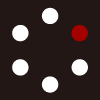
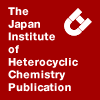
HETEROCYCLES
An International Journal for Reviews and Communications in Heterocyclic ChemistryWeb Edition ISSN: 1881-0942
Published online by The Japan Institute of Heterocyclic Chemistry
e-Journal
Full Text HTML
Received, 19th June, 2014, Accepted, 10th September, 2014, Published online, 19th September, 2014.
DOI: 10.3987/COM-14-S(K)39
■ An Efficient Domino Sonogashira/Double Carbopalladation/C–H-Activation Reaction Leading to Fluorescent Polycyclic Aromatic Hydrocarbons
Lutz F. Tietze* and Christoph Eichhorst
Institute of Organic and Biomolecular Chemistry, University of Göttingen, Tammannstr. 2, D-37077 Göttingen, Germany
Abstract
A facile synthesis of fluorescent polycyclic aromatic hydrocarbons through a highly productive palladium-catalyzed fourfold domino Sonogashira/double carbopalladation/C–H-activation process was developed.INTRODUCTION
Domino reactions allow the transformation of simple organic building blocks into complex functional structures within few steps.1 Beside this compelling characteristic of efficiency the concept is also reassuring due to its economic and environmental sustainability, since two or more reactions are included in one process without the need of any intermediate purification. Moreover, domino reactions can proceed via unstable intermediates, which is usually not possible in a step-wise approach. In addition to the development of domino Knoevenagel/hetero-Diels-Alder reactions,2 domino imine formation/hydride shift/alkylation reactions,3 domino amidation/spirocyclization/electrophilic aromatic substitution reactions4 and domino acetalization/allylation reactions,5 in the last years we have focused especially on palladium-catalyzed domino processes.6 In this aspect, it was one of our goals to include C–H-activation reactions7 in domino processes for the synthesis of natural products and functional materials. C–H activation reactions that are part of palladium-catalyzed domino processes have persuading advantages compared to typically used transition-metal catalyzed cross-coupling reactions, as no functionalization of the precursors is necessary.
Quite recently, we have developed a domino Sonogashira/carbopalladation/C–H-activation reaction for the synthesis of tetra-substituted helical alkenes as potential switches8 and domino Sonogashira/double carbopalladation/C–H-activation reactions for the preparation of condensed aromatic systems.9 Herein we report even more productive domino Sonogashira/double carbopalladation/C–H activation reactions that convert aryl iodides 1 and dialkyne 2 into polycyclic aromatic hydrocarbons 3 in up to 97% yield (Scheme 1).
RESULTS AND DISCUSSION
For the optimization of the reaction conditions, we investigated the domino reaction of 1a and 2 as coupling partners in the presence of a combination of Pd(OAc)2 and PPh3 in a ratio of 1:5 as catalytic system and (nBu)4NOAc as base in DMF at 100 °C. A catalyst loading of 10 mol% Pd(OAc)2 furnished the domino product 3a in 81% yield as the best result for this system (Table 1, Entry 1). Reduction of the catalyst loading to 5 mol% of Pd(OAc)2 led to 3a in 67% yield while 1 mol% of Pd(OAc)2 furnished 3a in 66% yield (Entries 2 and 3). Moreover, a higher catalyst loading of 20 mol% of Pd(OAc)2 was also not advantageous, since the yield of the domino reaction dropped to 60% (Entry 4). In order to further optimize the reaction conditions, we tested several ligands with a 20 mol% loading of Pd(OAc)2. Electron rich PCy3 led to 3a in 53% yield (Entry 5), while the electron rich and bulky SPhos ligand as well as the ionic species [PtBu4][BF4] gave 49% yield (Entries 6 and 7). The use of bidentate dppe furnished 3a in only 16% (Entry 8). Thus, PPh3 showed the best results and was used in the further investigations employing different substrates.
