 128.1, 132.7, 133.5, 133.6, 134.6, 154.3, 154.7, 172.8, 173.3. Anal. Calcd for C15H20N2O3 (276.3): C, 65.20; H, 7.30; N, 10.13. Found: C, 65.09; H, 7.31; N, 10.11.
(S)-N-Phenyl-2-(tert-butoxycarbonyl)-1,2,3,4-tetrahydroisoquinoline-3-carboxamide (1b): Method A; white crystals; mp 150–152 °C (EtOAc/hexane; 1/1); yield: 780 mg (61%); [α]D20 –68.5 (c 1.0, MeOH). 1H NMR (DMSO-d6, ppm, 75 °C): δ 1.38 (s, 9H, C(CH3)3), 3.05–3.10 (m, 1H, H(4a)), 3.21(dd, 1H, H(4b), 2J = 15.5 Hz, 3J = 6.2 Hz), 4.47–4.51 (m, 1H, H(1a)), 4.64–4.68 (m, 2H, H(1b) + H(3)), 6.99–7.03 (m, 1H, Ar), 7.15–7.21 (m, 4H, Ar), 7.23–7.27 (m, 2H, Ar), 7.50–7.52 (m, 2H, Ar), 9.69 (s, 1H, CONH). 13C NMR (DMSO-d6, ppm, 25 °C): (two rotamers) δ 27.8, 28.0, 31.8, 44.0, 44.9, 53.9, 56.0, 79.2, 79.4, 119.1, 123.1, 125.7, 126.4, 126.5, 127.0, 127.1, 127.7, 128.6, 132.3, 133.8, 134.0, 135.3, 138.9, 154.0, 154.5, 170.1, 170.6. Anal. Calcd for C21H24N2O3 (352.4): C, 71.57; H, 6.86; N, 7.95. Found: C, 71.78; H, 6.82; N, 8.05.
(S)-N-(Pyridine-2-yl)-2-(tert-butoxycarbonyl)-1,2,3,4-tetrahydroisoquinoline-3-carboxamide (1c): Method B; white crystals; mp 130–132 °C (cyclohexane); yield: 830 mg (65%); [α]D20 –65.1 (c 1.0, MeOH). 1H NMR (DMSO-d6, ppm, 75 °C): δ 1.38 (s, 9H, C(CH3)3), 3.07–3.12 (m, 1H, H(4a)), 3.19–3.25 (m, 1H, H(4b)), 4.50–4.53 (m, 1H, H(1a)), 4.64–4.68 (m, 1H, H(1b)), 4.80 (brs, 1H, H(3)), 7.03–7.06 (m, 1H, Py), 7.19 (s, 4H, Ar), 7.68–7.72 (m, 1H, Py), 7.93–7.95 (m, 1H, Py), 8.28–8.29 (m, 1H, Py), 10.17 (s, 1H, CONH). 13C NMR (DMSO-d6, ppm, 25 °C): (two rotamers) δ 27.8, 28.0, 31.8, 44.0, 44.9, 53.8, 55.6, 79.2, 79.5, 113.2, 113.3, 119.3, 125.6, 125.8, 126.4, 126.6, 127.0, 127.1, 127.7, 132.3, 133.6, 134.0, 135.2, 138.0, 138.1, 147.9, 151.8, 151.9, 154.0, 154.6, 171.0, 171.5. Anal. Calcd for C20H23N3O3 (353.4): C, 67.97; H, 6.56; N, 11.89. Found: C, 68.26; H, 6.64; N, 11.81.
(S)-N-Benzyl-2-(tert-butoxycarbonyl)-1,2,3,4-tetrahydroisoquinoline-3-carboxamide (1d): Method B; white crystals; mp 98–101 °C (EtOAc/hexane; 1/1); yield: 850 mg (65%); [α]D20 –17.5 (c 1.0, MeOH). 1H NMR (DMSO-d6, ppm, 75 °C): δ 1.40 (s, 9H, C(CH3)3), 3.09 (m, 2H, H(1a) + H(1b)), 4.20 (d, 2H, CH2Ph, 3J = 5.95 Hz), 4.46–4.63 (m, 3H, H(4a) + H(4b) + H(3)), 7.01 (m, 2H, Ar), 7.14–7.21 (m, 7H, Ar), 8.05 (brs, 1H, NH). 13C NMR (DMSO-d6, ppm, 25 °C): (two rotamers) δ 22.0, 22.3, 28.0, 28.2, 32.3, 44.4, 47.6, 55.0, 55.1, 79.2, 125.8, 125.9, 126.2, 126.5, 128.1, 133.6, 134.9, 144.3, 154.4, 170.6. Anal. Calcd for C22H26N2O3 (366.5): C, 72.11; H, 7.15; N, 7.64. Found: C, 72.12; H, 6.86; N, 7.89.
