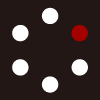
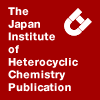
HETEROCYCLES
An International Journal for Reviews and Communications in Heterocyclic ChemistryWeb Edition ISSN: 1881-0942
Published online by The Japan Institute of Heterocyclic Chemistry
e-Journal
Full Text HTML
Received, 30th June, 2014, Accepted, 29th July, 2014, Published online, 5th August, 2014.
DOI: 10.3987/COM-14-S(K)62
■ Stereochemistry of Vinylogous Rubottom Oxidation of Proline-Fused Cyclohexadienol Silyl Ether
Kentaro Okano, Shun Okaya, Taichi Kurogi, Hideto Fujiwara, and Hidetoshi Tokuyama*
Department of Organic Chemistry, Graduate School of Pharmaceutical Sciences, Tohoku University, Aramaki, Aoba-ku, Sendai 980-8578, Japan
Abstract
The steric effect of vinylogous Rubottom oxidation of proline-fused cyclohexadienol silyl ether was investigated. The facial selectivity in the oxidation is governed by a synergistic effect of the proximal ester and a protective group on the nitrogen.γ-Hydroxyenone and its derivatives are often found in a variety of biologically active natural products1 (Figure 1). Representatively, γ-hydroxyenone is synthesized via Diels–Alder cycloaddition of singlet oxygen and a 1,3-diene followed by basic treatment (Kornblum–DeLaMare rearrangement),2 desymmetrization of a 1,4-diol,3 or ring opening of a β,γ-epoxycyclohexanone with a base4a or acid.4b As a direct method from an enone, stepwise transformation such as radicalic bromination followed by silver-mediated hydration has been employed by Allen and Danishefsky5 because allylic oxidation of an enone is generally difficult under SeO2- or CrO3-mediated conditions as a consequence of its electron-poor nature.6
As an alternative method starting from a cyclohexanone derivative, Kuwajima and co-workers reported a vinylogous Rubottom oxidation of siloxydiene 1 (Scheme 1).7 Because of the steric bias of the bicyclic system, enone 2 was exclusively converted into siloxydiene 1. Regio- and face-selective epoxidation and acidic ring opening of the resultant epoxide 3 provided γ-hydroxyenone 4 in 53% yield.
Grierson also reported a similar vinylogous Rubottom oxidation (Scheme 2).8 Epoxidation of bicyclic siloxydiene 5 with dimethyldioxirane (DMDO) occurred smoothly, even at −78 °C, from the convex face to give 6, which was transformed into γ-hydroxyenone 7 in 70% yield.
During the synthetic studies on acetylaranotin, we had to introduce a hydroxyl group at the γ-position of cyclohexenone (Scheme 3).9 On the basis of these precedents, we expected that the vinylogous Rubottom oxidation of proline-fused cyclohexadienol silyl ether 8 should occur from the convex face. However, the oxidation proceeded via unexpected α-epoxide 9 to give γ-hydroxyenone 10 with unnatural stereochemistry; γ-hydroxyenone 10 was subsequently converted into acetylaranotin via inversion of the hydroxyl group at the final stage of the total synthesis. Herein, we investigated the rationale for the unusual face selectivity in the oxidation and clarified that a synergistic effect of the proximal ester and a protective group on the nitrogen plays a key role in this synthesis.
Because we were interested in the unexpected face selectivity in the vinylogous Rubottom oxidation, we first investigated the steric effect of the methyl ester and observed that subjecting diastereomer 11 to the vinylogous Rubottom oxidation provided a mixture of the major isomer 12 and diastereomer 13 in 40% as a combined yield (Scheme 4).10
Diastereomer 11 was prepared via epimerization of the α-position of the methyl ester (Scheme 5). The synthesis commenced with TBS protection of the allylic alcohol 149 for the subsequent epimerization. Treatment of compound 15 with lithium diethylamide in THF/HMPA followed by kinetic protonation using t-BuOH provided the corresponding epimer 16, albeit in low yield.11 The allylic TBS ether was then converted into enone 18 in a two-step sequence, and 18 was transformed to dienol silyl ether 11 for the key vinylogous Rubottom oxidation.
