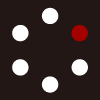
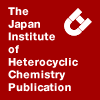
HETEROCYCLES
An International Journal for Reviews and Communications in Heterocyclic ChemistryWeb Edition ISSN: 1881-0942
Published online by The Japan Institute of Heterocyclic Chemistry
e-Journal
Full Text HTML
Received, 30th June, 2014, Accepted, 7th August, 2014, Published online, 18th August, 2014.
DOI: 10.3987/COM-14-S(K)59
■ Rhodium-Catalyzed 2-Methylthiolation Reaction of Thiazoles/Oxazoles Using 2-(Methylthio)thiazole
Mieko Arisawa,* Yuri Nihei, and Masahiko Yamaguchi*
Graduate School of Pharmaceutical Sciences, Tohoku University, Aramaki, Aoba-ku, sendai 980-8578, Japan
Abstract
RhH(PPh3)4 and 1,3-bis(dicyclohexyl)phosphinopropane (dcypp) catalyze the 2-methylthiolation of oxazoles and thiazoles using 2-(methylthio)thiazole as a thiolating reagent. The methylthio transfer reaction is under equilibrium, and various 2-methylthiolated thiazoles and oxazoles were obtained in moderate to good yields by removing thiazole under refluxing o-dichlorobenzene.INTRODUCTION
2-Organothiolated thiazoles and oxazoles belong to an important class of heterocyclic compounds in medicinal, biological, and natural product chemistry.1 2-Organothiolated azoles are synthesized by either the reaction of metal thiolates with 2-halo azoles or the arylation/alkylation reaction of azolyl-2-thiol.2 Both methods use stoichiometric amounts of metal reagents and/or metal bases. An attractive method of synthesizing 2-thiolated azoles is the catalytic conversion of the C-H bond to the C-S bond. Such 2-thiolation reactions of azoles employing transition-metal cayalysis are rare, although many examples of 2-arylation reactions of azoles and benzoazoles have been reported using stoichiometric amounts of bases or oxidizing reagents.3
Copper- and Lewis-acid (AgI, NiII, or FeII)-catalyzed 2-thiolation of azoles and benzoazoles with thiols was conducted under oxidative conditions using stoichiometric amounts of CuO, Cu(OAc)2, or oxygen.4 Copper-catalyzed reactions were carried out using stoichiometric amounts of bases such as K2CO3 and Cs2CO3.5 Recently, azoles and benzoazoles have been converted to 2-thiolated products using diaryl disulfides and stoichiometric amounts of bases such as tBuOLi and Cs2CO3.6 A mechanism involving the addition of copper thiolates to the CN double bond followed by oxidation was suggested by calculations,7 and a 2-deprotonation mechanism of azoles was also proposed.4a, 4b, 5a, 5c, 6 A general method that allows for the conversion of azoles to 2-thiolated azoles via CH bond activation is desirable, particularly without using stoichiometric amounts of bases or oxidizing reagents being a source of a large chemical energy.8
Previously, we developed a series of rhodium-catalyzed organothiolation reactions of organic compounds with pKa values of 16-27 using disulfide or organosulfur compounds.9 The reactions involve the transfer of an organothio group between organic compounds, and do not use stoichiometric amounts of a base and an oxidizing reagent. During the course of studies, we reported the 2-organothiolation reaction of benzothiazoles/benzoxazoles using α-(phenylthio)isobutyrophenone.9e The thiolation reaction of the less acidic substrates was then examined, and described in this paper is the rhodium-catalyzed 2-methylthiolation of oxazoles and thiazoles using 2-(methylthio)thiazole as another thiolation reagent (Scheme 1). This equilibrium reaction was promoted by removing volatile thiazole. The thiolation reactions are affected by pKa as indicated by the different reactivities of the less acidic azoles (pKa 27-29) from benzoazoles (pKa 24-27).10 The results, unlike conventional oxidative and deprotonation methods, are consistent with the involvement of the C-H activation mechanism.