(S)-N-[(4-Methylphenyl)sulfonyl]-2-(tert-butoxycarbonyl)-1,2,3,4-tetrahydroisoquinoline-3-carbox-amide (1e): Method B; white crystals; mp 98–101 °C (column chromatography; SiO2; EtOAc); yield: 900 mg (75%); [α]D20 +14.9 (c 1.1, MeOH). 1H NMR (DMSO-d6, ppm, 25 °C): (two rotamers) δ 1.12–1.42 (m, 9H, C(CH3)3), 2.36–2.38 (m, 3H, CH3), 2.83–2.97 (m, 1H, H(4a)), 3.08–3.17 (m, 1H, H(4b)), 4.35–4.65 (m, 3H, H(1a) + H(1b) + H(3)) 7.02–7.19 (m, 4H, Ar), 7.30–7.41 (m, 2H, Ar), 7.64–7.80 (m, 2H, Ar), 12.28 (s, 1H, NH). 13C NMR (DMSO-d6, ppm, 25 °C): (two rotamers) δ 21.1, 27.6, 28.1, 31.1, 44.0, 44.9, 54.0, 55.3, 79.5, 79.9, 125.9, 126.6, 126.8, 127.1, 127.3, 127.7, 129.5, 129.6, 131.7, 132.6, 133.8, 134.9, 136.3, 144.1, 144.4, 153.8, 154.4, 170.4, 170.7. Anal. Calcd for C22H26N2O5S (430.5): C, 61.38; H, 6.09; N, 6.51; S, 7.45. Found: C, 61.46; H, 6.02; N, 6.54; S, 7.40.
(S)-N-[(1R)-1-Phenylethyl]-2-(tert-butoxycarbonyl)-1,2,3,4-tetrahydroisoquinoline-3-carboxamide (1f): Method A; white crystals; mp 137–139 °C (EtOAc/hexane; 1/1); yield: 1.8 g (64%); [α]D20 –77.5 (c 1.0, MeOH). 1H NMR (DMSO-d6, ppm, 75 °C): δ 1.27 (d, 3H, CH3, 3J = 7.41 Hz), 1.41 (s, 9H, C(CH3)3), 3.02–3.13 (m, 2H, H(1a) + H(1b)), 4.41–4.45 (m, 1H, H(3)), 4.54–4.58 (m, 2H, H(4a) + H(4b)), 4.84 (q, 1H, CH3CHPh, 3J = 7.41 Hz), 7.15–7.25 (m, 9H, Ar), 7.82 (d, 1H, NH, 3J = 7.42 Hz). 13C NMR (DMSO-d6, ppm, 25 °C): (two rotamers) δ 22.0, 22.2, 27.9, 28.0, 31.8, 32.0, 44.0, 47.4, 53.1, 55.0, 79.0, 79.2, 125.4, 125.7, 125.8, 126.3, 126.4, 126.7, 127.2, 128.0, 132.5, 133.7, 133.8, 135.0, 144.4, 154.2, 154.6, 169.9, 170.4. Anal. Calcd for C23H28N2O3 (380.5): C, 72.60; H, 7.42; N, 7.36. Found: C, 72.75; H, 7.51; N, 7.20.
(S)-N-[(1S)-1-Phenylethyl]-2-(tert-butoxycarbonyl)-1,2,3,4-tetrahydroisoquinoline-3-carboxamide (1g): Method A; white crystals; mp 138–140 °C (EtOAc/hexane; 1/1); yield: 1.6 g (59%); [α]D20 +36.8 (c 1.0, MeOH). 1H NMR (DMSO-d6, ppm, 75 °C): δ 1.27–1.43 (m, 12H, C(CH3)3 + CH3), 3.02–3.13 (m, 2H, H(4a) + H(4b)), 4.45–4.56 (m, 3H, H(1a) + H(1b) + H(3)), 4.79–4.87 (q, 1H, CH3CHPh, 3J = 7.05 Hz), 7.07–7.09 (m, 2H, Ar), 7.13–7.23 (m, 7H, Ar), 7.77 (d, 1H, NH, 3J = 6.69 Hz). 13C NMR (DMSO-d6, ppm, 25 °C): (two rotamers) δ 21.9, 22.1, 27,9, 28.1, 31.8, 32.2, 44.3, 44.9, 47.5, 53.6, 55.0, 79.0, 79.4, 125.7, 125.8, 126.0, 126.3, 126.6, 126.8, 127.3, 127.9, 133.1, 133.5, 134.0, 134.8, 144.2, 154.3, 154.6, 170.1, 170.5. Anal. Calcd for C23H28N2O3 (380.5): C, 72.60; H, 7.42; N, 7.36. Found: C, 72.85; H, 7.46; N, 7.23.
General method for preparation of thioamides 1h, 1i.
The mixture of the corresponding amide 1f or 1g (1.3 mmol), Lawesson reagent (2,4-bis(4-methoxyphenyl)-1,3,2,4-dithiadiphosphetane-2,4-dithione; 283 mg; 0.7 mmol) in dry toluene (20 mL) was heated at the temperature of 110 °C for 6 h and then evaporated. The residue was purified through chromatography column (SiO2, CH2Cl2) and the solid residue was recrystallized from EtOAc/hexane (1/1).