To clarify the reason for the face selectivity that was controlled by the stereochemistry of the ester group, we calculated the most stable conformation of dienol silyl ether 8 using the MMFF94 molecular-mechanics force field in conjunction with the program CONFLEX712 (Figure 2). Interestingly, in the most stable conformation of cyclohexadienol silyl ether 8 with a pseudoequatorial methyl ester, in addition to the trimethylsilyl group, the benzyloxy group of the Cbz carbamate locates opposite the methyl ester (s-trans conformation to the methane carbon bearing the ester moiety) to reduce steric repulsion. Therefore, the aromatic ring sterically shielded the upper π-face (convex face) of diene 8, resulting in preferential oxidation at the concave face.
In contrast, in the most stable conformation of dienol silyl ether ent-11, whose stereochemistry at the ester α-position is opposite that of compound 8, the ester group locates at the pseudoaxial position (Figure 3). In addition, the pseudoequatorial α-proton of the ester forces the benzyloxy group of the Cbz group to adopt the s-cis conformation to the methane carbon bearing the ester moiety. The trimethylsilyl group shields the upper π-face (convex face), which should result in the decrease of face selectivity and lower yield of the corresponding products.
In conclusion, the unexpected facial selectivity in the vinylogous Rubottom oxidation was controlled by the stereochemistry of the proximal methyl ester. These results indicate that switching of the stereochemistry of the ester leads to a γ-hydroxyenone with another relative stereochemistry, which provides a guide to the stereochemistry of the ester for the synthesis of proline-fused γ-hydroxycyclohexenone and structurally related natural products, such as exserohilone, rostratins, and epicoccins.
EXPERIMENTAL
General Remarks: Materials were obtained from commercial suppliers and used without further purification unless otherwise mentioned. All reactions were carried out in oven-dried glassware under a slight positive pressure of argon unless otherwise noted. Anhydrous THF and dichloromethane were purchased from Kanto Chemical Co. Inc. Anhydrous diisopropylethylamine was dried and distilled according to the standard protocols. DMDO was prepared according to the reported procedure and titrated prior to use.13 Flash column chromatography was performed on Silica Gel 60N (Kanto, spherical neutral, 40–50 µm) using the indicated eluent. Preparative TLC and analytical TLC were performed on Merck 60 F254 glass plates precoated with a 0.25 mm thickness of silica gel. IR spectra were measured on a JASCO FT/IR-4100 spectrometer. NMR spectra were recorded on a JEOL ECA600 spectrometer and JNM-AL400 spectrometer. For 1H NMR spectra, chemical shifts are expressed in parts per million downfield from internal tetramethylsilane (δ 0) or relative internal CHCl3 (δ 7.26). For 13C NMR spectra, chemical shifts are expressed in ppm downfield from relative internal CDCl3 (δ 77.0). Mass spectra were recorded on a Bruker micrOTOF II (ESI).
(2R,3aS,4S,7aS)-(+)-1-Benzyl 2-methyl 4-hydroxy-2,3,3a,4,7,7a-hexahydro-1H-indole-1,2-dicarboxylate (14): a colorless oil. The analytical data (Rf, IR, HRMS, 1H and 13C NMR) were identical with those reported in the literature.9 [α]D25 118 (c 1.03, CHCl3).
Formation of dienol silyl ether and vinylogous Rubottom Oxidation. A flame-dried 10-mL screw-top test tube equipped with a Teflon-coated magnetic stirring bar and a rubber septum was charged with enone 18 (22.5 mg, 68.3 µmol), i-Pr2NEt (35.0 µL, 205 µmol), and dry CH2Cl2 (0.68 mL) under an argon atmosphere. To the solution was added TMSOTf (24.7 µL, 137 µmol) and the mixture was stirred at room temperature. To the resulting mixture was added additional TMSOTf after 30 min (2.5 µL, 14 µmol) and 40 min (2.5 µL, 14 µmol), respectively. The resulting mixture was stirred for another 10 min, after which time TLC (hexanes-EtOAc = 6:1, developed twice) indicated complete consumption of 18. The reaction mixture was cooled to 0 °C and was treated with 1 M aqueous HCl. The organic layer was separated and washed with H2O three times and brine. The organic extracts were dried over anhydrous sodium sulfate, and filtered. The organic solvents were removed under reduced pressure to give a crude dienol silyl ether 11.
