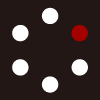
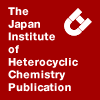
HETEROCYCLES
An International Journal for Reviews and Communications in Heterocyclic ChemistryWeb Edition ISSN: 1881-0942
Published online by The Japan Institute of Heterocyclic Chemistry
e-Journal
Full Text HTML
Received, 29th June, 2014, Accepted, 4th August, 2014, Published online, 6th August, 2014.
DOI: 10.3987/COM-14-S(K)55
■ An Access to the 13-Membered Cyclophane Substructure in GKK1032As: An Intramolecular 1,4-Addition Approach
Satoka Nagai, Yuka Yamagishi, Yuta Shimizu, Ken-ichi Takao, and Kin-ichi Tadano*
Department of Applied Chemistry, Emeritus, Keio University, 3-14-1, Hiyoshi, Kohoku-ku, Yokohama, Japan
Abstract
The construction of the 13-membered para-cyclophane substructure in GKK1032As, a member of novel pyrrolidinone-containing bioactive natural products, has been explored. An efficient approach for this synthetically formidable object was found, which relied on an intramolecular 1,4-addition between a nitromethylene group and a vinyl ketone moiety both incorporated as side chains into the 6/5/6-tricyclic (the A/B/C-ring system) of the GKK1032s.In recent years, some aryl- and pyrrolidinone-containing polycyclic antibiotics were isolated from microorganisms. GKK1032A1(1), A2(2) and B(3) (Figure 1) were isolated in 2001 by the Kyowa Hakko research group from a strain of Penicillium sp. GKK1032.1 GKK1032A2 (2) was also isolated by the research group at the Kitasato Institute, designated as FO-7711CD6, from the culture broth of a Penicillium sp.2 These novel compounds exhibit remarkable antibacterial activity against such as Bacillus subtilis No. 10707, including drug-resistant strain, as well as antitumor activity against epithelioid cell HeLaS3.1a The relative stereochemistries of these antibiotics were determined on the basis of extensive NMR analysis and finally established by single-crystal X-ray crystallography for 3. The structures of GKK1032s consist of a tricarbocyclic ring system (a 6/5/6-membered decahydrofluorene skeleton) referred to as A/B/C-ring with nine stereogenic carbons, including two quaternary ones, as the common part of 1-3. An additional structural characteristic of 1 and 2 is a 13-membered macrocyclic ether (or 12-membered ether for 3) consisting of a para-substituted phenolic ether (a paracyclophane structure) attaching to a 3-acyl-5-hydroxypyrrolidin-2-one functionality. The absolute configurations for 1-3 remain undetermined. In 2002, He et al. at the Wyeth-Ayerst Research reported the isolation and characterization of pyrrocidine A (4) and B (5) from the fermentation broth of a filamentous fungus LL-Cyan426, which showed similar biological activity to those of 1-3.3 A structural difference between GKK1032s and pyrrocidines is the stereochemistry of the A/B ring juncture, i.e., the trans-relationship for 1-3 or the cis-relationship for 4 and 5. Additionally, a methyl group is missing on the C3 carbon in the A-ring of 4 and 5, compared to 1-3. In 2005, Isaka et al. reported the isolation of hirsutellones A-E.4 The structures of the two major hirsutellones, A (6) and B (7), are depicted in Figure 1. These natural products were isolated from the insect pathogenic fungus Hirsutella nivea BCC 2594 and showed significant growth inhibitory activity against Mycobacterium tuberculosis H37Ra. Attracted by these unprecedented structures and prominent biological activities, a number of research groups have devoted their efforts to the total synthesis of these natural products. Until today, the groups of Kuwajima,5 Inoue/Katoh,6 and Uchiro7 have reported their synthetic results on the GKK1032s. As concerns the pyrrocidine synthesis, the groups of Kobayashi8 and Nay9 have reported their synthetic approaches. Sorensen