(S)-N-[(1R)-1-Phenylethyl]-2-(tert-butoxycarbonyl)-1,2,3,4-tetrahydroisoquinoline-3-thiocarbox-amide (1h): Yellow oil, yield: 260 mg (50%); [α]D20 –71.5 (c 3.1, MeOH). 1H NMR (DMSO-d6, ppm, 75 °C): δ 1.29 (d, 3H, CH3, 3J = 7.29 Hz), 1.37 (s, 9H, C((CH3)3), 3.14–3.21 (m, 2H, H(1a) + H(1b)), 4.48–4.59 (m, 2H, H(4a) + H(4b)), 4.81 (t, 1H, H(3), 3J = 6.29 Hz), 5.61 (q, 1H, CH3CHPh, 3J = 7.29 Hz), 7.13–7.31 (m, 9H, Ar), 9.56 (brs, 1H, NH). 13C NMR (DMSO-d6, ppm, 75 °C): δ 20.1, 20.4, 27.8, 28.1, 34.7, 35.1, 44.7, 45.6, 53.1, 61.4, 62.4, 79.4, 79.6, 126.1, 126.3, 126.6, 126.8, 127.0, 127.2, 127.2, 128.3, 128.4, 134.3, 135.9, 142.3, 154.2, 154.5,171.0, 202.4, 203.1. Anal. Calcd for C23H28N2O2S (380.5): C, 69.66; H, 7.12; N, 7.06; S, 8.09. Found: C, 69.75; H, 7.00; N, 7.14; S, 8.03.
(S)-N-[(1S)-1-Phenylethyl]-2-(tert-butoxycarbonyl)-1,2,3,4-tetrahydroisoquinoline-3-thiocarbox-amide (1i): Pale yellow crystals, mp 110–112 °C; yield: 270 mg (52%); [α]D20 –1.9 (c 1.0, MeOH). 1H NMR (DMSO-d6, ppm, 75 °C): δ 1.36 (s, 9H, C(CH3)3), 1.46 (d, 3H, CH3, 3J = 7.05 Hz), 3.19–3.30 (m, 2H, H(1a) + H(1b)), 4.51–4.61 (m, 2H, H(4a) + H(4b)), 4.85 (t, 1H, H(3), 3J = 6.04 Hz), 5.57 (q, 1H, CH3CHPh, 3J = 7.05 Hz), 6.96–6.98 (m, 2H, Ar), 7.14–7.28 (m, 7H, Ar), 9.58 (d, 1H, NH, 3J = 7.05 Hz). 13C NMR (DMSO-d6, ppm, 25 °C): (two rotamers) δ 19.9, 28.0, 35.1, 44.8, 53.2, 62.7, 79.3, 99.6, 126.1, 126.6, 127.3, 128.1, 134.2, 135.7, 141.9, 154.2, 202.6. Anal. Calcd for C23H28N2O2S (380.5): C, 69.66; H, 7.12; N, 7.06; S, 8.09. Found: C, 69.71; H, 7.05; N, 7.11; S, 8.01.
General method for deprotection of N-Boc intermediates.
The solution of N-Boc protected amides (1a-g) and thioamides (1h, i) was kept in trifluoroacetic acid (2 mL) at the temperature of 0 °C for 2 h. The residue obtained after evaporation of trifluoroacetic acid was dissolved in CH2Cl2 (30 mL). In the case of amides 1a-g, the organic phase was washed with water (1 × 20 mL), aqueous solution of NaOH (5%; 1 × 20 mL), saturated aqueous solution of NH4Cl (1 × 20 mL) and water (1 × 20 mL). In the case of thioamides 1h, 1i, the organic phase was mixed with dry Et3N (1 × 10 mL) and the formed salt was filtered off. After evaporation, the crude amides and thioamides were purified by flash column chromatography (SiO2; EtOAc) and recrystallized.
(S)-1,2,3,4-Tetrahydroisoquinoline-3-carboxamide (2a): From 1a (700 mg; 2 mmol); white crystals; mp 159–162 °C (EtOAc/hexane; 1/1); yield: 490 mg (70%); [α]D20 –31.5 (c 1.0, MeOH). 1H NMR (CDCl3, ppm, 25 °C): δ 1.76 (brs, 1H, NH), 2.87 (dd, 1H, H(4a), 2J = 16.28 Hz, 3J = 10.51 Hz), 3.24 (dd, 1H, H(4b), 2J = 16.28 Hz, 3J = 5.03 Hz), 3.59 (dd, 1H, H(3), 3J = 5.03 Hz, 3J = 10.51 Hz), 3.99–4.08 (m, 2H, H(1a) + H(1b)), 5.53 (brs, 1H, CONH2), 7.00–7.15 (m, 2H, CONH2 + Ar), 7.17–7.18 (m, 3H, Ar). 13C NMR (CDCl3, ppm, 25 °C): δ 29.7, 30.8, 47.7, 56.5, 125. 6, 126.3, 126.6, 129.3, 134.1, 135.7, 176.0. Anal. Calcd for C10H12N2O (176.2): C, 68.16; H, 6.86; N, 15.90. Found: C, 68.25; H, 6.77; N, 15.92.
