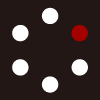
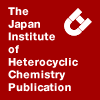
HETEROCYCLES
An International Journal for Reviews and Communications in Heterocyclic ChemistryWeb Edition ISSN: 1881-0942
Published online by The Japan Institute of Heterocyclic Chemistry
e-Journal
Full Text HTML
Received, 27th May, 2014, Accepted, 9th June, 2014, Published online, 13th June, 2014.
DOI: 10.3987/COM-14-13036
■ Design, Synthesis and Antiproliferative Activity Evaluation of New 5-Azaisoindigo Derivatives
Ping Zhao, Yun Yan, Yanzhong Li, Aiying Zhang, Xiaoping Zhan, Zenglu Liu, Zhenmin Mao,* Shaoxiong Chen, and Liqun Wang
School of Pharmacy, Shanghai Jiaotong University , No.800 Dongchuan Road, Minhang District, Shanghai 200240, China
Abstract
New 5-azaisoindigo derivatives were synthesized with two key intermediates 5-azaoxindole (7) and substituted indole-2,3-dione (10) in this paper. Intermediate 7 was prepared using 3-methylpridine (1) as the starting material via oxidation, nitration, condensation and cyclization, bromination and reduction reactions. Intermediate 10 was obtained by a convenient Sandmeyer’s method. The target compounds 5-azaisoindigo derivatives 11a-f were obtained by condensation of these two intermediates 7 and 10 in acidic condition. All target compounds were evaluated for their antiproliferative activity against seven cell lines by SRB assay. Compounds 11e and 11f showed significant antiproliferative activity against K562 cells (IC50: 8.9 µM and 13.6 µM, respectively).Indirubin (Figure 1), an ingredient of Indigo naturalis, has been used to treat chronic myeloid leukemia (CML) in clinic.1 Studies have revealed that indirubin derivatives inhibit cyclin-dependent kinases (CDKs), a family of vital cell-cycle regulators, through competing with ATP for binding to the catalytic site of the kinase.2,3 Indirubin derivatives are also strong inhibitors of glycogen synthase kinase 3β (GSK-3β), which associated with a host of high-profile diseases such as cancer, diabetes, neurodegeneration and inflammation due to its involvement in a great number of signaling pathways.4-7 However, indirubin can cause serious gastrointestinal reactions due to the poor water solubility, and long-term use may lead to bone marrow suppression and drug-resistance.8 The development of new indirubin derivatives with improved pharmacological properties and reduced toxicities have become a pursuing aim in this area. Many studies have been carried out by a group of scientists of Chinese Academy of Medical Sciences.9,10 Among them, meisoindigo (1-methylisoindigo, Figure 1), the methylation derivative of isoindigo (Figure 1), exhibited a higher solubility and antitumor activity and fewer adverse side effects than indirubin.11,12 Meisoindigo has replaced indirubin and become a crucial chemotherapy drug in treatment of CML.
Studies also indicate that the presence of a nitrogen atom in pyridine ring in this bis-indole coupling compounds can change the distribution of electron cloud, enhance the binding capacity to acceptors, and can also improve the solubility by pyridination and be beneficial to druggability.13,14 Much synthesis and biological activity researches on 7-azaindirubin and 7-azaisoindigo (Figure 1) derivatives have been carried out by scientists,8,15-17 but fewer on 5-azaisoindigo (Figure 1) derivatives.18 We described here a synthetic route for 5-azaisoindigo derivatives and obtained a series of 5-azaisoindigo derivatives that have never been reported before. Their antiproliferative activity against a series of cancer cell lines and a normal cell line were evaluated by Sulforhodamine B (SRB) assay.
Chemistry.
The target compounds 5-azaisoindigo derivatives 11a-f were synthesized according to the method described in Scheme 1.