RESULTS AND DISCUSSION
On the basis of our previous observations,9e the reaction of 4-phenylthiazole 1a and 2-(methylthio)benzothiazole 2 (1 equiv) was examined. When 1a (1 equiv) was treated with 2 (1 equiv) in the presence of RhH(PPh3)4 (10 mol%) and dcypp (20 mol%) in refluxing chlorobenzene for 3 h, 2-methylthio-4-phenyloxazole 3a was obtained in 23% yield along with benzothiazole 4 in 26% yield (Scheme 2). When the molar ratio of 2:1a was increased from 1 to 3, the yield of 3a increased to 36%. The use of dppe in place of dcypp decreased the yield to 12%. The equilibrium nature of the reaction was confirmed by the reverse reaction. When an equimolar amounts of 3a and benzothiazole 4 were treated with the same catalyst in refluxing chlorobenzene for 3 h, 1a and 2 were obtained in 46 and 48% yields, respectively. The rhodium complex catalyzes the equilibration of the methylthio transfer reaction between oxazole 1a and 2-(methylthio)benzothiazole 2. That the latter is thermodynamically stable is consistent with the pKa values of benzothiazole (pKa 27.0) and thiazole (pKa 29.4).10
The use of 2-(methylthio)thiazole 5 in place of 2 was then examined because of the less acidic nature of thiazoles than of benzothiazole. When 1a (1 equiv) was treated with 5 in the presence of RhH(CO)(PPh3)3 (10 mol%) and dcypp (20 mol%) in refluxing o-dichlorobenzene (180 °C) for 3 h, 2-methylthio-4-phenyloxazole 3a was obtained in 52% yield (Scheme 3). The reaction was accompanied by the formation of thiazole 6. No reaction occurred in the absence of either the rhodium complex or dcypp. The reverse reaction of 3a and 6 gave 1a and 5 in 31% and 12% yields, respectively. The result confirmed the equilibrium reaction and the favored formation of 3a. The yield of 3a decreased to 32% when the reaction temperature was lowered to that of refluxing chlorobenzene (132 °C). The higher yield at high temperature was ascribed to the equilibrium shift with the removal of thiazole 6 (bp. 117-118 °C). The presence of 6 was confirmed by the 1H-NMR analysis of vapor collected at the top of the refluxing condenser. The rhodium complex can effectively transfer a methylthio group between thiazoles.
When the molar ratio of 5:1a was changed from 1 to 3, the yield of 3a increased to 74%, which was accompanied by 2,5-di(methylthio)thiazole 7 (10%) (Table 1, entry 1). The treatment of 5 under the same catalytic conditions in the absence of 1a gave 7 in 8% yield (Scheme 4). Thus, the formation of 7 was due to the disproportionation of 5.
Various 4- and 5-substituted thiazole derivatives 1a-j were reacted with 5 (3 equiv) in the presence of the rhodium catalyst. 4-Arylthiazoles 1a-e gave 2-methylthiolated products 3a-e in moderate yields (entries 1-5). The reaction could be effectively applied to aliphatic substituted derivatives 1f-i containing methyl, methoxyethyl, vinyl, and trimethylsilyl groups without affecting the functional groups (entries 6-9). The yield of the methylthiolation reaction of 4-methoxycarbonylthiazole 1j slightly decreased to 30% yield (entry 10). 2-Metylthiolated thiazoles 3h, 3i, and 3j possessing vinyl, trimethylsilyl, and methoxycarbonyl groups can be used for the synthesis of multiple-substituted thiazoles. Benzothiazole 4 was also converted to 2-(methylthio)benzothiazole 2 in 79% yield (entry 11). The 2-thiolation reaction of 2-chloro-4-phenylthiazole did not occur, and the starting materials were recovered, which indicated the involvement of rhodium-catalyzed 2-CH bond activation rather than the nucleophilic reaction of thiolate.