(S)-N-Phenyl-1,2,3,4-tetrahydroisoquinoline-3-carboxamide (2b): From 1b (570 mg; 1.5 mmol); white crystals; mp 166–167 °C (EtOAc/hexane; 1/1); yield: 490 mg (70%); [α]D20 –86.0 (c 1.0, MeOH). 1H NMR (CDCl3, ppm, 25 °C): δ 1.72 (brs, 1H, NH), 2.87 (dd, 1H, H(4a), 2J = 16.44 Hz, 3J = 10.64 Hz), 3.30 (dd, 1H, H(4b), 2J = 16.44 Hz, 3J = 5.16 Hz), 3.62 (dd, 1H, H(3), 3J = 5.16 Hz, 3J = 10.64 Hz), 3.96–4.05 (m, 2H, H(1a) + H(1b)), 7.04–7.10 (m, 2H, Ar), 7.14–7.20 (m, 3H, Ar), 7.31 (t, 2H, Ar, 3J = 7.26 Hz), 7.60 (d, 2H, Ar, 3J = 7.26 Hz), 9.31 (brs, 1H, CONH). 13C NMR (CDCl3, ppm, 25 °C): δ 30.4, 47.4, 56.7, 119.3, 124.0, 125.4, 126.7, 128.9, 129.1, 134.3, 136.0, 137.6, 171.2. Anal. Calcd for C15H26N2O (252.3): C, 76.16; H, 6.39; N, 11.10. Found: C, 75.92; H, 6.69; N, 11.19.
(S)-N-(Pyridine-2-yl)-1,2,3,4-tetrahydroisoquinoline-3-carboxamide (2c): From 1c (300 mg; 0.8 mmol); white crystals; mp 108–109 °C (EtOAc/hexane; 1/1); yield: 490 mg (71%); [α]D20 –59.4 (c 1.0, MeOH). 1H NMR (CDCl3, ppm, 25 °C): δ 1.83 (brs, 1H, NH), 2.89 (dd, 1H, H(4a), 2J = 16.06 Hz, 3J = 10.29 Hz), 3.28 (dd, 1H, H(4b), 2J = 16.06 Hz, 3J = 5.33 Hz), 3.66 (dd, 1H, H(3), 3J = 5.33 Hz, 3J = 10.29 Hz), 3.96–4.04 (m, 2H, H(1a) + H(1b)), 6.99–7.06 (m, 2H, Ar), 7.14–7.17 (m, 3H, Ar), 7.64–7.68 (m, 1H, Ar), 8.23–8.27 (m, 2H, Ar), 9.88 (brs, 1H, CONH). 13C NMR (CDCl3, ppm, 25 °C): δ 30.5, 47.4, 56.8, 113.4, 119.9, 125.7, 126.5, 126.8, 129.2, 134.3, 136.4, 138.4, 148.1, 151.2, 172.2. Anal. Calcd for C15H15N3O (253.3): C, 71.13; H, 5.97; N, 16.59. Found: C, 70.87; H, 5.84; N, 16.36.
(S)-N-Benzyl-1,2,3,4-tetrahydroisoquinoline-3-carboxamide (2d): From 1d (600 mg; 1.6 mmol); white crystals; mp 103–105 °C (EtOAc/hexane; 1/1); yield: 320 mg (73%); [α]D20 –79.2 (c 1.0, MeOH). 1H NMR (CDCl3, ppm, 25 °C): δ 1.66 (brs, 1H, NH), 2.88 (dd, 1H, H(4a), 2J = 16.22 Hz, 3J = 10.30 Hz), 3.27 (dd, 1H, H(4b), 2J = 16.22 Hz, 3J = 4.99 Hz), 3.61 (dd, 1H, H(3), 3J = 4.99 Hz, 3J = 10.30 Hz), 3.93–4.02 (m, 2H, H(1a) + H(1b)), 4.42–4.52 (m, 2H, CH2Ph), 7.03–7.05 (m, 1H, Ar), 7.14–7.21 (m, 3H, Ar), 7.23–7.35 (m, 5H, Ar), 7.58 (brs, 1H, CONH). 13C NMR (CDCl3, ppm, 25 °C): δ 31.0, 43.1, 47.5, 56.4, 125.5, 126.6, 127.7, 128.6, 129.2, 134.4, 136.0, 138.3, 173.0. Anal. Calcd for C15H18N2O (266.34): C, 76.66; H, 6.81; N, 10.52. Found: C, 76.51; H, 6.80; N, 10.41.