As shown in Scheme 1, 5-azaoxindole (7) and substituted indole-2,3-dione (10) were the two key intermediates in this strategy. Intermediate 7 was obtained from 3-methylpyridine (1) through 6 reactions containing oxidation and so on. Each step was successfully achieved with a yield 80-96% and the overall yield was about 49%. The second intermediate 10 was obtained by a reported Sandmeyer’s method.19,20 In this reported method, the starting material aniline or substituted aniline are commercially readily available and inexpensive. Two-step reaction was also featured by simple operation and good yield. After that, these two moieties 7 and 10 were coupled by condensation into 5-azaisoindigo derivatives under acidic conditions with good yield. Although the intermediates 7 and 10 were the known compounds which obtained using common reactions, they made the synthesis of target compounds, novel 5-azaisoindigo derivatives very convenient and successful. Altogether, the present method was featured by availability of raw material, low synthesis cost and good yield. It represents a practical approach to the synthesis of 5-azaisoindigos and related compounds.
Antiproliferative activity evaluation.
The results have been shown in Table 1. It can be seen that most of the compounds showed no obvious inhibitory effect on normal human cells (HMVEC) but showed potential antiproliferative activity to six cancer cell lines, especially to two leukemia cell lines K562 and L1210, two solid cancer cell lines HCT-116 and MGC80-3. Compounds 11e and 11f exhibited significant activity against K562 cells with IC50 8.9 and 13.6 µM, respectively, which were lower than the control compound meisoindigo. Compounds 11a, 11b, 11c and 11d showed better or similar antiproliferative activity against L1210 cells compared with meisoindigo. Compound 11e also showed an inhibitory activity to MGC80-3 cells with IC50 40.5 µM, which was lower than meisoindigo. These results indicate that chlorine substituted at 6 or 7 position of 5-azaisoindigo may be beneficial for the improvement of inhibitory activity to K562 and MGC80-3 cells.
EXPERIMENTAL
Chemistry.
Analytical grade solvents and commercially available reagents were used without further purification. Flash column chromatography was performed on a column packed with silica gel 60 (200-300 mesh). Melting points were determined on an SGW X-4 digital micro-melting point apparatus. 1H NMR (400 MHz) and 13C NMR (100 MHz) spectra were recorded on a Bruker Avance 400 spectrometer with DMSO-d6, CDCl3 or Pyridine-d5 as solvent and TMS as internal standard. High resolution mass spectra (HRMS) data were obtained on an AB SCIEX TripleTOF® 4600 mass spectrometer using electrospray ionization (ESI) technique. The procedure has been shown in Scheme 1.
3-Methylpyridine-1-oxide (2)
3-Methylpyridine (1, 4.0 g, 43 mmol) was added into a round bottom flask containing acetic acid (30 mL). Then 6.5 mL of ice-cold peroxide (30% solution, 64.5 mmol) was added to the mixture. The resulting solution was stirred at 70 °C overnight. The reaction mixture was concentrated under vacuum and the residue was treated with aqueous Na2CO3 solution (40%) while maintaining the temperature at 0 °C. The mixture was then extracted with dichloro xmethane (DCM). The combined organic layer was washed with brine, dried over anhydrous sodium sulfate and concentrated under vacuum to get 3-methylpyridine- 1-oxide (4.6 g, 96%) as light brown oil. 1H NMR (400 MHz, DMSO-d6, δ ppm): 8.11 (s, 1H), 8.03 (d, 1H, J = 8.0 Hz), 7.29 (t, 1H, J = 10.0 Hz, J = 8.0 Hz), 7.16 (d, 1H, J = 10.0 Hz), 2.22 (s, 3H).
4-Nitro-3-methylpyridine-1-oxide (3)
Compound 2 (2.0 g, 18.3 mmol) was dissolved in 10 mL of concentrated H2SO4 and warmed to 60 °C. While keeping the temperature below 80 °C, 4 mL of 90% fuming HNO3 was added dropwise. The mixture was stirred at 80 °C for 3 h. Afterwards, the reaction mixture was cooled to room temperature and poured onto crushed ice. The cooled mixture was then neutralized with Na2CO3 until a yellow precipitate formed. This precipitate was filtered and dried in a vacuum oven to get compound 3 with 85% yield, mp 137-139 °C. 1H NMR (400 MHz, CDCl3, δ ppm): 8.14-8.11 (m, 2H), 8.03 (d, 1H, J = 9.2 Hz), 2.63 (s, 3H).