The crude dienol silyl ether 11 was diluted with dry CH2Cl2 (0.91 mL), and the mixture was treated with DMDO (0.104 M in acetone, 660 µL, 68.6 µmol) at –78 °C. The resulting suspension was stirred at –78 °C for 30 min, after which time TLC (hexanes-EtOAc = 1:1) indicated complete consumption of 11. The organic solvents were removed under reduced pressure to give a crude material, which was dissolved in CH2Cl2 and treated with acidic silica gel (1 g). The resulting mixture was dried under reduced pressure and passed through glass filter using EtOAc as an eluent. The filtrate was concentrated under reduced pressure give a crude γ-hydroxyenone, which was purified by preparative TLC (CH2Cl2-MeOH = 40:1, developed five times) to afford γ-hydroxyenone 12 (5.2 mg, 15 µmol, 22%) and 13 (4.0 mg, 12 µmol, 18%).
(2S,3aS,7R,7aR)-(+)-1-Benzyl 2-methyl 7-hydroxy-4-oxo-2,3,3a,4,7,7a-hexahydro-1H-indole-1,2-dicarboxylate (12): colorless oil: Rf = 0.61 (CH2Cl2-MeOH = 40:1, developed three times); IR (neat, cm–1): 3428, 3033, 2953, 1747, 1678, 1419, 1354, 1306, 1244, 1217, 1120, 1073, 793, 749, 699; 1H NMR (400 MHz, CDCl3): δ 7.49–7.28 (J = 10.4, 2.0 Hz), 6.03 (dd, 1H, J = 10.4, 2.0 Hz), 5.24 (d, 1H, J = 12.0 Hz), 5.07 (d, 1H, J = 12.0 Hz), 4.88–4.78 (m, 2H), 4.49–4.36 (m, 2H), 3.79 (s, 0.5H), 3.54 (s, 2.5H), 3.07 (ddd, 1H, J = 13.2, 8.4, 8.4 Hz), 2.67 (ddd, 1H, J = 13.2, 7.6, 7.6 Hz), 1.97 (ddd, 1H, J = 12.8, 12.8, 9.6 Hz); 13C NMR (100 MHz, CDCl3): δ 195.3, 172.1, 156.4, 150.5, 135.3, 128.6, 128.5, 128.3, 127.1, 70.1, 68.4, 65.5, 59.3, 52.5, 45.7, 32.9; [α]D25 20.8 (c 0.380 CHCl3); HRMS (ESI): Calcd. for Calcd. for C18H19NNaO6 (M++Na), 368.1105; Found: 368.1104.
(2S,3aS,7S,7aR)-(+)-1-Benzyl 2-methyl 7-hydroxy-4-oxo-2,3,3a,4,7,7a-hexahydro-1H-indole-1,2-dicarboxylate (13): colorless oil: Rf = 0.55 (CH2Cl2-MeOH = 40:1, developed three times); IR (neat, cm–1): 3461, 2953, 1748, 1707, 1683, 1418, 1358, 1299, 1212, 1125, 1095, 1016, 769, 698; 1H NMR (400 MHz, CDCl3): δ 7.47–7.27 (m, 5H), 6.99–6.86 (m, 1H), 6.20–6.10 (m, 1H), 5.21 (d, 0.5H, J = 12.4 Hz), 5.18-5.07 (m, 1H), 5.03 (d, 0.5H, J = 12.4 Hz), 4.62 (ddd, 1H, J = 18.8, 10.4, 8.0 Hz), 4.46 (dd, 0.5H, J = 6.8, 6.8 Hz), 4.37 (dd, 0.5 H, J = 8.0, 8.0 Hz), 3.79 (s, 1.5H), 3.56 (s, 1.5H), 3.35–3.22 (m, 0.5H), 3.17–3.04 (m, 0.5H), 2.84–2.69 (m, 1H), 2.37–2.16 (m, 2H); 1H NMR (400 MHz, DMSO-d6, 100 °C): δ 7.39–7.26 (m, 5H), 6.92 (ddd, 1H, J = 10.4, 5.6, 2.4 Hz), 5.99 (dd, 1H, J = 10.4, 2.4 Hz), 5.51 (s, 1H), 5.14–5.02 (m, 2H), 