Rhodium-catalyzed 2-methylthiolation was also applied to 4- and 5-substituted oxazoles. When 4-phenyloxazole 8a was reacted with 5 (3 equiv) in the presence of RhH(CO)(PPh3)3 (10 mol%) and dcypp (20 mol%) in refluxing o-dichlorobenzene for 3 h, 2-(methylthio)-4-phenyloxazole 9a (58%) was obtained (Table 2, entry 1). The yield of 9a decreased to 33% using a molar ratio of 8a:5 of 1 (entry 1). Various 4-aryl oxazoles 8a-e reacted with 5 (3 equiv) giving 4-aryl-2-(methylthio)oxazoles in moderate to good yields (entries 1-5). The p-electron-donating groups on the aryl moiety affect the increase in yield compared with the p-electron-withdrawing groups. 4,5-Diphenyloxazole 8f also reacted efficiently (entry 6). The 2-methylthiolation of oxazoles possessing alkyl substituents such as 4-(t-butyl)oxazole 8g and 5-butyl-4-(pentyl)oxazole 8h gave 2-methylthiolated products 9g and 9h in moderate yields (entries 7 and 8). Thus, the 2-methylthiolation reaction of oxazoles (pKa 27.1) proceeded as well as that of thiazoles with the less acidic 2-protons (pKa 29.4).
A possible mechanism of the reaction follows (Figure 1). The initial step is the oxidative addition of thiazoles/oxazoles to the rhodium(I) complex to provide the C-Rh(III)-H intermediates. The rhodium(III) intermediate then undergo the reaction with 2-(methilthio)thiazole 5 forming C-Rh(III)-SMe complex and thiazole 6, and the 2-methylthiolated products are liberated by the reductive elimination with the regeneration of the rhodium catalyst. The present methylthiolation reaction of the thiazoles/oxazoles is a reversible reaction.
RhH(PPh3)4 and dcypp catalyze the 2-methylthiolation of oxazoles and thiazoles using 2-(methylthio)thiazole 5 as a thiolating reagent. The reaction was promoted by using 5 than 2-(methylthio)benzothiazole 2. Various 2-methylthiolated azoles were obtained in moderate yields by removing thiazole under refluxing o-dichlorobenzene to shift the equilibrium. The intermolecular reversible transfer of a methylthio group takes place between azoles, using a small activation energy without using a large chemical energy derived from bases and oxidizing reagents.
EXPERIMENTAL
General: 1H-, 13C-NMR spectra were recorded on a Varian Mercury (400 MHz). Tetramethylsilane was used as standard. IR spectra were measured on a JASCO FT/IR-410 spectrophotometer. Melting points were determined with a Yanaco micro melting point apparatus without correction. High- and low-resolution mass spectra were measured on a JEOL JMS-DX-303, a JEOL JMS-700, or a JMS-T100GC. Merck silica gel 60 (63-200 µm) was employed for flash column chromatography.
2-(Methylthio)-4-phenylthiazole 3a: 1,3-Bis(dicyclohexylphosphino)propane (dcypp: 20.0 mol%, 21.9 mg) was dissolved in o-dichlorobenzene (1.0 mL) in a two-necked flask equipped under argon atmosphere. Then, RhH(CO)(PPh3)3 (10.0 mol%, 23.0 mg), 4-phenylthiazole 1a (0.25 mmol, 40.3 mg) and 2-methylthiothiazole 5 (0.75 mmol, 78 μL) were added to the solution, and the mixture was heated at reflux for 3 h. Then, the solvent was removed under reduced pressure, and the residue was purified by flash columm chromatography on silica gel giving 2-(methylthio)-4-phenylthiazole 3a (38.5 mg, 74%). 3a11: Colorless oil. 1H-NMR (400 MHz, CDCl3) δ 2.76 (3H, s), 7.33 (2H, m), 7.41 (2H, t, J = 7.6 Hz), 7.89 (2H, d, J = 7.2 Hz). 13C-NMR (100 MHz, CDCl3) δ 16.7, 111.8, 126.3, 128.1, 128.7, 134.1, 155.4, 166.2. IR (neat) ν 1477, 1418, 1053, 1037,722, 691. MS (EI) m/z 207 (M+, 100%). HRMS Calcd for C10H9NOS: 207.0176, Found: 207.0161.