et al. first reported the rapid and asymmetric synthesis of the upper decahydrofluorene core of the hirsutellones.10 Nicolaou et al. reported the first total synthesis of natural (+)-hirsutellone B (7), which established the absolute stereochemistry of the natural product.11 Then Uchiro et al. reported their total synthesis of (+)-hirsutellone B in 2011.12 Two formal syntheses13,14 of (+)-hirsutellones and several synthetic approaches toward hirsutellones15,16 have been reported to date. We have been involved in the total synthesis of the GKK1032s in this decade, and our asymmetric synthetic approach toward the fully functionalized A/B/C-ring (the decahydrofluorene skeleton) in both enantiomeric forms has been recently reported.17 In this report, we disclose our synthetic efforts on the construction of the 13-membered para-cylophane moiety of 1 and 2, featuring (1) a copper-mediated Ullmann ether synthesis for introduction of the aryl alkyl ether moiety and (2) an intramolecular 1,4-addition reaction for construction of the 13-membered para-cyclophane structure.18
Our stereoselective synthesis of the decahydrofluorene moiety (the A/B/C-ring system) of 1-3 is outlined in Schemes 1 and 2.17 The endo-adduct (10), produced in the Diels-Alder cycloaddition of (E)-2-methyl- 1,3-pentadiene (8) and maleic anhydride (9) as an exclusive product, was converted efficiently into bicyclic γ-acetoxy-γ-lactone (11). Thus obtained racemic C-ring equivalent (11) was optically resolved by Lipase AK-mediated kinetic hydrolysis. As a result, the kinetically hydrolyzed product [(+)-12] and unreacted γ-acetyl-γ-lactone [(+)-13] were isolated both in excellent yield and high enantiomeric excess (ee). From (+)-13, (–)-12 was also obtained. The absolute stereochemistries of both enantiomers, i.e., (+)- and (–)-12, were unambiguously established through independent synthetic efforts.
The highly enantioenriched bicyclic γ-hydroxy-γ-lactone [(+)-12] was converted efficiently into the substrate (14) of intramolecular Diels-Alder (IMDA) reaction. The thermal IMDA reaction of 14 in the presence of silica gel provided the desired adduct (15) as a sole product with complete endo- and π-facial selectivity. The configuration at the carbon bearing a hydroxyl group (B-ring) in the adduct (15) was inverted using an oxidation/reduction strategy, providing 16 stereoselectively.
The construction of the 13-membered para-cylophane moiety of 1 and 2 was started from diol (16)19 (Scheme 3). Selective protection of the primary hydroxyl group in diol (16) as an SEM [(2-trimethylsilyl)ethoxymethyl] ether provided 17.20 Similar to Buchwald’s procedure,21 the secondary hydroxy group in 17 was reacted with 1,4-diiodobenzene in the presence of copper(I) iodide, 1,10-phenanthroline, and cesium carbonate in toluene at 160 °C (in a sealed tube) for 43 h. The desired p-iodophenyl ether (18)22 was obtained in 48% yield, and 17 was recovered in 48% yield.23 Replacement of the iodo group in the phenyl ether (18) by a cyano group using copper cyanide at 160 °C (Rosenmund–von Braun reaction) smoothly provided benzonitrile (19). The cyano group in 19 was converted into benzaldehyde (20) by treating with diisobutylaluminum hydride (DIBAL-H) at –78 °C. The thus efficiently obtained benzaldehyde (20) was subjected to a nitroaldol (Henry) reaction with nitromethane using sodium methoxide as a base, providing β-nitroalcohol (21) as a 5:4 diastereomeric mixture. This mixture (21) was treated with a mixed solution of acetic anhydride and pyridine (1:1). As a result, the desired trans-β-nitrostyrene (22)24 was obtained through β-elimination of initially formed β-nitro acetates. The nitrostyrene (22) was then reduced with sodium borohydride, providing p-β-nitroethylphenyl ether (23).