4.41 (dd, 1H, J = 9.2, 7.6 Hz), 4.21 (dd, 1H, J = 8.4, 7.6 Hz), 3.61 (s, 3H), 2.32–2.25 (m, 1H), 2.11 (dd, 1H, J = 13.2, 10.0 Hz) (two protons are missing due to overlap); 13C NMR (150 MHz, CDCl3): δ 197.6, 197.4, 173.1, 173.0, 154.2, 153.9, 149.3, 149.0, 136.1, 136.0, 128.6, 128.4, 128.2, 128.1, 127.9, 127.8, 125.6, 79.8, 79.2, 67.5, 67.4, 63.6, 63.3, 58.3, 58.1, 52.7, 52.4, 41.3, 40.3, 32.0. 31.6 (complexity due to rotamers; [α]D27 35.9 (c 0.320 CHCl3); HRMS (ESI): Calcd. for Calcd. for C18H19NNaO6 (M++Na), 368.1105; Found: 368.1086.
(2R,3aS,4S,7aS)-(+)-1-Benzyl 2-methyl 4-((tert-butyldimethylsilyl)oxy)-2,3,3a,4,7,7a-hexahydro-1H-indole-1,2-dicarboxylate (15): A flame-dried 50-mL two-necked round-bottomed flask equipped with a Teflon-coated magnetic stirring bar, a rubber septum, and an inlet adapter with three-way stopcock was charged with allyl alcohol 14 (447 mg, 1.35 mmol), 2,6-lutidine (314 µL, 2.70 mmol) and dry CH2Cl2 (13.5 mL) under argon atmosphere. To the solution was added TBSOTf (371 µL, 1.62 mmol) at 0 °C. The resulting mixture was stirred at 0 °C for 10 min, after which time TLC (hexanes-EtOAc = 1:1) indicated complete consumption of 14. The reaction was quenched with 1 M aqueous HCl, and the aqueous layer was extracted with CH2Cl2 three times. The combined organic extracts were dried over anhydrous sodium sulfate and filtered. The organic solvents were removed under reduced pressure to give a crude material, which was purified by flash silica gel chromatography (hexanes-EtOAc = 6:1) to afford TBS ether 15 (469 mg, 1.05 mmol, 78%) as a colorless oil. Rf = 0.66 (hexanes-EtOAc = 1:1); IR (neat, cm–1): 3033, 2953, 1750, 1710, 1413, 1351, 1294, 1204, 1176, 1119, 1067, 1042, 874, 837, 775, 749, 698; 1H NMR (400 MHz, CDCl3): δ 7.40–7.25 (m, 5H), 5.77–5.57 (m, 2H), 5.26–5.18 (m, 1H), 5.10 (d, 0.5H, J = 12.8 Hz), 4.99 (d, 0.5H, J = 12.0 Hz), 4.36–4.26 (m, 1H), 4.21–4.10 (m, 1H), 4.05–3.93 (m, 1H), 3.76 (s, 1.5H), 3.55 (s, 1.5H), 2.85–2.74 (m, 0.5H), 2.67–2.42 (m, 1.5H), 2.10–1.83 (m, 2H), 1.81–1.63 (m, 1H), 0.88 (s, 9H), 0.06 (s, 6H); 13C NMR (100 MHz, CDCl3): δ 172.9, 172.6, 154.7, 154.1, 136.4, 136.3, 129.5, 128.5, 128.3, 128.2, 127.80, 127.77, 127.7, 127.65, 127.6, 125.6, 125.1, 67.6, 67.0, 66.9, 66.7, 58.0, 57.8, 52.7, 52.1, 51.9, 51.5, 44.0, 43.4, 32.8, 31.6, 27.9, 27.4, 25.69, 25.66, 18.0, 17.9, –4.6, –4.7, –4.8 (complexity due to rotamers); [α]D25 97.6 (c 1.62, CHCl3); HRMS (ESI): Calcd. for C24H36NO5Si (M++H), 446.2357; Found: 446.2346.