Thiazole (6)12: Colorless oil. 1H-NMR (400 MHz, CDCl3) δ 7.30 (1H, m), 7.86 (1H, d, J = 2.8 Hz), 8.76 (1H, s). 13C-NMR (100 MHz, CDCl3) δ 118.4, 143.2, 152.5. IR (neat) ν 3411, 3085, 1481, 1380, 1319, 1121, 866. MS (EI) m/z 85 (M+, 100%), 58 (M+-27, 76%). HRMS Calcd for C3H3NS: 84.9986. Found: 84.9936.
2,5-Bis(methylthio)thiazole (7): Yellow oil. 1H-NMR (400 MHz, CDCl3) δ 2.43 (3H, s), 2.68 (3H, s), 7.54 (1H, s). 13C-NMR (100 MHz, CDCl3) δ 16.3, 22.4, 131.3, 146.2, 169.3. IR (neat) ν 2920, 1725, 1479, 1430, 1379, 1312, 1028, 965. MS (EI) m/z 177 (M+, 100%), 144 (M+-33, 73%). HRMS Calcd for C5H7NS3: 176.9741. Found: 176.9724.
4-(4-Methoxyphenyl)-2-(methylthio)thiazole (3b)13: Colorless crystals. Mp 83.5~84.5 ℃ (Hexane). 1H-NMR (400 MHz, CDCl3) δ 2.74 (3H, s), 3.84 (3H, s), 6.93 (2H, d, J = 8.4 Hz), 7.19 (1H, s), 7.82 (1H, d, J = 8.4 Hz). 13C-NMR (100 MHz, CDCl3) δ 16.7, 55.3, 110.0, 114.0, 127.1, 127.6, 155.2, 159.6, 165.9. IR (KBr) ν 1606, 1482, 1411, 1249, 1175, 1050, 1024, 835, 741. MS (EI) m/z 237 (M+, 100%). HRMS Calcd for C11H11NOS2: 237.0282, Found: 237.0274.
4-(4-Methylphenyl)-2-(methylthio)thiazole (3c): Colorless crystals. Mp 52.0 ℃ (Hexane). Lit.11 59-60 °C. 1H-NMR (400 MHz, CDCl3) δ 2.37 (3H, s), 2.74 (3H, s), 7.20 (2H, d, J = 8.0 Hz), 7.27 (1H, s), 7.77 (2H, d, J = 8.0 Hz). 13C-NMR (100 MHz, CDCl3) δ 16.7, 21.2, 111.1, 126.2, 129.3, 131.4, 138.0, 155.5, 165.9. IR (KBr) ν 3113, 1483, 1420, 1053, 969, 827, 746. MS (EI) m/z 225 (M+, 100%), 210 (M+-CH3, 32%). HRMS Calcd for C11H11NS2: 221.0333, Found: 221.0320.
4-(4-Chlorophenyl)-2-(methylthio)thiazole (3d)13: Colorless crystals. Mp 93.5~94.5 ℃ (Hexane). 1H-NMR (400 MHz, CDCl3) δ 2.78 (3H, s), 7.31 (1H, s), 7.36 (2H, d, J = 8.4 Hz), 7.81 (2H, d, J = 8.4 Hz). 13C-NMR (100 MHz, CDCl3) δ 16.7, 112.1, 127.5, 128.8, 132.6, 133.8, 154.1, 166.6. IR (KBr) ν 1472, 1419, 1088, 1057, 736. MS (EI) m/z 241 (M+, 100%). HRMS Calcd for C10H8NS2: 240.9787, Found: 240.9789.
2-(Methylthio)-4-(4-trisfluoromethyphenyl)thiazole (3e): Colorless crystals. Mp 74.0~75.0 ℃ (Hexane). 1H-NMR (400 MHz, CDCl3) δ 2.76 (3H, s), 7.44 (1H, s), 7.66 (2H, d, J = 8.0 Hz), 7.99 (2H, d, 8.4 Hz). 13C-NMR (100 MHz, CDCl3) δ 16.7, 113.6, 124.1 (q, J = 270.9 Hz), 125.6 (q, J=3.7 Hz), 126.4, 129.9 (q, J=32.0 Hz), 137.3, 153.8, 167.0. IR (KBr) ν 1327, 1109, 1068. MS (EI) m/z 275 (M+, 100%). HRMS Calcd for C11H8F3NS2: 275.0050, Found: 275.0050.