(S)-N-[(4-Methylphenyl)sulfonyl]-1,2,3,4-tetrahydroisoquinoline-3-carboxamide (2e): From 1e (300 mg; 0.7 mmol); yellowish oil; yield: 320 mg (74%); [α]D20 –55.7 (c 1.0, MeOH). 1H NMR (CDCl3, ppm, 25 °C): δ 2.28 (s, 3H, CH3), 3.07–3.23 (m, 2H, H(4a) + H(4b)), 4.02–4.05 (m, 1H, H(3)), 4.24–4.28 (m, 1H, H(1a)), 4.40–4.44 (m, 1H, H(1b)), 6.98–7.06 (m, 4H, Ar), 7.16–7.26 (m, 2H, Ar), 7.32–7.50 (m, 2H, Ar), 8.34 (brs, 1H, NH). 13C NMR (CDCl3, ppm, 25 °C): δ 21.4, 29.7, 30.3, 45.0, 57.3, 126.3, 126.5, 127.0, 127.8, 128.5, 128.7, 129.0, 131.9, 139.8, 141.8, 173.5. Anal. Calcd for C17H18N2O3S (330.40): C, 61.80; H, 5.49; N, 8.48; S, 9.70. Found: C, 61.61; H, 5.91; N, 7.95; S, 9.79.
(S)-N-[(1R)-1-Phenylethyl]-1,2,3,4-tetrahydroisoquinoline-3-carboxamide (2f): From 1f (600 mg; 1.6 mmol); white crystals; mp 124–126 °C (EtOAc/hexane; 1/1); yield: 300 mg (69%); [α]D20 –118.0 (c 1.0, MeOH). 1H NMR (CDCl3, ppm, 25 °C): δ 1.49 (d, 3H, CH3, 3J = 6.78 Hz), 1.68 (brs, 1H, NH), 2.86 (dd, 1H, H(4a), 2J = 16.32 Hz, 3J = 10.56 Hz), 3.24 (dd, 1H, H(4b), 2J = 16.53 Hz, 3J = 5.01 Hz), 3.53 (dd, 1H, H(3), 3J = 5.01 Hz, 3J = 10.56 Hz), 4.00 (s, 2H, H(1a) + H(1b)), 5.14 (q, 1H, CH3CHPh, 3J = 7.28 Hz), 7.04–7.05 (m, 1H, Ar), 7.14–7.20 (m, 3H, Ar), 7.26–7.29 (m, 1H, Ar), 7.33–7.37 (m, 4H, Ar), 7.46 (d, 1H, CONH, 3J = 7.50 Hz). 13C NMR (CDCl3, ppm, 25 °C): δ 22.0, 26.9, 31.0, 47.6, 48.3, 56.5, 125.6, 126.2, 126.6, 127.3, 128.6, 129.2, 134.3, 135.8, 143.3, 172.3. Anal. Calcd for C18H20N2O (280.4): C, 77.11; H, 7.19; N, 9.99. Found: C, 76.92; H, 7.18; N, 9.72.
(S)-N-[(1S)-1-Phenylethyl]-1,2,3,4-tetrahydroisoquinoline-3-carboxamide (2g): From 1g (570 mg; 1.5 mmol); white crystals; mp 124–126 °C (EtOAc/hexane; 1/1); yield: 290 mg (68%); [α]D20 +42.2 (c 1.0, MeOH). 1H NMR (CDCl3, ppm, 25 °C): δ 1.50 (d, 3H, CH3, 3J = 6.61 Hz), 1.64 (brs, 1H, NH), 2.79 (dd, 1H, H(4a), 2J = 16.53 Hz, 3J = 9.92 Hz), 3.31 (dd, 1H, H(4b), 2J = 16.53 Hz, 3J = 4.41 Hz), 3.57 (dd, 1H, H(3), 3J = 4.41 Hz, 3J = 9.92 Hz), 3.92–4.02 (m, 2H, H(1a) + H(1b)), 5.13 (q, 1H, CH3CHPh, 3J = 7.05 Hz), 7.02–7.04 (m, 1H, Ar), 7.11–7.18 (m, 3H, Ar), 7.22–7.24 (m, 3H, Ar), 7.28–7.32 (m, 2H, Ar), 7.52 (d, 1H, CONH, 3J = 8.03 Hz). 13C NMR (CDCl3, ppm, 25 °C): δ 22.0, 30.1, 47.5, 48.1, 56.4, 125.5, 155.9, 126.1, 127.1, 128.6, 129.1, 134.4, 135.9, 143.3, 172.1. Anal. Calcd for C18H20N2O (280.4): C, 77.11; H, 7.19; N, 9.99. Found: C, 76.98; H, 7.11; N, 9.78.
