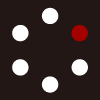
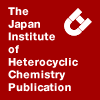
HETEROCYCLES
An International Journal for Reviews and Communications in Heterocyclic ChemistryWeb Edition ISSN: 1881-0942
Published online by The Japan Institute of Heterocyclic Chemistry
e-Journal
Full Text HTML
Received, 27th June, 2014, Accepted, 16th July, 2014, Published online, 24th July, 2014.
DOI: 10.3987/COM-14-S(K)46
■ Total Syntheses of Zwitterionic Indole Alkaloids, Flavocarpine and Dihydrovincarpine, by Extended Method for Substituted Pyridine Synthesis through Azaelectrocyclization
Yoshikatsu Hirose, Hiroshi Tsuchikawa, Toyoharu Kobayashi,* and Shigeo Katsumura*
School of Science and Technology, Kwansei Gakuin University, 2-1 Gakuen, Sanda, Hyogo 669-1337, Japan
Abstract
The total syntheses of flavocarpine (1) and dihydrovincarpine (2) have been accomplished through the common intermediate, 2,4,5-trisubstituted pyridine compound 3 which was prepared by the accelerated 6π-azaelectrocyclization of 4-alkoxycarbonylazatriene 4 followed by aromatization of the resulting dihydropyridine derivative.Flavocarpine (1) and its dihydroderivative, dihydrovincarpine (2), were isolated from stem bark of Pleiocarpa mutica Benth. and Vinca major L. var. elegantissima Hort. by Büchi’s group and Pakrashi’s group in 1962 and 1976, respectively (Figure 1).1,2 These naturally occurring compounds are unique zwitterionic indole alkaloids possessing the indolo[2,3-a]quinolizine ring system including a 1,2,4,5-tetrasubstituted pyridinium core. The total synthesis of flavocarpine (1) has been reported by two groups,1,3 but the synthesis of dihydrovincarpine (2) has not yet.
In 2000, we have developed the novel synthetic method for 2,4-disubstituted pyridines through the accelerated 6π-azaelectrocyclization followed by aromatization due to the remarkable substituent effect of the Schiff base prepared from (E)-3-alkoxycarbonyl-2,4,6-trienal and the appropriate amine.4 Furthermore, the method was extended to the more efficient one-pot variant for 2-arylpyridine synthesis.5,6 We now describe the concise total syntheses of zwitterionic indole alkaloids 1 and 2 by further extension of the previous method to 2,4,5-trisubstituted pyridine synthesis featured by the accelerated 6π-azaelectrocyclization.
The synthetic strategy of these alkaloids is outlined in Figure 2. The target molecules 1 and 2 could be synthesized from the common intermediate, 2,4,5-trisubstituted pyridine derivative 3, through the construction of the zwitterionic indolo[2,3-a]quinolizine ring system. The key intermediate 3 would be obtained via 6π-azaelectrocyclization followed by aromatization of the azatriene 4, which could be prepared by Schiff base formation between the aldehyde 5 and appropriate amine. Aldehyde 5 could be synthesized by the Migita-Kosugi-Stille coupling of the tetrasubstituted vinyl iodide 67 with vinylstannane 7.
The synthesis of aldehyde 5, the precursor of 6π-azaelectrocyclization, is shown in Scheme 1. The known 2-indolecarbaldehyde derivative 88 was reacted with carbon tetrabromide and tripheylphosphine9 to produce the corresponding dibromide in 81% yield, which was successively treated with lithium bis(trimethylsilyl)amide and then n-butyllithium to give the desired acetylene 9 in 95% yield. The regio- and stereo-selective hydrostannylation of 9 produced the (E)-vinylstannane 7 in 89% yield. The Migita-Kosugi-Stille coupling of 7 with 67 afforded the corresponding coupling product 10 in 70% yield, which was followed by oxidation with manganese dioxide to give the desired aldehyde 510 in 85% yield (Scheme 1).