4,5-Dimethyl-2-(methylthio)thiazole (3f): Colorless oil. 1H-NMR (400 MHz, CDCl3) δ 2.28 (3H, d, J = 0.6 Hz), 2.30 (3H, d, J = 0.6 Hz), 2.62 (3H, s). 13C-NMR (100 MHz, CDCl3) δ 11.3, 14.6, 17.0, 125.9, 148.3, 160.8. IR (neat) ν 2921, 1557, 1417, 1028. MS (EI) m/z 159 (M+, 100%). HRMS Calcd for C6H9NS2: 159.0165, Found: 159.0176.
5-(2-Methoxyethyl)-4-methyl-2-(methylthio)thiazole (3g): Colorless oil. 1H-NMR (400 MHz, CDCl3) δ 2.31 (3H, s), 2.64 (3H, s), 2.94 (2H, t, J = 6.4 Hz), 3.37 (3H, s), 3.53 (2H, t, J = 6.8 Hz). 13C-NMR (100 MHz, CDCl3) δ 14.9, 16.8, 27.1, 58.7, 72.4, 127.8, 148.6, 162.2. IR (neat) ν 2925, 1415, 1118. MS (EI) m/z 203 (M+, 52%), 158 (M+-45, 100%). HRMS Calcd for C8H13NS2: 203.0439, Found: 203.0427.
4-Methyl-2-(methylthio)-5-vinylthiazole (3h): Colorless oil. 1H-NMR (400 MHz, CDCl3) δ 2.37 (3H, s), 2.66 (3H, s), 5.16 (1H, d, J = 10.8 Hz), 5.28 (1H, d, J = 16.8 Hz), 6.71 (1H, dd, J=17.2, 10.8 Hz). 13C-NMR (100 MHz, CDCl3) δ 15.2, 16.5, 114.7, 126.4, 130.6, 149.9, 163.4. IR (neat) ν 2925, 1617, 1413, 1374, 1310, 1078, 1024, 969, 891. MS (EI) m/z 225 (M+, 100%), 210 (M+-CH3, 32%). HRMS Calcd for C7H9NS2: 171.0176, Found: 171.0165.
2-(Methylthio)-5-(trismethylsilyl)thiazole (3i): Colorless oil. 1H-NMR (400 MHz, CDCl3) δ 0.32 (9H, s), 2.71 (3H, s), 7.63 (1H, s). 13C-NMR (100 MHz, CDCl3) δ -0.1, 17.0, 132.4, 148.3, 171.1. IR (neat) ν 2955, 1478, 1376, 1254, 1016, 842, 758. MS (EI) m/z 203 (M+, 100%), 188 (M+-CH3, 47%). HRMS Calcd for C7H13NS2Si: 203.0259, Found: 203.0257.
2-(Methylthio)-4-thiazolecarboxylic acid methyl ester (3j): Colorless crystals. Mp 82.5~83.5 ℃ (Hexane). Lit.14 88.0-89.0 °C. 1H-NMR (400 MHz, CDCl3) δ 2.74 (3H, s), 3.94 (3H, s), 8.05 (3H, s). 13C-NMR (100 MHz, CDCl3) δ 16.8, 52.4, 126.8, 146.8, 161.4, 168.1. IR (KBr) ν 3111, 1704, 1494, 1321, 1242, 1055, 981, 776, 766. MS (EI) m/z 189 (M, 96%), 157 (M+-CH3OH, 32%), 157 (M+-HCO2Me, 32%). HRMS Calcd for C6H7NO2S2: 188.9918, Found: 188.9902.