(S)-N-[(1R)-1-Phenylethyl]-1,2,3,4-tetrahydroisoquinoline-3-thiocarboxamide (2h): From 1h (270 mg; 0.7 mmol); yellow oil; yield: 160 mg (77%); [α]D20 –119.9 (c 2.0, MeOH). 1H NMR (CDCl3, ppm, 25 °C): δ 1.49 (d, 3H, CH3, 3J = 7.03 Hz), 1.71 (s, 1H, NH), 2.85 (dd, 1H, H(4a), 2J = 15.79 Hz, 3J = 9.81 Hz), 3.49 (dd, 1H, H(4b), 2J = 15.79 Hz, 3J = 5.28 Hz), 3.79 (dd, 1H, H(3), 3J = 5.28 Hz, 3J = 9.81 Hz), 3.83–3.94 (m, 2H, H(1a) + H(1b)), 5.71 (q, 1H, CH3CHPh, 3J = 6.90 Hz), 7.02–7.04 (m, 1H, Ar), 7.11–7.18 (m, 3H, Ar), 7.23–7.29 (m, 1H, Ar), 7.30–7.35 (m, 4H, Ar), 9.72 (d, 1H, CSNH, 3J = 6.27 Hz). 13C NMR (CDCl3, ppm, 25 °C): δ 20.2, 34.5, 46.6, 53.1, 62.6, 125.1, 126.1, 126.2, 126.6, 127.5, 128.4, 128.5, 134.6, 136.1, 141.2, 202.2. Anal. Calcd for C18H20N2S (296.4): C, 72.93; H, 6.80; N, 9.45; S, 10.82. Found: C, 72.99; H, 6.73; N, 9.53; S, 10.74.
(S)-N-[(1S)-1-Phenylethyl]-1,2,3,4-tetrahydroisoquinoline-3-thiocarboxamide (2i): From 1i (700 mg; 1.8 mmol); white crystals, mp 110–112 °C (propan-2-ol/hexane, 2/1); yield: 370 mg (69%); [α]D20 +28.0 (c 1.0, MeOH). 1H NMR (CDCl3, ppm, 25 °C): δ 1.59 (d, 3H, CH3, 3J = 6.75 Hz), 1.76 (brs, 1H, NH), 2.93 (dd, 1H, H(4a), 3J = 9.03 Hz, 2J = 15.76 Hz), 3.52 (dd, 1H, H(4b), 2J = 15.76 Hz, 3J = 5.75 Hz), 3.89–4.03 (m, 3H, H(1a) + H(1b) + H(3)), 5.71 (q, 1H, CH3CHPh, 3J = 7.06 Hz), 7.07–7.11 (m, 3H, Ar), 7.16–7.22 (m, 3H, Ar), 7.24–7.30 (m, 3H, Ar), 9.81 (brs, 1H, CSNH). 13C NMR (CDCl3, ppm, 25 °C): δ 20.4, 34.5, 46.7, 53.1, 52.6, 125.3, 126.1, 126.3, 127.3, 126.6, 134.8, 136.2, 141.5, 202.5. Anal. Calcd for C18H20N2S (296.4): C, 72.93; H, 6.80; N, 9.45; S, 10.82. Found: C, 72.81; H, 6.79; N, 9.35; S, 10.72.
Preparation of cyclic compounds 3a, 3b.
(5S)-3(4-Nitrophenyl)-2-[(1R)-1-phenylethyl)]-2,3,10,10a-tetrahydro-5H-imidazo[1,5-b]isoquinoline-1-thione (3a). Compound 3a was prepared by reaction of compound 2i (30 mg; 0.1 mmol) with 4-nitrobenzaldehyde (16.5 mg; 0.11 mmol) in CH2Cl2 (1 mL) in the presence of molecular sieve 4Å for a reaction period of 3 days. Then the reaction mixture was evaporated, and the product was obtained by column chromatography (SiO2, EtOAc/petroleum ether, 1/2). Yield: 28.6 mg (66%), mp 82–83 °C; [α]D20 –31.9 (c 1.0, MeOH). 1H NMR (CDCl3, ppm, 25 °C): δ 1.56 (d, 3H, CH3, 3J = 6.54 Hz), 1.23 (dd, 1H, 2J = 11.75 Hz, 2J = 16.72 Hz), 3.45–3.54 (m, 3H), 3.84 (d, 1H, 2J = 14.54 Hz), 4.25 (q, 1H, CH3CHPh, 3J = 6.54 Hz), 5.10 (s, 1H), 6.91–6.93 (m, 1H, Ar), 7.11–7.15 (1H, Ar), 7.18–7.21 (m, 1H, Ar), 7.23–7.27 (m, 2H, Ar), 7.32–7.36 (m, 2H, Ar), 7.39–7.41 (m, 2H, Ar), 7.74–7.77 (m, 2H, Ar), 8.23–8.25 (m, 2H, Ar). 13C NMR (CDCl3, ppm, 25 °C): δ 24.0, 34.0, 53.6, 66.8, 67.1, 73.5, 124.1, 126.2, 126.4, 126.8, 126.9, 127.1, 128.5, 129.3, 129.4, 144.3, 145.0, 148.4, 165.5. Anal. Calcd for C25H23N3O2S (429.5): C, 69.91; H, 5.45; N, 9.78; S, 7.47. Found: C, 69.75; H, 5.38; N, 9.69; S, 7.41.