In the following, we extended the scope of the fourfold domino reaction using differently substituted substrates 1b-f. The corresponding polycyclic aromatic hydrocarbons 3b-f were obtained highly selectively and in good to excellent yield ranging from 54 – 97% (Scheme 2). Moreover, the results show that functional groups having electron-accepting and electron-donating properties are tolerated. Thus, the already mentioned naphthyl iodide 1a containing a 4-nitro-phenoxy group in the ortho position gave 3a in 81% yield and the 2-trifluorophenyl iodide 1b led to 3b in 97% yield. Excellent yields of 90% and 80%, respectively, were also obtained with the electron neutral 1c and the phenyl iodide 1d containing a 2-methoxyphenoxy group in the ortho position. Interesting examples are the phenyl iodides 1e and 1f. Compound 1e containing four methyl groups is sterically highly hindered and in 1f there is a competing vinyl group which might undergo an intermolecular Mizoroki-Heck reaction after the primary oxidative addition. However, the Sonogashira reaction of 1f with 2 seems to be much faster than the also possible Mizoroki-Heck reaction. Anyhow, in both cases the yields with 64% and 54% were slightly lower.
The domino Sonogashira/double carbopalladation/C–H activation process as described for the reaction of aryl iodide 1a and dialkyne 2 starts with a Sonogashira reaction that couples 1a with 2 to form intermediate 4 (Scheme 3). After an oxidative addition at the C–Br bond in 4, a first carbopalladation takes place at one of the two triple bonds in 4 to provide vinyl-palladium species 5 which contains a newly generated six-membered heterocycle. The vinyl-palladium species then undergoes a carbopalladation at the second triple bond furnishing dialkene 6 containing another vinyl-palladium species and a five-membered ring which is built up during the carbopalladation process. Finally, a C–H-activation reaction transforms 6 into the desired polycyclic aromatic hydrocarbon 3a whereupon the two newly formed double bonds become part of the aromatic naphthalene system in 3a. This fact might act as a driving force and could explain the smooth and high yielding course of the domino process.
The syntheses of the substrates 1 and 2 for the domino reactions either followed literature known protocols8–11 or the necessary compounds were commercially available as 1b, c and e.
Building block 1a was prepared through an iodination of naphthol 7 and a subsequent nucleophilic aromatic substitution coupling naphthol 8 with 4-fluoronitrobenzene 9 to give 1a in 78% yield (Scheme 4).
The sequence leading to building block 2 started with a nucleophilic aromatic substitution of phenol 10 and 2-fluoronitrobenzene (11), which was followed by a reduction of the nitro group and a Sandmeyer reaction to give the aryl iodide 12. Then, compound 12 was converted into the dialkyne 2 through a Sonogashira reaction using 1,6-heptadiyne as coupling partner (Scheme 5).
Compounds 3b-f exhibit an interesting fluorescence activity and we investigated molecule 3c exemplarily by fluorescence spectrometry (Figure 1).12 Irradiation at a wavelength of 352 nm caused an emission of blue light with a maximum at a wavelength of 436 nm. The fluorescence properties of 3b-f could be useful for the application of compounds of type 3 as fluorescence dyes or chemical sensors.
In conclusion, we have developed a fourfold domino process with a C–H-activation reaction as the final step to transform simple precursors highly efficiently into polycyclic aromatic hydrocarbons with pronounced fluorescence activity.
EXPERIMENTAL
General method for the domino reaction: 9-[2-(4-Nitrophenoxy)naphthalene-1-yl]-11,12-dihydro-10H-indeno[6,5,4-kl]xanthene (3a):
A mixture of 1a (73.6 mg, 189 µmol, 1.00 equiv.), 2 (67.1 mg, 198 µmol, 1.05 equiv.), Pd(OAc)2 (4.22 mg, 18.8 µmol, 0.10 equiv.), PPh3 (24.7 mg, 94.0 µmol, 0.50 equiv.) and (nBu)4NOAc (341 mg, 1.13 mmol, 6.00 equiv.) in degassed DMF (5 mL) was stirred at 100 °C for 21 h. The reaction mixture was filtered through SiO2, flushed with EtOAc and the solvent of the filtrate was removed in vacuo. Column chromatography (SiO2, n-pentane/EtOAc 20:1) yielded 3a as a yellow solid (79.9 mg, 153 µmol, 81%).