2-(Methylthio)benzothiazole (2)15: Colorless oil. H-NMR (500 MHz, CDCl3) δ 2.77 (s, 3H), 7.24 (td, J = 7.7, 1.2 Hz, 1H), 7.28 (td, J = 7.6, 1.1 Hz, 1H), 7.44 (d, J = 8.0 Hz, 1H), 7.61 (d, J = 7.5 Hz, 1H). 13C-NMR (125 MHz, CDCl3) δ 14.5, 109.8, 118.3, 123.8, 124.3, 142.0, 152.0, 165.7. IR (KBr) 2930, 1498, 1451, 1236, 1215, 1130, 1096, 739 cm-1. MS (EI) m/z 165 (M+, 100%), 132 (M+-SH, 63%). HRMS (EI) Calcd for C8H7NOS: 165.0248. Found: 165.0250.
4-(4-Methoxyphenyl)-2-(methylthio)oxazole (9b): Colorless crystals. Mp 54.0~56.0 ℃ (Hexane). 1H-NMR (400 MHz, CDCl3) δ 2.71 (3H, s) 3.83 (3H, s), 6.93 (2H, d, J = 8.8 Hz), 7.64 (2H, d, J = 9.2 Hz), 7.83 (1H, s). 13C-NMR (100 MHz, CDCl3) δ14.8, 155.3, 14.1, 123.5, 126.8, 133.7, 141.8, 159.5, 161.2. IR (KBr) ν 1506, 1270, 1248, 1179, 1029, 836. MS (EI) m/z 221 (M+, 100%), 206 (M+-CH3, 46%). HRMS Calcd for C11H11NO2S: 221.0510. Found: 221.0507.
2-(Methylthio)-4-phenyloxazole (9a): Yellow oil. 1H-NMR (400 MHz, CDCl3) δ 2.71 (3H, s), 7.31 (2H, t, J = 8.4 Hz), 7.39 (2H, t, J = 7.6 Hz), 7.71 (2H, d, J = 6.8 Hz), 7.90 (1H, s). 13C-NMR (100 MHz, CDCl3) δ14.8, 125.4, 128.1, 128.7, 130.7, 134.7, 142.0, 161.5. IR (neat) ν 3148, 1504, 1447, 1258, 1064, 936, 734, 693. MS (EI) m/z 191 (M+, 100%), 176 (M+-CH3, 26%). HRMS Calcd for C10H9NOS: 191.0387, Found: 191.0405.
4-(4-Methylphenyl)-2-(methylthio)oxazole (9c): Colorless crystals. Mp 38.0~39.0 ℃ (Hexane). 1H-NMR (400 MHz, CDCl3) δ 2.37 (3H, s), 2.71 (3H, s), 7.20 (2H, d, J = 8.0 Hz), 7.60 (2H, d, J = 8.0 Hz), 7.87 (1H, s). 13C-NMR (100 MHz, CDCl3) δ 14.8, 21.3, 125.4, 127.9, 129.4, 134.3, 137.9, 141.0, 161.2. IR (KBr) ν1506, 1255,1159, 1098, 1058, 933, 822, 751. MS (EI) m/z 205 (M+, 100%), 190 (M+-CH3, 27%). HRMS Calcd for C11H11NOS: 205.0561, Found: 205.0552.
4-(4-Chlorophenyl)-2-(methylthio)oxazole (9d): Colorless crystals. Mp 56.5~58.0 ℃ (MeOH). 1H-NMR (400 MHz, CDCl3) δ 2.70 (3H, s), 6.35 (2H, d, J = 8.8 Hz), 7.64 (2H, d, J = 8.4 Hz), 7.90 (1H, s). 13C-NMR (100 MHz, CDCl3) δ 14.7, 126.7, 128.9, 129.3, 133.7, 134.8, 141.0, 161.8. IR (KBr) ν 1498, 1485, 1253, 1169, 1099, 1086, 1063, 933, 845, 828. MS (EI) m/z 225 (M+, 100%), 210 (M+-CH3, 32%). HRMS Calcd for C10H8ClNOS: 225.0015, Found: 225.0021.