Next, introduction of a vinyl ketone moiety, as an acceptor of the 1,4-addition, into the p-β- nitroethylphenyl derivative (23) was explored (Scheme 4). The SEM group in 23 was removed by acid treatment, generating primary alcohol (24). Oxidation of 24 with Dess–Martin periodinane to provide aldehyde (25). The Grignard reaction of 25 with vinylmagnesium bromide smoothly provided the vinyl adduct (26) as an approximately 3:1 diastereomeric mixture. This mixture was oxidized with Dess–Martion periodinane to provide the vinyl ketone (27).25 When 27 was treated with an equimolar of DBU in benzene at room temperature for 1 h, two intramolecular 1,4-addition products (28) were obtained in 26% and 28% yields. One of the 1,4-addition products was isolated as a single diastereomer in the keto form;26 otherwise, the other products likely to be an inseparable mixture of keto and enol forms of another diastereomeric 1,4-adduct. At the present time, we could not determine conclusively the configurations of the newly introduced stereogenic centers in both 1,4-adducts (28).27
In conclusion, we have developed an access to the construction of the 13-membered para-cylophane moiety of GKK1032s from one enantiomer of the A/B/C-ring equivalent, which in turn has stereoselectively synthesized in virtually enantiopure form. Our approach to this issue was characterized by (1) a copper-mediated Ullmann ether synthesis for introduction of the aryl alkyl ether moiety in B-ring and (2) an intramolecular 1,4-addition reaction between a β-nitroethylphenyl group and a vinyl ketone embedded in the substrate, which was derived from the Ullmann reaction product, for constructing the 13-membered para-cyclophane substructure.
ACKNOWLEDGEMENTS
This work was supported in part by Grants-in-Aid for Scientific Research on Priority Areas (A), “Creation of Biologically Functional Molecules (17035075),” from the Ministry of Education, Culture, Sports, Science, and Technology of Japan. We thank Dr. Keisuke Yoshida for his technical assistance.
References
1. (a) F Koizumi, A. Hasegawa, K. Ando, T. Ogawa, and M. Hara, Jpn. Kokai Tokkyo Koho, JP 2001-247574A 20010911, 2001 (Chem. Abstr., 2001, 135, 209979); (b) A. Hasegawa, F. Koizumi, Y. Takahashi, K. Ando, T. Ogawa, M. Hara, and M. Yoshida, The 43rd Symposium on the Chemistry of Natural Products, Symposium Papers; Osaka: Japan 2001.
2. S. Omura, H. Komiyama, M. Hayashi, R. Masuma, and A. Fukami, Jpn. Kokai Tokkyo Koho, JP 2002-255969 A 20020911, 2002 (Chem Abstr., 2002, 137, 231476).
3. H. He, H. Y. Yang, R. Bigelis, E. H. Solum, M. Greenstein, and G. T. Carter, Tetrahedron Lett., 2002, 43, 1633. CrossRef
4. M. Isaka, N. Rugseree, P. Maithip, P. Kongsaeree, S. Prabpai, and Y. Thebtaranonth, Tetrahedron, 2005, 61, 5577; CrossRef M. Isaka, P. Kittakoop, K. Kirtikara, N. L. Hywel-Jones, and Y. Thebtaranonth, Acc. Chem. Res., 2005, 38, 813. CrossRef