(E)-3-(2-(Dimethylamino)vinyl)-4-nitropyridine-1-oxide (4)
To the stirred solution of compound 3 (0.5 g, 3.2 mmol) in 3 mL of DMF was added 0.56 mL of N,N-dimethylformamide dimethyl acetal (DMF-DMA, 4.21 mmol) dropwise at room temperature. After complete addition, the reaction mixture was stirred at 90 °C for 4 h. The reaction mixture was poured into cold water and the precipitated solid was filtered, washed with cold water and dried to get compound 4 (650 mg, 96%) as brown violet solid. 1H NMR (400 MHz, CDCl3, δ ppm): 8.34 (d, 1H, J = 2.4 Hz), 7.90 (d, 1H, J = 10.0 Hz), 7.64 (dd, 1H, J = 10.0 Hz, J = 2.4 Hz), 7.12 (d, 1H, J = 17.6 Hz), 6.01 (d, 1H, J = 17.6 Hz), 3.03 (s, 6H).
1H-Pyrrolo[3,2-c]pyridine (5-Azaindole, 5)
210 mg (1 mmol) of compound 4 in 8 mL of EtOH were mixed with 350 mg of Raney nickel (50% in EtOH) and hydrogenated in hydrogen atmosphere firstly for 3 h at room temperature and then 2 h at 40 °C. The catalyst was removed by suction filtering and the filtrate was purified by flash chromatography (DCM/MeOH 3:1) to get compound 5 as pale white solid (97 mg, 83%). mp 110-112 °C; 1H NMR (400 MHz, DMSO-d6, δ ppm): 11.61 (s, 1H), 8.85 (s, 1H), 8.15 (d, 1H, J = 7.6 Hz), 7.48 (t, 1H, J = 4.4 Hz), 7.42 (d, 1H, J = 7.6 Hz), 6.61 (d, 1H, J = 3.3 Hz).
3,3,7-Tribromo-1H-pyrrolo[3,2-c]pyridin-2(3H)-one (6)
The bromination of compound 5 was carried out according to the literature previously reported.20 To a stirred solution of compound 5 (0.5 g, 4.23 mmol) in t-BuOH (30 mL) and H2O (30 mL) at room temperature was added dropwise neat Br2 (0.87 mL, 16.8 mmol) over 30 min. Afterwards, by the careful, slow addition of saturated aqueous NaHCO3 solution over 30 min, the pH of the mixture was adjusted to 6.5-7.0. During this period, precipitation of the yellow product became evident. The precipitates were filtered, washed with water and dried in the air to give compound 6 (1.25 g, 80%). mp>250; 1H NMR (400 MHz, DMSO-d6, δ ppm): 8.71 (s, 1H), 8.60 (s, 1H).
5-Azaoxindole (7)
To a solution of compound 6 (114 mg, 0.31 mmol) in acetic acid (3 mL) was added active zinc dust (200 mg, 3.1 mmol). The mixture was stirred at room temperature for 4 h. After the reaction completed, filtered the mixture, concentrated the filtrate under vacuum to remove the solvent and the residue was purified by flash chromatography (cyclohexane/EtOAc 1:1) to get compound 7 as pale solid (37 mg, 90%). 1H NMR (400 MHz, DMSO-d6, δ ppm): 10.95 (s, 1H), 8.30 (d, 1H, J = 8.0 Hz), 8.26 (s, 1H), 6.93 (d, 1H, J = 8.0 Hz), 3.58 (s, 2H).