4-(4-Cyanophenyl)-2-(methylthio)oxazole (9e): Colorless crystals. Mp 112.5~113.5 ℃ (MeOH). 1H-NMR (400 MHz, CDCl3) δ 2.72 (3H, s), 7.68 (2H, d, J = 8.4 Hz), 7.83 (2H, d, J = 8.4 Hz), 8.01 (1H, s). 13C-NMR (100 MHz, CDCl3) δ 14.8, 111.4, 118.8, 125.9, 132.6, 135.2, 136.3, 140.5, 162.6. IR (KBr) ν 3105, 2223, 1612, 1500, 1063, 935, 845. MS (EI) m/z 216 (M+, 100%), 201 (M+-CH3, 15%). HRMS Calcd for C11H8N2OS: 216.0357, Found: 216.0341.
2-(Methylthio)-4,5-diphenyloxazole (9f): Colorless crystals. Mp 63.0~64.5 ℃ (EtOH). 1H-NMR (400 MHz, CDCl3) δ 2.73 (3H, s), 7.29~7.40 (6H, m), 7.56 (2H, dd, J = 8.4, 1.6 Hz), 7.65 (2H, dd, J = 8.4, 2.0 Hz). 13C-NMR (100 MHz, CDCl3) δ 14.7, 126.2, 127.9, 128.2, 128.4, 128.5, 128.6, 128.7, 132.2, 136.5, 147.0, 159.9. IR (KBr) ν 1507, 1496, 1204, 764, 697. MS (EI) m/z 267 (M+, 100%), 252 (M+-CH3, 14%). HRMS Calcd for C16H13NOS: 267.0718, Found: 267.0724.
4-(t-Butyl)-2-(methylthio)oxazole (9g): Colorless oil. 1H-NMR (400 MHz, CDCl3) δ 1.24 (9H, s), 2.62 (3H, s), 7.31 (1H, s). 13C-NMR (100 MHz, CDCl3) δ 14.7, 29.0, 31.0, 133.1, 152.0, 159.8. IR (KBr) ν 2961, 1504, 1278, 1141, 1076, 966, 656. MS (EI) m/z 171.1 (M+, 79%), 156 (M+-CH3, 100%). HRMS Calcd for C8H13NOS: 171.0718, Found: 171.0714.
4-Butyl-2-(methylthio)-5-pentylthiazole (9h): Colorless oil. 1H-NMR (400 MHz, CDCl3) δ 0.90 (6H, m), 1.31 (6H, m), 1.57 (4H, m), 2.37 (2H, t, J = 8.0 Hz), 2.54 (2H, t, J = 7.6 Hz), 2.60 (3H, s). 13C-NMR (100 MHz, CDCl3) δ 13.7, 14.0, 14.7, 22.1, 22.4, 24.3, 25.7, 28.8, 30.5, 31.5, 136.1, 148.7, 157.3. IR (KBr) ν 2930, 2860, 1505, 1466, 1190. MS (EI) m/z 241 (M+, 100%), 226 (M+-CH3, 20%). HRMS Calcd for C13H23NOS: 241.1500, Found: 241.1483.
ACKNOWLEDGEMENTS
This work was supported by a Grant-in-Aid for Scientific Research (No. 21229001), and Platform for Drug Discovery, Informatics, and Structural Life Science from the Ministry of Education, Culture, Sports, Science and Technology, Japan. M. A. expresses her appreciation to the financial supports from the Grant-in-Aid for Scientific Research (No. 22689001 and 25109503), and also to the Asahi Glass Foundation.