With the key aldehyde 5 in hand, we next examined the construction of the 2,4,5-trisubstituted pyridine skeleton by our two methods that were previously developed for 2,4-disubstitued pyridine synthesis.4 First, the treatment of aldehyde 5 with lithium bis(trimethylsilyl)amide in THF at room temperature unexpectedly gave no desired dihydropyridine derivative 11 and the starting material 5 was only admitted by TLC. When the reaction mixture was refluxed in THF, 11 was detected by TLC and the mixture was successively treated with DDQ at room temperature, however, a trace amount of the desired trisubstituted pyridine derivative 3 was obtained. In this case, the 6π-azaelectrocyclization of the resulted Schiff base of aldehyde 5 did not proceed at room temperature due to its increased steric hindrance, and the intermediary 11 produced under reflux would not be stable for DDQ treatment. Since the condition via Peterson olefination gave unsatisfactory results, we next attempted the oxime route.4 Thus, aldehyde 5 was reacted with hydroxylamine in pyridine for 15 min, and the produced oxime derivative was continuously treated with acetyl chloride at 50 oC for 2 hour to successfully provide the desired 2,4,5-trisubstituted pyridine compound 311 in 86% yield (Scheme 2). In this case, both 6π-azaelectrocyclization and aromatization proceeded smoothly under the reaction conditions.
As the key intermediate 3 was obtained, our attention was turned to the construction of the indolo[2,3-a]quinolizine ring system for the syntheses of zwitterionic indole alkaloids 1 and 2. Hydrolysis of the TBS group of 3 led to alcohol 1312 in 94% yield, the hydroxyl group of which was then converted into a mesyl group, and the resulting mesylate 14 was treated with SiO2 to successfully provide the cyclized product 15 in 86% yield for 2 steps. Finally, treatment of 15 with aqueous NaOH solution in methanol at 60 oC resulted in the hydrolysis of both N-phenylsulfonyl and methyl ester groups, that accompanied by the aromatization to directly produce the desired flavocarpine (1)13 in 79% yield (Scheme 3).
In the final pathway from 15 to 1, the aromatization would proceed through deprotonation at the C-6 position followed by elimination of the N-phenylsulfonyl protecting group as depicted in Figure 3. Therefore, for the synthesis of dihydrovincarpine (2), cleavage of the N-phenylsulfonyl protecting group must be prior to the construction of the indolo[2,3-a]quinolizine ring system.
The synthesis of dihydrovincarpine (2) is shown in Scheme 4. After several trials, the cleavage of the N-phenylsulfonyl protecting group was achieved by hydrogenolysis of 13 with Raney Ni as a catalyst and the desired compound 1614 was obtained in 73% yield. Mesylation of 16 followed by cyclization with SiO2 afforded the expected 5,6-dihydroindolo[2,3-a]quinolizine derivative 18 in 73% yield for 2 steps. Finally, hydrolysis of the methyl ester in 18 provided dihydrovincarpine (2)15 in 85% yield without aromatization as expected.
In conclusion, we achieved the total syntheses of both flavocarpine (1) and dihydrovincarpine (2) by applying the accelerated 6π-azaelectrocyclization followed by the aromatization protocol for 2,4,5-trisubstituted pyridine synthesis. The present syntheses clearly demonstrate that the accelerated 6π-azaelectrocyclization is a powerful method for the synthesis of alkaloids possessing the polysubstituted pyridine nucleus.
ACKNOWLEDGEMENTS
This study was supported by a Grant-in-Aid for Scientific Research on Innovative Areas ‘Organic Synthesis based on Reaction Integration’ and a Grant-in-Aid for Scientific Research (C) 25410183 from the Ministry of Education, Culture, Sports, Science and Technology, Japan.