General procedure for the synthesis of substituted indole-2,3-dione (10a-f)
The second key intermediate 10a-f were obtained by Sandmeyer's method.20,21 To the stirred mixture of H2O (56 mL), chloral hydrate (3.73 g, 22.6 mmol), anhydrous sodium sulfate (25.5 g, 179.7 mmol) was added aniline or various substituted aniline (8, 20.35 mmol) dissolved in 15 mL of H2O. The mixture was dissolved by stirring and heating at 35 °C. Then 2.2 mL of concentrated HCl and hydroxylamine hydrochloride (4.48 g, 69.5 mmol) in 20 mL of water were added to the mixture. The mixture was stirred at 80-90 °C for 2 h. Then, cooled down the mixture to 50 °C and filtered, washed the solid with water and dried at 40 °C overnight to get the corresponding compound 9.
Compound 9 (7.1 mmol ) was added to a round bottom flask containing 86% sulfuric acid (5 mL) and stirred at 60 °C for 50 min. Then the temperature was raised to 80 °C and maintained the reaction for 15 min. After that, cooled down the mixture to room temperature and poured into water, stirred and filtered, and washed the solid with water. The crude product was purified by flash chromatography (cyclohexane/ EtOAc 5:1-3:1) to get the corresponding compound 10a-f as orange solid.
Indole-2,3-dione (10a)
10a was obtained in 90% yield as an orange red solid; mp 203-205 °C; 1H NMR (400 MHz, DMSO-d6, δ ppm): 11.03 (br s, 1H), 7.58 (t, 1H, J = 10.4 Hz), 7.50 (d, 1H, J = 10.4 Hz), 7.06 (t, 1H, J = 10.4 Hz), 6.90 (d, 1H, J = 10.4 Hz).
5-Methylindole-2,3-dione (10b)
10b was obtained in 74% yield as an orange red solid; mp 187-188 °C; 1H NMR (400 MHz, CDCl3, δ ppm): 8.48 (br s, 1H, NH), 7.40 (m, 2H), 6.84 (d, 1H, J = 10.4 Hz), 2.33 (s, 3H).
6-Methylindole-2,3-dione (10c)
10c was obtained in 65% yield as an orange red solid; mp 182-184 °C; 1H NMR (400 MHz, DMSO-d6, δ ppm): 11.17 (br s, 1H), 7.52 (d, 1H, J = 8.0 Hz), 7.11 (d, 1H, J = 8.0 Hz), 6.93 (s, 1H).
7-Methylindole-2,3-dione (10d)
10d was obtained in 64% yield as an orange red solid; mp 266-268 °C; 1H NMR (400 MHz, DMSO-d6, δ ppm): 11.02 (br s, 1H), 7.41 (d, 1H, J = 10.0 Hz), 7.31 (d, 1H, J = 9.6 Hz), 6.97 (t, 1H, J = 10.0 Hz), 2.17 (s, 3H).
6-Chloroindole-2,3-dione (10e)
10e was obtained in 53% yield as an orange red solid; mp 259-261 °C; 1H NMR (400 MHz, DMSO-d6, δ ppm): 11.17 (br s, 1H), 7.53 (d, 1H, J = 8.0 Hz), 7.10 (d, 1H, J = 8.0 Hz), 6.93 (s, 1H).
7-Chloroindole-2,3-dione (10f)
10f was obtained in 50% yield as an orange red solid; mp 187-191 °C; 1H NMR (400 MHz, DMSO-d6, δ ppm): 11.46 (br s, 1H), 7.65 (d, 1H, J = 10.0 Hz), 7.48 (d, 1H, J = 10.0 Hz), 7.08 (t, 1H, J = 10.0 Hz).
General procedure for the synthesis of target compounds 11a-f
The target compounds 11a-f were obtained by condensation of intermediates 7 and 10 in acidic condition. To the stirred solution of compound 7 (0.43 mmol) and compound 10 (0.43 mmol) in acetic acid (8 mL) was added three drops of concentrated HCl. Under the protection of nitrogen, the mixture was stirred under reflux for 8-12 h and the product was precipitated from solution. After the reaction completed, the precipitate was filtered and washed with EtOAc and water and dried to get compounds 11a-f.