(5S)-3,3-Dimethyl-2-[(1R)-1-phenylethyl)]-2,3,10,10a-tetrahydro-5H-imidazo[1,5-b]isoquinoline-1-thione (3b). Compound 3b was prepared by reaction of compound 2i (30 mg; 0.1 mmol) with acetone (1 mL; 13 mmol) for a reaction period of 7 days. Then the mixture was evaporated to provide the crude product in almost quantitative yield. 1H NMR (CDCl3, ppm, 25 °C): δ 1.19 (s, 3H, CH3), 1.26 (s, 1H), 1.45 (s, 3H, CH3), 1.92 (q, 3H, CH3CHPh, 3J = 7.50 Hz), 2.63 (s, 1H), 3.03 (dd, 1H, 3J = 10.70 Hz, 2J = 15.75 Hz), 3.45 (dd, 1H, 3J = 4.19 Hz, 2J = 16.19 Hz), 3.68 (dd, 1H, 3J = 4.34 Hz, 2J = 11.13 Hz), 3.84 (s, 1H), 7.06–7.38 (m, 9H, Ar). Anal. Calcd for C21H24N2S (336.5): C, 74.96; H, 7.19; N, 8.33; S, 9.53. Found: C, 74.82; H, 6.96; N, 8.54; S, 9.61.
Asymmetric Aldol reaction.
To a solution of 4-nitrobenzaldehyde (30 mg; 0.2 mmol) in acetone or aqueous acetone (2 mL), catalyst 2a-i was added (0.04 mmol) and the resulting mixture was stirred at the temperature of 25 °C or –10 °C for a time period of 7 days. The residue formed after evaporation of the solvent was analyzed by 1H NMR. The crude product was purified by column chromatography (SiO2, EtOAc), and the product obtained was analyzed by HPLC. Daicel Chiralpak AS-H (250 × 4.6 mm), n-hexane/i-PrOH 70:30; flow rate 0.5 mL min–1; λ = 254 nm; tR (R) = 25.53 min; tR (S) = 33.42 min.
Crystallographic data for compound 2h.
The single crystals of 2h suitable for X-ray diffraction were obtained by slow evaporation from saturated solution in EtOAc/n-hexane. The X-Ray data for light yellow crystals were obtained at 150 K using Oxford Cryostream low-temperature device on a Nonius KappaCCD diffractometer with Mo Kα radiation (λ = 0.71073 Å), a graphite monochromator, and the φ and χ scan mode. Data reductions were performed with DENZO-SMN.19 The absorption was corrected by integration methods.20 Structures were solved by direct methods (Sir92)21 and refined by full matrix least-square based on F2 (SHELXL97).22 Hydrogen atoms were mostly localized on a difference Fourier map, however to ensure uniformity of treatment of crystal, all hydrogen were recalculated into idealized positions (riding model) and assigned temperature factors Hiso(H) = 1.2 Ueq (pivot atom) or of 1.5Ueq (methyl). H atoms in methyl, methylene, methine moieties and hydrogen atoms in aromatic rings were placed with C–H distances of 0.96, 0.97, 0.98 and 0.93Å and H atoms for N–H were assigned according to maxima on the electron density difference Fourier map. Rint = ∑Fo2 – Fo,mean2/∑Fo2, GOF = [∑(w(Fo2 – Fc2)2)/(Ndiffrs – Nparams)]½ for all data, R(F) = ∑Fo – Fc/∑Fofor observed data, wR(F2) = [∑(w(Fo2 – Fc2)2)/(∑w(Fo2)2)]½ for all data. Crystallographic data have been deposited with the Cambridge Crystallographic Data Centre (CCDC# 1001485). Copies of the data can be obtained free of charge on application to the CCDC, 12 Union Road, Cambridge CB21EZ, UK (fax: (+44)-1223-336-033; e-mail: deposit@ccdc.cam.ac.uk or www: http:// www.ccdc.cam.ac.uk). Crystallographic data: C18H20N2S, M = 296.42, a = 5.8660(4), b = 8.6230(7), c = 31.1701(18) Å, α = β = γ = 90 °, Z = 4, V = 1576.66(19) Å3, Dc = 1.249 g.cm-3, µ = 0.201 mm–1, Tmin/Tmax = 0.963/0.984; –6 ≤ h ≤ 7, –10 ≤ k ≤ 11, –32 ≤ l ≤ 40; 7205 reflections measured (θmax = 27.5 °), 7164 independent (Rint = 0.0463), 2888 with I > 2σ(I), 190 parameters, S = 1.160, R1(obs. data) = 0.0480, wR2(all data) = 0.0886; max., min. residual electron density = 0.240, –0.286 eǺ–3. The compound 2h crystallizes in the orthorhombic chiral space group P212121 with 4 molecules within the crystal lattice. The supramolecular architecture of the molecule consists of infinite chains where the molecules are interconnected by N–H···S short contacts. The values found for interatomic distances and angles are within the typical range for single and double bonds, respectively,23 and also comparable to similar structures of appropriate compounds reported elsewhere.24
ACKNOWLEDGEMENTS
This research work was financially supported by the Ministry of Education, Youth and Sports of the Czech Republic, Project CZ.1.07/2.3.00/30.0021 “Strengthening of Research and Development Teams at the University of Pardubice”.