(2S,3aS,4S,7aS)-(+)-1-Benzyl 2-methyl 4-((tert-butyldimethylsilyl)oxy)-2,3,3a,4,7,7a-hexahydro-1H-indole-1,2-dicarboxylate (16): A flame-dried 50-mL two-necked round-bottomed flask equipped with a magnetic stirring bar, a rubber septum, and an inlet adapter with three-way stopcock was charged with diethylamine (400 µL, 3.87 mmol) and dry THF (10 mL) under argon atmosphere. To the solution was added n-BuLi in hexane (1.52 M, 2.34 mL, 3.56 mmol) at 0 °C. The resulting mixture was stirred at 0 °C for 20 min. A portion of this solution (5.5 mL) was discarded via syringe, and the rest of the resulting solution was then cooled to –78 °C. Another flame-dried 50-mL two-necked round-bottomed flask equipped with a magnetic stirring bar, a rubber septum, and an inlet adapter with three-way stopcock was charged with TBS ether 15 (224 mg, 503 µmol) and dry THF (14.4 mL) under an argon atmosphere. While the previously described solution was stirred for 20 min at –78 °C, HMPA (1.44 mL) and the solution of substrate 15 in THF were added. The resulting mixture was stirred for 20 min before quenching with t-BuOH (1.41 mL) in dry THF (2.83 mL), followed immediately by saturated aqueous NH4Cl at –78 °C and the aqueous layer was extracted twice with Et2O. The combined organic layers were washed with 1 M aqueous HCl and saturated aqueous NaHCO3. The organic extracts were dried over anhydrous sodium sulfate, and filtered. The organic solvents were removed under reduced pressure to give a crude TBS ether 16, which was purified by MPLC (toluene-Et2O = 19:1) and GPC to afford TBS ether 16 (48.6 mg, 109 µmol, 22%) as a colorless oil. Rf = 0.65 (hexanes-EtOAc = 1:1); IR (neat, cm–1): 3032, 2953, 1751, 1709, 1415, 1352, 1292, 1200, 1175, 1110, 1071, 1042, 871, 837, 774, 745, 697; 1H NMR (400 MHz, CDCl3): δ 7.40–7.27 (m, 5H), 5.78–5.57 (m, 2H), 5.24–4.94 (m, 2H), 4.42–4.09 (m, 2H), 4.07–3.98 (m, 1H), 3.75 (s, 1.5H), 3.56 (s, 1.5H), 2.86–2.72 (m, 0.5H), 2.71–2.58 (m, 0.5H), 2.44–2.28 (m, 2H), 2.26–2.11 (m, 1H), 1.81–1.64 (m, 1H), 0.89 (s, 9H), 0.07 (s, 6H); 13C NMR (100 MHz, CDCl3): δ 173.3, 173.1, 154.6, 154.0, 136.5, 128.4, 127.9, 127.8, 125.8, 125.6, 67.1, 66.7, 58.5, 52.4, 52.2, 52.0, 51.8, 45.8, 45.2, 32.8, 31.9, 27.4, 27.0, 25.8, 18.1, –4.5, –4.8 (complexity due to rotamers); IR (neat, cm–1): 3032, 2953, 1751, 1709, 1415, 1352, 1042, 837, 774; [α]D24 50.5 (c 0.700, CHCl3); HRMS (ESI): Calcd. for C24H36NO5Si (M++H), 446.2357; Found: 446.2359.