5-Azaisoindigo (11a)
11a was obtained in 78% yield as a dark brown solid; mp>300 °C; 1H NMR (400 MHz, DMSO-d6, δ ppm): 12.73 (br s, 1H), 11.36 (s, 1H), 10.11 (s, 1H), 9.08 (d, 1H, J = 10.4 Hz), 8.65 (d, 1H, J = 8.0 Hz), 7.47 (t, 1H, J = 8.0 Hz), 7.42 (d, 1H, J = 4.4 Hz), 7.06 (t, 1H, J = 7.6 Hz), 6.94 (d, 1H, J = 8.4 Hz); 13C NMR (100 MHz, DMSO- d6, δ ppm): 168.8, 168.5, 155.6, 149.2, 145.6, 143.3, 138.0, 135.0, 132.4, 130.2, 126.0, 121.8, 119.3, 110.4, 106.8. HRMS-ESI: m/z [M+H]+ calcd. for C15H9N3O2: 264.0768; found: 264.0779.
5’-Methyl-5-azaisoindigo (11b)
11b was obtained in 64% yield as a dark purple solid; mp>300 °C; 1H NMR (400 MHz, DMSO-d6, δ ppm): 12.67 (br s, 1H), 11.22 (s, 1H), 10.11 (s, 1H), 8.93 (s, 1H), 8.64 (d, 1H, J = 6.4 Hz), 7.40 (d, 1H, J = 6.0 Hz), 7.29 (d, 1H, J = 8.0 Hz), 6.83 (d, 1H, J = 7.6 Hz), 2.28 (s, 3H); 13C NMR (100 MHz, DMSO-d6, δ ppm): 168.8, 168.4, 155.7, 147.1, 143.5, 142.9, 139.2, 138.4, 135.5, 130.5, 125.5, 121.0, 119.3, 110.1, 106.8, 20.9. HRMS-ESI: m/z [M+H]+ calcd. for C16H11N3O2: 278.0924; found: 278.0928.
6’-Methyl-5-azaisoindigo (11c)
11c was obtained in 69% yield as a dark brown solid; mp>300 °C; 1H NMR (400 MHz, DMSO-d6, δ ppm): 12.78 (br s, 1H), 11.33 (s, 1H), 10.09 (s, 1H), 8.97 (d, 1H, J = 8.0 Hz), 8.65 (d, 1H, J = 6.4 Hz), 7.45 (d, 1H, J = 6.0 Hz), 6.88 (d, 1H, J = 9.6 Hz), 6.77 (s, 1H), 2.35 (s, 3H); 13C NMR (100 MHz, Pyridine-d5, δ ppm): 171.3, 170.8, 151.6, 150.9, 149.8, 146.7, 145.4, 136.5, 135.5, 131.4, 124.5, 123.4, 123.3, 111.4, 106.1, 22.5. HRMS-ESI: m/z [M+H]+ calcd. for C16H11N3O2: 278.0924; found: 278.0926.
7’-Methyl-5-azaisoindigo (11d)
11d was obtained in 67% yield as a dark purple solid; mp>300 °C; 1H NMR (400 MHz, DMSO-d6, δ ppm): 12.82 (br s, 1H), 11.20 (s, 1H), 10.08 (s, 1H), 8.91 (d, 1H, J = 10.8 Hz), 8.61 (d, 1H, J = 8.4 Hz), 7.41 (d, 1H, J = 8.4 Hz), 7.27 (d, 1H, J = 10.0 Hz), 6.94 (t, 1H, J = 8.4 Hz), 2.21 (s, 3H); HRMS-ESI: m/z [M+H]+ calcd. for C16H11N3O2: 278.0924; found: 278.0932.