Rf = 0.40 (n-pentane/EtOAc, 20:1); UV/Vis (MeCN) nm (lg ε) = 193 (4.9352), 223 (4.3783), 256 (3.8842), 286 (3.6870), 350 (3.4956), 367 (3.4858); IR (ATR) cm-1 = 1580, 1509, 1485, 1463, 1442, 1338, 1307, 1279, 1240, 1166, 1128, 1110, 1066, 1045, 1028, 1012, 958, 860, 852, 836, 821, 808, 768, 761, 750, 734, 703, 688, 667, 645, 629, 618, 530, 522, 514; 1H-NMR (600 MHz, C6D6) δ: 1.58–1.70 (m, 2H, 6’’-H2), 2.33 (ddd, J = 16.2 and 8.4 and 6.2 Hz, 1H), 2.50 (dt, J = 15.9 and 7.9 Hz, 1H), 2.87 (dt, J = 15.6 and 7.5 Hz, 1H, 5’’-Ha), 2.94 (ddd, J = 15.8 and 8.2 and 5.9 Hz, 1H, 5’’-Hb), 6.37 (d, J = 9.2 Hz, 2H, 2’-H, 6’-H), 6.77 (dd, J = 7.9 and 1.6 Hz, 1H, 10’’-H), 6.84–6.92 (m, 3H, 3’’-H, 8’’-H, 9’’-H), 6.97 (ddd, J = 8.3 and 7.3 and 1.4 Hz, 1H, 2’’-H), 7.03–7.09 (m, 2H, 3-H, 1’’-H), 7.14 (mC, 1H, 7-H), 7.26 (ddd, J = 8.1 and 6.8 and 1.2 Hz, 1H, 6-H), 7.43 (dd, J = 8.5 and 1.1 Hz, 1H, 8-H), 7.59 (d, J = 9.2 Hz, 2H, 3’-H, 5’-H), 7.67 (d, J = 8.9 Hz, 1H, 4-H), 7.71 (d, J = 8.4 Hz, 1H, 4’’-H), 7.73 (d, J = 8.4 Hz, 1H, 5-H); 13C-NMR (126 MHz, C6D6) δ: 25.5 (C-6’’), 32.2 (C-7’’), 35.3 (C-5’’), 108.1 (C-8’’), 117.0 (C-2’, C-6’), 117.2 (C-1’’), 118.2 (C-10’’), 120.7 (C-3), 122.4, 122.6 (C-4’’a), 123.1 (C-3’’), 123.3 (C-4’’b), 125.5 (C-3’, C-5’), 125.7, 126.1 (C-6, C-8), 127.0, 127.1, 127.6 (C-7, C-4’’, C-9’’), 127.9 (C-1), 128.3, 128.6 (C-5), 129.6 (C-2’’), 130.2 (C-4), 132.0 (C-4a), 133.9 (C-8a, C-4’’c/C-7’’a), 134.1 (C-7’’d), 143.0 (C-1’), 146.4 (C-4’’c/C-7’’a), 150.2 (C-2), 151.7 (C-10a), 153.4 (C-10’’b), 162.7 (C-1’’) (Further signals could not be assigned); MS (ESI): 544.2 (41) (M+Na+); HRMS (ESI): calcd for C35H23NO4+Na+: 544.1519. Found: 544.1505.
ACKNOWLEDGEMENTS
We thank the Bundesland Niedersachsen, the Deutsche Forschungsgemeinschaft and the Volkswagen-Stiftung for their generous support.