References
1. G. Büchi, R. E. Manning, and F. A. Hochstein, J. Am. Chem. Soc., 1962, 34, 3393. CrossRef
2. E. Ali, V. S. Giri, and S. C. Pakrashi, Tetrahedron Lett., 1976, 17, 4887. CrossRef
3. G. W. Gribble and D. A. Johnson, Tetrahedron Lett., 1987, 28, 5259. CrossRef
4. (a) K. Tanaka and S. Katsumura, Org. Lett., 2000, 2, 373; CrossRef (b) K. Tanaka, H. Mori, M. Yamamoto, and S. Katsumura, J. Org. Chem., 2001, 66, 3099. CrossRef
5. T. Kobayashi, S. Hatano, H. Tsuchikawa, and S. Katsumura, Tetrahedron Lett., 2008, 49, 4349. CrossRef
6. T. Sakaguchi, T. Kobayashi, S. Hatano, H. Tsuchikawa, K. Fukase, T. Tanaka, and S. Katsumura, Chem. Asian J., 2009, 4, 1573. CrossRef
7. T. Kobayashi, K. Takeuchi, J. Miwa, H. Tsuchikawa, and S. Katsumura, Chem. Commun., 2009, 3363. CrossRef
8. J. T. Kuethe, I. W. Davies, P. G. Dormer, R. A. Reamer, D. J. Mathre, and P. J. Reider, Tetrahedron Lett., 2002, 43, 29. CrossRef
9. E. J. Corey and P. L. Fuchs, Tetrahedron Lett., 1972, 13, 3769. CrossRef
10. 5: IR (KBr disk, cm–1) 2984, 2855, 1732, 1667, 1449, 1366, 1217, 1090; 1H NMR (400 MHz, CDCl3) δ 10.3 (s, 1H), 8.29 (d, 1H, J = 8.4 Hz), 7.80 (d, 1H, J = 16.8 Hz), 7.74 (d, 2H, J = 8.0 Hz), 7.54-7.25 (m, 7H), 4.04 (s, 3H), 3.93 (t, 2H, J = 6.4 Hz), 2.99 (t, 2H, J = 6.4 Hz), 2.36 (q, 2H, J = 7.2 Hz), 1.10 (t, 3H, J = 7.6 Hz), 0.69 (s, 9H), –0.38 (s, 6H); 13C NMR (100 MHz, CDCl3) δ 190.6, 168.0, 145.9, 139.3, 138.3, 136.9, 134.5, 133.8, 130.8, 129.1, 127.1, 126.8, 126.0, 124.0, 123.3, 122.5, 119.7, 115.2, 62.6, 52.6, 28.7, 25.8, 22.1, 18.3, 13.7, –5.8; ESI-HRMS m/z calcd for C31H39NNaO6SSi [M+Na]+ 604.2165, found 604.2167.
11. 3: IR (KBr disk, cm–1) 3450, 2992, 1736, 1473, 1370, 1179; 1H NMR (400 MHz, CDCl3) δ 8.69 (s, 1H), 8.17 (d, 1H, J = 8.8 Hz), 7.98 (s, 1H), 7.68 (dd, 1H, J = 1.2, 7.6 Hz), 7.51 (d, 1H, J = 7.2 Hz), 7.47-7.23 (m, 6H), 3.95 (s, 3H), 3.67 (t, 2H, J = 7.2 Hz), 3.08 (q, 2H, J = 7.6 Hz), 2.81 (t, 2H, J = 7.2 Hz), 1.35 (t, 3H, J = 7.2 Hz), 0.77 (s, 9H), –0.13 (s, 6H); 13C NMR (100 MHz, CDCl3) δ 166.3, 151.1, 149.6, 138.6, 136.9, 136.8, 135.8, 135.6, 133.4, 131.3, 128.6, 127.1, 127.0, 125.5, 124.1, 123.8, 120.0, 115.9, 62.8, 52.4, 28.0, 25.86, 25.82, 24.7, 15.5, −5.5; ESI-HRMS m/z calcd for C31H38N2NaO5SSi [M+Na]+ 601.2168, found 601.2150.