6’-Chloro-5-azaisoindigo (11e)
11e was obtained in 73% yield as a dark brown solid; mp>300 °C; 1H NMR (400 MHz, DMSO-d6, δ ppm): 12.70 (br s, 1H), 11.65 (s, 1H), 10.06 (s, 1H), 9.08 (d, 1H, J = 9.2 Hz), 8.62 (d, 1H, J = 5.6 Hz), 7.39 (d, 1H, J = 6.0 Hz), 7.12 (d, 1H, J = 9.2 Hz), 7.10 (s, 1H); 13C NMR (100 MHz, DMSO-d6, δ ppm): 168.7, 168.5, 159.2, 152.1, 146.8, 142.2, 138.5, 131.5, 126.1, 122.6, 121.6, 119.7, 116.7, 112.3, 110.2. HRMS-ESI: m/z [M+H]+ calcd. for C15H8ClN3O2: 298.0378; found: 298.0381.
7’-Chloro-5-azaisoindigo (11f)
11f was obtained in 67% yield as a dark brown solid; mp>300 °C; 1H NMR (400 MHz, DMSO-d6, δ ppm): 12.76 (br s, 1H), 11.64 (s, 1H), 10.09 (s, 1H), 9.07 (d, 1H, J = 8.4 Hz), 8.66 (d, 1H, J = 7.2 Hz), 7.56 (d, 1H, J = 8.0 Hz), 7.42 (t, 1H, J = 6.0 Hz), 7.11 (d, 1H, J = 6.0 Hz); 13C NMR (100 MHz, DMSO-d6, δ ppm): 168.7, 168.5, 151.4, 150.0, 148.4, 141.8, 133.9, 132.7, 130.9, 128.1, 125.0, 123.0, 122.4, 113.9, 105.6. HRMS-ESI: m/z [M+H]+ calcd. for C15H8ClN3O2: 298.0378; found: 298.0382.
Antiproliferative activity evaluation.
Cell culture. K562, HCT-116, MGC80-3, L1210, A549, HeLa and HMVEC cells were cultured at 37 °C in an atmosphere containing 5% CO2, with RPMI 1640 medium (Hyclone, SH30027 LS, USA) supplemented with 10% heat-inactivated fetal bovine serum (Hyclone), 100 IU penicillin and 100µg/mL streptomycin.
Drug treatment. Compounds were dissolved in DMSO and stored at -20 °C. For each experiment, the stock solution was further diluted in medium to obtain the desired concentration. The final solvent concentration in cell culture was ≤0.5%, v/v of DMSO, which was found to be nontoxic to the cells. Parallel cultures of cells in medium with DMSO were used as controls.
Antiproliferation assay. The effects of target compounds on cells proliferation were examined by SRB assay.21,22 Cells suspension (5×103 cells/well) were seeded into 96-well plates and incubated for 24 h. The cells were then treated with different concentration of drugs in their respective culture medium and maintained with the treatment for additional 48 h. Thereafter, the cells were fixed with ice-cold trichloroacetic acid (TCA) for 1 h at 4 °C. The TCA solution was removed lightly and the plates were washed five times in distilled water and dried in the air. 50 μL of 0.4% SRB solution was added to each well and stained at room temperature for 30 min. The SRB solution was removed by washing the plates quickly with 1% (v/v) acetic acid, five times. The washed plates were dried in the air. 100 μL of 10 mM unbuffered Tris Base solution (pH 10.5) was added to each well to dissolve the bound SRB. The absorbance was measured at 570 nm using a plate reader (MultiScan MK3, Thermo).
ACKNOWLEDGEMENTS
The authors thank the National Significant and Special Project of New Created Drugs (No. 2010ZX09401-404) for financial support of this work. We also thank Instrumental Analysis Center of Shanghai Jiaotong University for spectral data.