(2S,3aS,4S,7aS)-(+)-1-Benzyl 2-methyl 4-hydroxy-2,3,3a,4,7,7a-hexahydro-1H-indole-1,2-dicarboxylate (17): A 10-mL screw-top test tube equipped with a Teflon-coated magnetic stirring bar was charged with TBS ether 16 (46.4 mg, 104 µmol) and dry THF (208 µL). To the solution was added TBAF in THF (1 M, 208 µL, 208 µmol) at room temperature. The resulting mixture was stirred at room temperature for 16 h, after which time TLC (hexanes-EtOAc = 6:1, developed twice) indicated incomplete consumption of 16. To the reaction mixture was added additional TBAF in THF (1 M, 52.0 µL, 52.0 µmol). After another 1.5 h, the reaction mixture was treated with saturated aqueous NH4Cl and aqueous layer was extracted with EtOAc three times. The combined organic extracts were dried over anhydrous sodium sulfate and filtered. The organic solvents were removed under reduced pressure to give a crude material, which was purified by flash silica gel chromatography (hexanes-EtOAc = 1:1) to afford allyl alcohol 17 (34.2 mg, 103 µmol, 99%) as a colorless oil. Rf = 0.20 (hexanes-EtOAc = 1:1); IR (neat, cm–1): 3444, 3031, 2952, 1748, 1697, 1417, 1356, 1294, 1215, 1001, 751, 698; 1H NMR (400 MHz, CDCl3): δ 7.45–7.29 (m, 5H), 5.86–5.70 (m, 2H), 5.24–4.97 (m, 2H), 4.44–4.16 (m, 2H), 4.14–4.02 (m, 1H), 3.76 (s, 1.5H), 3.56 (s, 1.5H), 2.87–2.75 (m, 0.5H), 2.72–2.60 (m, 0.5H), 2.52–2.39 (m, 2H), 2.36–2.21 (m, 1H), 1.80–1.65 (m, 1H); 13C NMR (100 MHz, CDCl3): δ 173.2, 173.1, 154.5, 154.2, 136.5, 136.3, 128.5, 128.4, 128.0, 127.8, 127.2, 126.2, 126.0, 67.1, 65.5, 58.5, 58.4, 52.3, 52.1, 51.6, 51.1, 44.7, 44.2, 32.8, 31.8, 27.5, 27.0; IR (neat, cm–1): 2924, 2856, 1695, 1452, 1435, 1267, 1258, 1016, 868; [α]D24 42.9 (c 0.590, CHCl3); HRMS (ESI): Calcd. for C18H21NNaO5 (M++Na), 354.1312; Found: 354.1309.
(2S,3aS,7aS)-(+)-1-Benzyl 2-methyl 4-oxo-2,3,3a,4,7,7a-hexahydro-1H-indole-1,2-dicarboxylate (18): A 10-mL screw-top test tube equipped with a Teflon-coated magnetic stirring bar was charged with allyl alcohol 17 (34.2 mg, 103 µmol), IBX (43.4 mg, 155 µmol) and EtOAc (413 µL). The resulting mixture was stirred at reflux for 25 min, after which time TLC (hexanes-EtOAc = 1:1) indicated complete consumption of 17. The reaction mixture was cooled to room temperature and passed through a pad of Celite using EtOAc as an eluent. The filtrate was concentrated under reduced pressure to give a crude material, which was purified by flash silica gel chromatography (hexanes-EtOAc = 2:1 to 1:1) to afford enone 18 (31.0 mg, 94.1 µmol, 91%) as a colorless oil. Rf = 0.43 (hexanes-EtOAc = 1:1); IR (neat, cm–1): 3033, 2952, 1748, 1707, 1673, 1415, 1355, 1296, 1208, 1175, 1113, 1001, 750, 699; 1H NMR (400 MHz, CDCl3): δ 7.49–7.28 (m, 5H), 7.03–6.81 (m, 1H), 6.19–6.02 (m, 1H), 5.27–4.98 (m, 2H), 4.68–4.34 (m, 2H), 3.76 (s, 1.5H), 3.57 (s, 1.5H), 3.23–2.84 (m, 2H), 2.83–2.56 (m, 2H), 2.24–1.99, (m, 1H); 13C NMR (100 MHz, CDCl3): δ 196.7, 172.7, 154.0, 153.8, 147.6, 147.3, 136.0, 128.5, 128.4, 128.2, 128.1, 128.0, 127.84, 127.80, 67.5, 67.3, 59.1, 58.8, 56.7, 56.2, 52.5, 52.3, 47.8, 47.1, 32.3, 31.4, 28.3, 27.6; [α]D26 56.2 (c 1.27, CHCl3); HRMS (ESI): Calcd. for C18H19NNaO5 (M++Na), 352.1155; Found: 352.1138.