5. N. Arai, H. Ui, S. Omura, and I. Kuwajima, Synlett, 2005, 1691.
6. M. Asano, M. Inoue, and T. Katoh, Synlett, 2005, 1539; M. Asano, M. Inoue, and T. Katoh, Synlett, 2005, 2599; M. Asano, M. Inoue, K. Watanabe, H. Abe, and T. Katoh, J. Org. Chem., 2006, 71, 6942. CrossRef
7. H. Uchiro, R. Kato, Y. Sakuma, Y. Takagi, Y. Arai, and D. Hasegawa, Tetrahedron Lett., 2011, 52, 6242. CrossRef
8. R. Tanaka, K. Ohishi, N. Takanashi, T. Nagano, H. Suizu, T. Suzuki, and S. Kobayashi, Org. Lett., 2012, 14, 4886. CrossRef
9. H. Abdelkafi, L. Evanno, A. Deville, L. Dubost, A. Chiaroni, and B. Nay, Eur. J. Org. Chem., 2011, 2789. CrossRef
10. S. D. Tilley, K. P. Reber, and E. J. Sorensen, Org. Lett., 2009, 11, 701; CrossRef K. P. Reber, S. D. Tilley, and E. J. Sorensen, Chem. Soc. Rev., 2009, 38, 3022. CrossRef
11. K. C. Nicolaou, D. Sarlah, T. R. Wu, and W. Zhan, Angew. Chem. Int. Ed., 2009, 48, 6870; CrossRef K. C. Nicolaou, Y.-P. Sun, D. Sarlah, W. Zhan, and T. R. Wu, Org. Lett., 2011, 13, 5708. CrossRef
12. H. Uchiro, R. Kato, Y. Arai, M. Hasegawa, and Y. Kobayakawa, Org. Lett., 2011, 13, 6268. CrossRef
13. K. P. Reber, S. D. Tilley, C. A. Carson, and E. J. Sorensen, J. Org. Chem., 2013, 78, 9584. CrossRef
14. N. Riache, I. Ndoye, X.-W. Li, and B. Nay, Synlett, 2011, 2685; X.-W. Li, A. Ear, L. Roger, N. Riache, A. Deville, and B. Nay, Chem. Eur. J., 2013, 19, 16389; CrossRef X.-W. Li, A. Ear, and B. Nay, Nat. Prod. Rep., 2013, 30, 765. CrossRef
15. M. Huang, C. Huang, and B. Liu, Tetrahedron Lett., 2009, 50, 2797; CrossRef M. Huang, L. Song, and B. Li, Org. Lett., 2010, 12, 2504. CrossRef
16. G. T. Halvorsen and W. R. Roush, Tetrahedron Lett., 2011, 52, 2072. CrossRef
17. K. Tadano, Chem. Rec., Early View; DOI: 10.1002/tcr.201402008. The synthesis of enantio-enriched (+)-12 and (–)-12, the conversion of (+)-12 into 14, the IMDA reaction of 14, and the conversion of the IMDA adduct (15) into 16 were described in detail in this paper. CrossRef
18. We initially presented our works described in this communication at the 29th Seminar on Synthetic Organic Chemistry, Y. Yamagishi, S. Nagai, Y. Shimizu, K. Takao, and K. Tadano; Shizuoka, September 6, 2012. Abstract paper, P-81.
19. We selected arbitrarily the enantiomer (16) as the starting compound of the present work owing to the absolute stereochemistries of GKK1032s are still undetermined.
20. Compounds (17-20), (22-25), (27) and (28) were fully characterized by spectroscopic means (1H and 13C NMR, IR) and gave satisfactory HRMS. Diastereomeric mixtures (21) and (26) were characterized their structures based on 1H NMR analysis.
21. M. Wolter, G. Nordmann, G. E. Job, and S. L. Buchwald, Org. Lett., 2002, 4, 973. CrossRef
22. Compound (18) as a colorless oil: TLC Rf 0.66 (EtOAc/hexane, 1:8); [α]20D –66.3 (c 1.03, CHCl3); IR (neat) 3018, 2952, 2926, 1582 cm-1; 1H NMR (500 MHz, CDCl3) δ 0.02 (s, 9H), 0.53 (ddd, 1H, J = 12.0, 12.0, 12.0 Hz), 0.73-0.77 (m, 1H), 0.75 (d, 3H, J = 6.0 Hz), 0.85 (d, 3H, J = 6.3 Hz), 0.88-0.92 (m, 2H), 0.98-1.01 (m, 1H), 1.02 (s, 3H), 1.12 (s, 3H), 1.60-1.63 (m, 1H), 1.69-1.80 (m, 4H),1.77 (s, 3H), 1.91 (dd, 1H, J = 3.2, 12.0 Hz), 2.22 (ddd, 1H, J = 4.1, 11.5, 11.5 Hz), 2.73 (dd, 1H, J = 8.1, 10.0 Hz), 3.42-3.48 (m, 2H), 3.59 (m, 1H), 4.16 (d, 1H, J = 6.6 Hz), 4.47 (d, 1H, J = 6.6 Hz), 4.75 (s, 1H), 4.92-4.95 (m, 1H), 4.94 (dd, 1H , J = 1.5, 17.2 Hz), 4.98 (dd, 1H, J = 1.5, 10.4 Hz), 5.61 (dd, 1H, J = 10.6, 17.2 Hz), 6.71 (d, 2H, J = 8.9 Hz), 7.44 (d, 2H, J = 8.9 Hz); 13C NMR (68 MHz) δ –1.02, 15.6, 18.4, 20.6, 22.0, 23.0, 26.0, 27.2, 28.0, 42.7, 44.8, 45.8, 48.9, 49.2, 51.3, 59.0, 62.0, 65.1, 67.4, 79.2, 82.7, 94.5, 115.0, 120.0, 132.1, 135.8, 138.0, 142.7, 159.0; HRMS (EI) calcd for C33H51O3SiI (M+ ) m/z 650.2653, found 650.2646.