References
1. L. F. Tietze (Ed), 'Domino Reactions', Wiley-VCH, Weinheim, 2014; CrossRef L. F. Tietze, S.-C. Düfert, and J. Hierold, 'Domino Reactions in the Total Synthesis of Natural Products' in "Domino Reactions: Concepts for Efficient Organic Synthesis", ed. by L. F. Tietze, Wiley-VCH, Weinheim, 2014, pp. 523–578; CrossRef L. G. Voskressensky, A. A. Festa, and A. V. Varlamov, Tetrahedron, 2014, 70, 551; CrossRef C. M. R. Chandra, I. Atodiresei, and M. Rueping, Chem. Rev., 2014, 114, 2390; CrossRef J. Muzart, Tetrahedron, 2013, 69, 6735; CrossRef L. F. Tietze and A. Düfert in 'Modern Tools for the Synthesis of Complex Bioactive Molecules', Wiley, Hoboken, NJ, 2012; H. Pellissier, Adv. Synth. Catal., 2012, 48, 5889; L. F. Tietze and A. Düfert in 'Catalytic Asymmetric Conjugate Reactions', Wiley-VCH, Weinheim, 2010, pp. 321-350; CrossRef L. F. Tietze, G. Brasche, and K. M. Gericke in 'Domino Reactions in Organic Synthesis', WILEY-VCH, Weinheim, 2006; CrossRef L. F. Tietze, Chem. Rev., 1996, 96, 115; CrossRef L. F. Tietze, S. Dietz, N. Böhnke, M. A. Düfert, I. Objartel, and D. Stalke, Eur. J. Org. Chem., 2011, 6574. CrossRef
2. L. F. Tietze, S. Dietz, N. Böhnke, M. A. Düfert, I. Objartel, and D. Stalke. Eur. J. Org. Chem., 2011, 6574. CrossRef
3. J. Wölfling, E. Frank, G. Schneider, and L. F. Tietze, Eur. J. Org. Chem., 2004, 90. CrossRef
4. L. F. Tietze, N. Tölle, D. Kratzert, and D. Stalke, Org. Lett., 2009, 11, 5230. CrossRef
5. L. F. Tietze, T. Kinzel, and C. Brazel, Acc. Chem. Res., 2009, 42, 367. CrossRef
6. L. F. Tietze, S. Jackenkroll, J. Hierold, L. Ma, and B. Waldecker, Chem. Eur. J., 2014, 20, 8628; CrossRef M. Sickert, H. Weinstabl, B. Peters, X. Hou, and M. Lautens, Angew. Chem. Int. Ed., 2014, 53, 5147; E. Elboray, M. F. Aly, H. H. Abbas-Temirek, G. H. Churchill, and R. Grigg, Tetrahedron, 2014, 70, 110; CrossRef C. Zheng, J. J. Chen, and R. Fan, Org. Lett., 2014, 16, 816; CrossRef S. R. Walker, M. L. Czyz, and J. C. Morris, Org. Lett., 2014, 16, 708; CrossRef L. F. Tietze, S.-C. Duefert, J. Clerc, M. Bischoff, C. Maaß, and D. Stalke, Angew. Chem. Int. Ed., 2013, 52, 3191; CrossRef L. F. Tietze, S. Jackenkroll, C. Raith, D. A. Spiegl, J. R. Reiner, and M. C. Ochoa Campos, Chem. Eur. J., 2013, 19, 4876; CrossRef L. F. Tietze, T. Hungerland, J. Ammermann, C. Eichhorst, S. O. Reichmann, and D. Stalke, J. Indian Chem. Soc., 2013, 90, 1537; Q. Liu, L. Wu, H. Jiao, X. Fang, R. Jackstell, and M. Beller, Angew. Chem. Int. Ed., 2013, 52, 8064; CrossRef R. Y. Nimje, M. V. Leskinen, and P. M. Pihko, Angew. Chem. Int. Ed., 2013, 52, 4818; E. Kaneko, Y. Matsumoto, and K. Kamikawa, Chem. Eur. J., 2013, 19, 11837; N. M. Groome, E. E. Elboray, M. W. Imman, H. Ali Dondas, R. M. Phillips, C. Kilner, and R. Grigg, Chem. Eur. J., 2013, 19, 2180; CrossRef M. Leibeling and D. B. Werz, Chem. Eur. J., 2012, 18, 6138; CrossRef M. Leibeling, M. Pawliczek, D. Kratzert, D. Stalke, and D. B. Werz, Org. Lett., 