ACKNOWLEDGMENTS
This work was financially supported by the Ministry of Education, Culture, Sports, Science, and Technology, Japan; the Cabinet Office, Government of Japan through its “Funding Program for Next Generation World-Leading Researchers (LS008),” and the KAKENHI, a Grant-in-Aid for Scientific Research (A) (26253001) for H.T.; the KAKENHI, a Grant-in-Aid for Scientific Research (C) (25460003), a Grant-in-Aid for Scientific Research on Innovative Areas “Molecular Activation Directed toward Straightforward Synthesis” (25105705), a Research Grant from the Tokyo Biochemical Research Foundation (TBRF), and a Research Grant from the Research Foundation for Pharmaceutical Sciences for K.O.
References
1. (a) K. Sugawara, F. Sugawara, G. A. Strobel, Y. Fu, H. Cun-Heng, and J. Clardy, J. Org. Chem., 1985, 50, 5631; CrossRef (b) R. X. Tan, P. R. Jensen, P. G. Williams, and W. Fenical, J. Nat. Prod., 2004, 67, 1374; CrossRef (c) H. Guo, B. Sun, H. Gao, X. Chen, S. Liu, X. Yao, X. Liu, and Y. Che, J. Nat. Prod., 2009, 72, 2115; CrossRef (d) J.-M. Wang, G.-Z. Ding, L. Fang, J.-G. Dai, S.-S. Yu, Y.-H. Wang, X.-G. Chen, S.-G. Ma, J. Qu, S. Xu, and D. Du, J. Nat. Prod., 2010, 73, 1240. CrossRef
2. (a) N. Kornblum and H. E. DeLaMare, J. Am. Chem. Soc., 1951, 73, 880; CrossRef For a catalytic enantioselective synthesis using a chiral amine, (b) S. T. Staben, X. Linghu, and F. D. Toste, J. Am. Chem. Soc., 2006, 128, 12658; CrossRef For a review, see (c) M. Balci, Chem. Rev., 1981, 81, 91. CrossRef
3. M. C. Willis, J. Chem. Soc., Perkin Trans. 1, 1999, 1765. CrossRef
4. (a) M. Acemoglu, P. Uebelhart, M. Rey, and C. H. Eugster, Helv. Chim. Acta, 1988, 71, 931; CrossRef (b) H. Yamamoto and T. Oritani, Tetrahedron Lett., 1995, 36, 5797. CrossRef
5. J. G. Allen and S. J. Danishefsky, J. Am. Chem. Soc., 2001, 123, 351. CrossRef
6. D. B. Ushakov and M. E. Maier, Synlett, 2013, 24, 705. CrossRef
7. M. Seto, K. Sakurai, Y. Horiguchi, and I. Kuwajima, Synlett, 1994, 993. CrossRef
8. S. Piguel, G. Ulibarri, and D. S. Grierson, Tetrahedron Lett., 1999, 40, 295. CrossRef
9. H. Fujiwara, T. Kurogi, S. Okaya, K. Okano, and H. Tokuyama, Angew. Chem. Int. Ed., 2012, 51, 13062. CrossRef
10. Compounds 12 and 13 were identified by comparison of the protons of enone with those of compound 10 and its diastereomer.
11. P. Wipf and J.-L. Methot, Org. Lett., 2000, 2, 4213. CrossRef
12. (a) H. Goto and E. Osawa, J. Am. Chem. Soc., 1989, 111, 8950; CrossRef (b) H. Goto and E. Osawa, J. Chem. Soc., Perkin Trans. 2, 1993, 187; CrossRef (c) CONFLEX7, H. Goto, S. Obata, N. Nakayama, and K. Ohta, CONFLEX Corporation, Tokyo, Japan, 2012.
13. W. Murray and M. Singh, Org. Synth., Coll. Vol. IX, 1998, 288.