23. As unreacted starting compound (17) was efficiently recovered, we did not explore other reaction conditions for this aryl ether formation.
24. Compound (22) as a pale yellow oil: TLC Rf 0.74 (EtOAc/hexane, 8:1); [α]20D –77.5 (c 1.13, CHCl3); IR (neat) 3020, 2953, 2927, 1600 cm-1; 1H NMR (500 MHz, CDCl3) δ 0.02 (s, 9H), 0.56 (ddd, 1H, J = 12.3, 12.3, 12.3 Hz), 0.72 (d, 3H, J = 6.0 Hz), 0.79 (dd, 1H, J = 12.3, 12.3 Hz), 0.86-0.97 (m, 2H), 0.89 (d, 3H, J = 6.3 Hz), 1.04-1.08 (m, 1H), 1.06 (s, 3H), 1.15 (s, 3H), 1.68-1.77 (m, 5H), 1.81 (s, 3H), 1.95 (dd, 1H, J = 3.5, 12.3 Hz), 2.29 (ddd, 1H, J = 4.3, 11.8, 11.8 Hz), 2.86 (dd, 1H, J = 8.3, 10.2 Hz), 3.47-3.51 (m, 1H), 3.57 (dd, 1H, J = 2.6, 10.2 Hz), 3.56-3.65 (m, 1H), 4.21 (d, 1H, J = 6.6 Hz), 4.53 (d, 1H, J = 6.6 Hz), 4.79 (s, 1H), 4.97 (dd, 1H, J = 1.5, 17.2 Hz), 5.00 (dd, 1H, J = 1.5, 10.6 Hz), 5.16 (dd, 1H, J = 4.3, 6.6 Hz), 5.64 (dd, 1H, J = 10.6, 17.2 Hz), 7.02 (d, 2H, J = 8.9 Hz), 7.43 (d, 2H, J = 8.9 Hz), 7.50 (d, 1H, J = 13.5 Hz), 7.94 (d, 1H, J = 13.5 Hz); 13C NMR (68 MHz) δ –1.41, 15.4, 18.2, 20.6, 21.8, 22.8, 25.7, 27.0, 27.9, 42.6, 44.5, 45.7, 48.7, 49.0, 51.2, 58.6, 61.9, 65.1, 67.3, 78.9, 94.3, 114.9, 117.1, 122.0, 130.9, 132.0, 134.7, 135.5, 139.2, 142.4, 162.7; HRMS (EI) calcd for C35H53NO5Si (M+) m/z 595.3693, found 595.3693.