2012, 14, 346; CrossRef X. Jia, D. A. Petrone, and M. Lautens, Angew. Chem. Int. Ed., 2012, 51, 9870; CrossRef H. Liu, M. El-Salfiti, and M. Lautens, Angew. Chem. Int. Ed., 2012, 51, 9846; CrossRef L. F. Tietze, T. Hungerland, M. A. Düfert, I. Objartel, and D. Stalke, Chem. Eur. J., 2012, 18, 3286; CrossRef L. F. Tietze, T. Hungerland, C. Depken, C.Maaß, and D. Stalke, Synlett, 2012, 2516; CrossRef L. F. Tietze, M. A. Düfert, T. Hungerland, K. Oum, and T. Lenzer, Chem. Eur. J., 2011, 17, 8452; CrossRef J. E. Rixson, T. Chaloner, C. H. Heath, L. F. Tietze, and S. G. Stewart, Eur. J. Org. Chem., 2011, 544; L. F. Tietze, A. Düfert, F. Lotz, L. Sölter, K. Oum, T. Lenzer, T. Beck, and R. Herbst-Irmer, J. Am. Chem. Soc., 2009, 131, 17879; CrossRef L. F. Tietze, D. A. Spiegl, F. Stecker, J. Major, C. Raith, and C. Grosse, Chem. Eur. J., 2008, 14, 8956; CrossRef L. F. Tietze, K. M. Sommer, J. Zinngrebe, and F. Stecker, Angew. Chem., Int. Ed., 2005, 44, 257. CrossRef
7. K. Gao and N. Yoshikai, Acc. Chem. Res., 2014, 47, 1208; CrossRef A. R. Kapdi, Dalton Trans., 2014, 43, 3021; CrossRef E. M. Ferreira, Nat. Chem., 2014, 6, 94; CrossRef N. Yoshikai and Y. Wei, Asian J. Org. Chem., 2013, 2, 466; J. Wencel-Delord and F. Glorius, Nat. Chem., 2013, 5, 369; CrossRef M. Garcia-Melchior, A. A. C. Braga, A. Lledos, G. Ujaque, and F. Maseras, Acc. Chem. Res., 2013, 46, 2626; CrossRef J. J. Mousseau and A. B. Charette, Acc. Chem. Res., 2013, 46, 412; CrossRef L. Ackermann, A. R. Kapdi, H. Potukuchi, and S. I. Kozhushkov, in 'Handbook of Green Chemistry', Wiley-VCH, Weinheim 2012, p. 259; A. Facchetti, L. Vaccaro, and A. Marrocchi, Angew. Chem. Int. Ed., 2012, 51, 3520; CrossRef S. R. Neufeldt and M. S. Sanford, Acc. Chem. Res., 2012, 45, 936. CrossRef
8. L. F. Tietze, T. Hungerland, C. Eichhorst, A. Düfert, C. Maaß, and D. Stalke, Angew. Chem. Int. Ed., 2013, 52, 3668. CrossRef
9. L. F. Tietze, C. Eichhorst, T. Hungerland, and M. Steinert, Chem. Eur. J., 2014, 20, early view.
10. J. A. De la Fuente, J. Med. Chem., 2003, 46, 5208; CrossRef F. Wen, H. Zhang, Z. Yu, H. Jin, Q. Yang, and T. Hou, Pestic. Biochem. Physiol., 2010, 98, 248. CrossRef
11. D. Sun, P. Lai, W. Xie, J. Deng, and Y. Jiang, Synth. Commun., 2007, 37, 2989; CrossRef M. R. Acheson and G. C. M. Lee, J. Chem. Soc., Perkin Trans. 1, 1987, 11, 2321. CrossRef
12. Fluorescence spectrum: The spectrum was recorded on a fluorimeter FP 6500 by JASCO. Light source: 150 W, Xe lamp; photometric system: monochromatic light; monochromator: signal-to-noise ratio (Raman-band of water, 350 nm excitation wavelength, 2 sec response time, 5 nm bandwidth) of 200:1 (excitation and emission monochromator), measuring wavelength range: zero order, 220–750 nm; resolution: 1 nm (excitation and emission), wavelength accuracy: +/- 1.5 nm (excitation and emission); wavelength reproducibility: +/-0.3 nm (excitation and emission); detector: photomultiplier tube (excitation and emission).