References
1. R. Hoessel, S. Leclerc, J. Endicott, M. Nobel, A. Lawrie, P. Tunnah, M. Leost, E. Damiens, D. Marie, D. Marko, E. Niederberger, W. Tang, G. Eisenbrand, and L. Meijer, Nat. Cell Biol., 1999, 1, 60. CrossRef
2. P. Polychronopoulos, P. Magiatis, A. Skaltsounis, V. Myrianthopoulos, E. Mikros, A. Tarricone, A. Musacchio, S. Roe, L. Pearl, M. Leost, P. Greengard, and L. Meijer, J. Med. Chem., 2004, 47, 935. CrossRef
3. D. Marko, S. Schatzle, A. Friedel, A. Genzlinger, H. Zankl, L. Meijer, and G. Eisenbrand, Br. J. Cancer, 2001, 84, 283. CrossRef
4. E. Xingi, D. Smirlis, V. Myrianthopoulos, P. Magiatis, K. Grant, L. Meijer, E. Mikros, A. Skaltsounis, and K. Soteriadou, Int. J. Parasitol., 2009, 39, 289. CrossRef
5. L. Meijer, A. Skaltsounis, P. Magiatis, P. Polychronopoulos, M. Knockaert, M. Leost, X. Ryan, C. Vonica, A. Brivanlou, R. Dajani, C. Crovace, C. Tarricone, A. Musacchio, S. Roe, L. Pearl, and P. Greengard, Chem. Biol., 2003, 10, 1255. CrossRef
6. A. Martinez, A. Castro, I. Dorronsoro, and M. Alonso, Med. Res. Rev., 2002, 22, 373. CrossRef
7. M. Medina and A. Castro, Curr. Opin. Drug Disc., 2008, 11, 533.
8. Z. H. Wang, T. Wang, S. N. Yao, J. C. Chen, W. Y. Hua, and Q. Z. Yao, Arch. Pharm., 2010, 343, 160. CrossRef
9. X. J. Ji and F. R. Zhang, Acta Pharm. Sin., 1985, 20, 137.
10. K. M. Wu, M. Y. Zhang, Z. Fang, and L. Huang, Acta Pharm. Sin., 1985, 20, 821.
11. X. J. Zhang, F. R. Liu, and Y. Hu, Acta. Pham. Sin., 1985, 20, 247.
12. Z. J. Xiao, Y. S. Hao, B. C. Liu, and L. S. Qian, Leukemia & Lymphoma, 2002, 43, 1763. CrossRef
13. Z. H. Wang, W. Y. Li, F. L. Li, L. Zhang, W. Y. Hua, J. C. Cheng, and Q. Z. Yao, Chin. Chem. Lett., 2009, 20, 542. CrossRef
14. Q. Z. Yao, Z. H. Wang, J. C. Cheng, and W. Y. Hua, Patent. CN 101074229A, 2007.
15. Z. H. Wang, Y. Dong, T. Wang, M. H. Shang, W. Y. Hua, and Q. Z. Yao, Chin. Chem. Lett., 2010, 21, 297. CrossRef
16. M. Kritsanida, P. Magiatis, A. L. Skaltsounis, Y. Peng, P. Li, and L. P. Wennogle, J. Nat. Prod., 2009, 72, 2199. CrossRef
17. F. Bouchikhi, F. Anizon, and P. Moreau, Eur. J. Med. Chem., 2008, 43, 755. CrossRef
18. Q. Z. Yao, Z. H. Wang, and W. Y. Hua, Patent. EP2168964A1, 2010.
19. T. Sandmeyer, Helv. Chim. Acta, 1919, 2, 234. CrossRef
20. S. J. Garden, J. G. Torres, A. A. Ferreira, R. B. Silva, and A. C. Pinto, Tetrahedron Lett., 1997, 38, 1501. CrossRef
21. P. Skehan, R. Storeng, D. Scudiero, A. Monks, J. McMahon, D. Visteca, J. T. Warrem, H. Bokesch, S. Kenney, and N. R. Boyd, J. Nat. Cancer Inst., 1988, 82, 1107. CrossRef
22. S. H. Baek, H. J. Oh, J. A. Lim, H. J. Chun, H. O. Lee, J. W. Ahn, N. B. Perry, and H. M. Kim, Bull. Korean Chem. Soc., 2004, 25, 195 CrossRef