References
1. K. Hayashi, Y. Ozaki, K. Nunami, and N. Yoneda, Chem. Pharm. Bull., 1983, 31, 312.
2. G. Balboni, S. Salvadori, E. D. Marczak, B. I. Knapp, J. M. Bidlack, L. H. Lazarus, X. M. Peng, Y. G. Si, and J. L. Neumeyer, Eur. J. Med. Chem., 2011, 46, 799. CrossRef
3. E. Mannekens, M. Crisma, S. Van Cauwenberghe, and D. Tourké, Eur. J. Org. Chem., 2003, 3300. CrossRef
4. Y. Zhang, J. Feng, C. Liu, L. Zhang, J. Jiao, H. Fang, L. Su, X. Zhang, J. Zhang, M. Li, B. Wang, and W. Xu, Bioorg. Med. Chem., 2010, 18, 1761. CrossRef
5. S. R. Klopfenstein, A. G. Evdokimov, A.-O. Colson, N. T. Fairweather, J. J. Neuman, M. B. Maier, J. L. Gray, G. S. Gerwe, G. E. Stake, B. W. Howard, J. A. Farmer, M. E. Pokross, T. R. Downs, B. Kasibhatla, and K. G. Peters, Bioorg. Med. Chem. Lett., 2006, 16, 1574. CrossRef
6. E. Nuti, F. Casalini, S. Santamaria, P. Gabelloni, S. Bendinelli, E. Da Pozzo, B. Costa, L. Marinelli, V. La Pietra, E. Novellino, M. M. Bernardo, R. Fridman, F. Da Settimo, C. Martini, and A. Rossello, Eur. J. Med. Chem., 2011, 46, 2617. CrossRef
7. S. K. Chakka, P. G. Andersson, G. E. M. Maguire, H. G. Kruger, and T. Govender, Eur. J. Org. Chem., 2010, 972. CrossRef
8. . CrossRef
9. G. Xu and Z. Z. Liu, Chin. Chem. Lett., 2010, 21, 309. CrossRef
10. T. Naicker, K. Petzold, T. Singh, P. I. Arvidsson, H. G. Kruger, G. E. M. Maguire, and T. Govender, Tetrahedron: Asymmetry, 2010, 21, 2859. CrossRef
11. D. Gryko and R. Lipinski, Adv. Synth. Catal., 2005, 347, 1948. CrossRef
12. D. Gryko and R. Lipinski, Eur. J. Org. Chem., 2006, 3864.
13. D. Gryko, M. Zimnicka, and R. Lipinski, J. Org. Chem., 2007, 72, 964. CrossRef
14. S. Nordhoff, S. Cerezo-Gálvez, A. Feurer, O. Hill, V. G. Matassa, G. Metz, C. Rummey, M. Thiemann, and P. J. Edwards, Bioorg. Med. Chem. Lett., 2006, 16, 1774. CrossRef
15. F. G. Bordwell, D. J. Algrim, and J. A. Harrelson, J. Am. Chem. Soc., 1988, 110, 5903. CrossRef
16. P. M. Pihko, I. Majander, and A. Erkkilä, ′Enamine Catalysis′, in ′Asymmetric Organocatalysis′, ed. by B. List, Springer, Heidelberg, 2009, pp. 33–40. CrossRef
17. K. Sakthivel, W. Notz, T. Bui, and C. F. Barbas III, J. Am. Chem. Soc., 2001, 123, 5260. CrossRef
18. D. Seebach, A. K. Beck, D. M. Badine, M. Limbach, A. Eschenmoser, A. M. Treasurywala, R. Hobi, W. Prikoszovich, and B. Linder, Helv. Chim. Acta, 2007, 90, 425. CrossRef
19. Z. Otwinowski and W. Minor, Methods Enzymol., 1997, 276, 307. CrossRef
20. P. Coppens, ′Crystallographic Computing′, ed. by F. R. Ahmed, S. R. Hall, and C. P. Huber, Munksgaard, Copenhagen, 1970, pp. 255–270.
21. A. Altomare, G. Cascarano, C. Giacovazzo, and A. Guagliardi, J. Appl. Crystallogr., 1994, 27, 1045. CrossRef
22. G. M. Sheldrick, ′SHELXL-97′, University of Göttingen, Göttingen, 2008.
23. F. H. Allen, O. Kennard, D. G. Watson, L. Brammer, A. G. Orpen, and R. Taylor, J. Chem. Soc., Perkin Trans. 2, 1987, S1. CrossRef
24. T. Naicker, T. Govender, H. G. Kruger, and G. E. M. Maguire, Acta Crystallogr., Sect. E: Struct. Rep. Online, 2011, 67, o3441. CrossRef