12. 13: IR (KBr disk, cm–1) 3400, 2900, 1734, 1449, 1370, 1179; 1H NMR (400 MHz, CDCl3) δ 8.64 (s, 1H), 8.22 (d, 1H, J = 8.4 Hz), 8.20 (s, 1H), 7.45-7.05 (m, 8H), 5.68 (brs, 1H), 3.97 (s, 3H), 3.76 (brs, 2H), 3.09 (q, 2H, J = 8.0 Hz), 2.81 (brt, 2H, J = 5.2 Hz), 1.35 (t, 3H, J = 7.2 Hz); 13C NMR (100 MHz, CDCl3), δ 166.1, 149.6, 148.1, 139.1, 138.3, 136.8, 136.4, 135.2, 133.7, 132.9, 131.5, 128.5, 128.1, 126.9, 126.2, 124.9, 119.4, 117.2, 61.2, 52.6, 26.9, 24.8, 15.5; ESI-HRMS m/z calcd for C25H24N2NaO4S [M+Na]+ 487.1304, found 487.1298.
13. Flavocarpine (1): IR (KBr disk, cm–1) 3100, 2926, 2450, 1900, 1595, 1527, 1466, 1390, 1080, 845, 793; 1H NMR (400 MHz, CD3OD/TFA) 9.38 (s, 1H), 9.22 (s, 1H), 8.87 (d, 1H, J = 7.2 Hz), 8.78 (d, 1H, J = 6.8 Hz), 8.40 (d, 1H, J = 8.4 Hz), 7.84 (d, 1H, J = 8.8 Hz), 7.77 (t, 1H, J = 6.8 Hz), 7.51 (t, 1H, J = 6.8 Hz), 3.31 (q, 2H, J = 7.2 Hz), 1.43 (t, 3H, J = 7.2 Hz); 13C NMR (100 MHz, CD3OD/TFA) δ 166.7, 143.8, 139.0, 138.6, 137.1, 133.2, 132.1, 131.5, 128.3, 126.3, 124.7, 123.6, 123.2, 122.4, 119.3, 114.0, 25.8, 15.1; ESI-HRMS m/z calcd for C18H15N2O2 [M+H]+ 291.1134, found 291.1146.
14. 16: IR (KBr disk, cm–1) 3300, 2932, 1732, 1601, 1437, 1275, 1096; 1H NMR (400 MHz, CDCl3) δ 9.22 (brs, 1H), 8.46 (s, 1H), 8.00 (s, 1H), 7.57 (d, 1H, J = 8.0 Hz), 7.30 (d, 1H, J = 8.4 Hz), 7.19 (t, 1H, J = 8.4 Hz), 7.08 (t, 1H, J = 7.2 Hz), 4.07 (t, 2H, J = 6.0 Hz), 3.96 (s, 3H), 3.32 (t, 2H, J = 6.0 Hz), 2.93 (q, 2H, J = 7.6 Hz), 1.25 (t, 3H, J = 7.2 Hz); 13C NMR (100 MHz, CDCl3) δ 166.4, 160.0, 148.9, 137.4, 137.2, 136.2, 132.9, 129.2, 123.5, 120.6, 119.8, 119.1, 113.1, 63.4, 63.3, 52.6, 27.6, 24.6, 15.5; ESI-HRMS m/z calcd for C19H20N2NaO3 [M+Na]+ 347.1372, found 347.1365.
15. Dihydrovincarpine (2): IR (KBr disk, cm–1) 3500, 3150, 1650, 1581, 1421, 1366, 1142; 1H NMR (400 MHz, CD3OD/TFA) δ 8.77 (s, 1H), 8.46 (s, 1H), 7.69 (d, 1H, J = 8.4 Hz), 7.50 (d, 1H, J = 8.4 Hz), 7.39 (t, 1H, J = 7.2 Hz), 7.20 (t, 1H, J = 7.2 Hz), 4.94 (t, 2H, J = 7.2 Hz), 3.47 (t, 2H, J = 8.0 Hz), 3.11 (q, 2H, J = 7.6 Hz), 1.36 (t, 3H, J = 8.0 Hz); 13C NMR (100 MHz, CD3OD/TFA) δ 166.5, 148.0, 147.3, 143.8, 141.8, 140.1, 128.1, 126.4, 126.0, 122.5, 122.0, 121.5, 119.5, 113.7, 57.6, 25.3, 20.4, 15.1; ESI-HRMS m/z calcd for C18H17N2O2 [M+H]+ 293.1290, found 293.1296.