25. Compound (27) as a colorless oil: TLC Rf 0.42 (EtOAc/hexane, 1:4); [α]20D –124.0 (c 1.12, CHCl3); IR (neat) 3020, 2952, 2927, 1676 cm-1; 1H NMR (500 MHz, CDCl3) δ 0.46-0.54 (m, 1H), 0.51 (d, 3H, J = 6.0 Hz), 0.77 (dd, 1H, J = 12.3, 12.3 Hz), 0.88 (d, 3H, J = 6.3 Hz), 0.92 (dd, 1H, J = 6.7, 10.9 Hz), 1.10 (s, 3H), 1.15 (s, 3H), 1.65-1.80 (m, 4H), 1.84 (s, 3H), 1.96 (dd, 1H, J = 3.2, 12.3 Hz), 2.73 (ddd, 1H, J = 4.6, 12.0, 12.0 Hz), 2.83 (d, 1H, J = 12.0 Hz), 3.20 (t, 2H, J = 7.3 Hz), 4.47 (dd, 1H, J = 4.6, 6.7 Hz), 4.52 (t, 2H, J = 7.3 Hz), 4.83 (s, 1H), 4.99 (dd, 1H, J = 1.4, 17.2 Hz), 5.05 (dd, 1H, J = 1.4, 10.6 Hz), 5.23 (dd, 1H, J = 1.5, 10.3 Hz), 5.69 (dd, 1H, J = 10.6, 17.2 Hz), 5.98 (dd, 1H, J = 1.5, 17.2 Hz), 6.36 (dd, 1H, J = 10.3, 17.2 Hz), 6.65 (d, 2H, J = 8.6 Hz), 6.96 (d, 2H, J = 8.6 Hz); 13C NMR (68 MHz) δ 15.5, 20.7, 21.8, 22.7, 26.9, 27.1, 27.7, 32.8, 42.4, 45.0, 45.7, 48.8, 48.9, 58.3, 62.6, 62.7, 76.7, 78.1, 114.9, 116.2, 126.9, 129.0. 131.0, 135.6, 136.1, 143.3, 158.6, 200.8; HRMS (EI) calcd for C31H41NO4 (M+) m/z 491.3036, found 491.3033.
26. A single stereoisomer (28) obtained as colorless solids: TLC Rf 0.29 (EtOAc/hexane, 1:4); IR (neat) 3020, 2952, 2928, 1712, 1687, 1580 cm-1, 1H NMR (500 MHz, CDCl3) δ 0.48 (d, 3H, J = 6.1 Hz), 0.50-0.60 (m, 1H), 0.74 (dd, 1H, J = 11.8, 11.8 Hz), 0.85-0.89 (m, 1H), 0.86 (d, 3H, J = 6.2 Hz), 1.10 (s, 3H), 1.21 (s, 3H), 1.60-1.72 (m, 4H), 1.81 (s, 3H), 1.90-1.98 (m, 1H), 2.08-2.20 (m, 1H), 2.30-2.44 (m, 2H), 2.65-2.77 (m, 3H), 3.04 (dd, 1H, J = 9.5, 14.6 Hz), 4.46 (dd, 1H, J = 3.5, 6.1 Hz), 4.60-4.65 (m, 1H), 4.75 (s, 1H), 4.96 (dd, 1H, J = 1.2, 17.2 Hz), 4.99 (dd, 1H, J = 1.2, 10.3 Hz), 5.73 (dd, 1H, J = 10.3, 17.2 Hz), 6.78 (d, 2H, J = 8.6 Hz), 6.95 (d, 2H, J = 8.6 Hz); 13C NMR (68 MHz) δ 14.4, 16,1, 20.8, 21.7, 22.9, 27.0, 27.9, 28.0, 39.0, 40.2, 42.7, 44.8, 45.8, 49.4, 49.6, 54.5, 58.3, 63.1, 65.1, 80.0. 89.2, 114.0, 116.8, 127.4, 129.9, 131,3, 136.2, 142.7, 159.2, 210.0; HRMS (EI) calcd for C31H41NO4 (M+) m/z 491.3036, found 491.3033.
27. When 27 was treated with sodium methoxide in methanol at 0 °C briefly, the 1,4-adduct of a methoxide ion at the β-carbon of the vinyl ketone moiety was obtained predominantly. We did not explore other reaction conditions for this intramolecular 1,4-addition.