References
1. For examples, a) N. S. Kumar and R. N. Young, Bioorg. Med. Chem., 2009, 17, 5388; CrossRef b) K. C. Nicolaou, P. K. Sasmal, G. Rassias, M. V. Reddy, K.-H. Altmann, M. Wartmann, A. O’Brate, and P. Giannakakou, Angew. Chem. Int. Ed., 2003, 42, 3515; CrossRef c) W. Du, C. Hardouin, H. Cheng, I. Hwang, and D. L. Boger, Bioorg. Med. Chem. Lett., 2005, 15, 103; CrossRef d) G. S. Jayatilake and B. J. Baker, Org. Lett., 1999, 1, 661. CrossRef
2. For examples, a) I. M. Yonova, C. A. Osborne, N. S. Morrissette, and E. R. Jarvo, J. Org. Chem., 2014, 79, 1947; CrossRef b) C. Dunst and P. Knochel, J. Org. Chem., 2011, 76, 6972; CrossRef c) N. Park, K. Park, M. Jang, and S. Lee, J. Org. Chem., 2011, 76, 4371; CrossRef d) D. R. Williams and L. Fu, Org. Lett., 2010, 12, 808; CrossRef e) K. Lee, C. M. Counceller, and J. P. Stambuli, Org. Lett., 2009, 11, 1457; CrossRef f) S. M. Riyadh, H. Ishii, and T. Fuchigami, Tetrahedron, 2002, 58, 9273. CrossRef
3. For examples, a) S. Tani, T. N. Uehara, J. Yamaguchi, and K. Itami, Chem. Sci., 2014, 5, 123; CrossRef b) B. Liu, J. Li, F. Song, and J. You, Chem. Eur. J., 2012, 18, 10830; CrossRef c) T. Yamamoto, K. Muto, M. Komiyama, J. Canivet, J. Yamaguchi, and K. Itami, Chem. Eur. J., 2011, 17, 10113; CrossRef d) F. Yang, Z. Xu, Z. Wang, Z. Yu, and R. Wang, Chem. Eur. J., 2011, 17, 6321; CrossRef e) N. S. Nandurkar, M. J. Bhanushali, M. D. Bhor, and B. M. Bhanage, Tetrahedron Lett., 2008, 49, 1045; CrossRef f) H.-Q. Do and O. Daugulis, J. Am. Chem. Soc., 2007, 129, 12404. CrossRef
4. a) Z. He, F. Luo, Y. Li, and G. Zhu, Tetrahedron Lett., 2013, 54, 5907; CrossRef b) C. Dai, Z. Xu, F. Huang, Z. Yu, and Y.-F. Gao, J. Org. Chem., 2012, 77, 4414; CrossRef c) A.-X. Zhou, X.-Y. Liu, K. Yang, S.-C. Zhao, and Y.-M. Liang, Org. Biomol. Chem., 2011, 9, 5456. CrossRef
5. a) A. R. Rosario, K. K. Casola, C. E. S. Oliveira, and G. Zeni, Adv. Synth. Catal., 2013, 355, 2960; CrossRef b) H. Inomata, A. Toh, T. Mitsui, and S. Fukuzawa, Tetrahedron Lett., 2013, 54, 4729; CrossRef c) S. Fukuzawa, E. Shimizu, Y. Atsuumi, M. Haga, and K. Ogata, Tetrahedron Lett., 2009, 50, 2374. CrossRef
6. a) L.-H. Zou, J. Reball, J. Mottweiler, and C. Bolm, Chem. Commun., 2012, 48, 11307; CrossRef b) I. Popov, H.-Q. Do, and O. Daugulis, J. Org. Chem., 2009, 74, 8309. CrossRef
7. S. Ranjit, R. Lee, D. Heryadi, C. Shen, J.-E. Wu, P. Zhang, K.-W. Huang, and X. Liu, J. Org. Chem., 2011, 76, 8999. CrossRef
8. Review: M. Arisawa, Tetrahedron Lett., 2014, 55, 3391. CrossRef
9. a) M. Arisawa, Y. Nihei, and M. Yamaguchi, Tetrahedron Lett., 2012, 53, 5729; CrossRef b) M. Arisawa, K. Fujimoto, S. Morinaka, and M. Yamaguchi, J. Am. Chem. Soc., 2005, 127, 12226; CrossRef c) M. Arisawa, F. Toriyama, and M. Yamaguchi, Heteroatom. Chem., 2011, 22, 18; CrossRef d) M. Arisawa, F. Toriyama, and M. Yamaguchi, Tetrahedron, 2011, 67, 2305; CrossRef e) M. Arisawa, F. Toriyama, and M. Yamaguchi, Tetrahedron Lett., 2011, 52, 2344. CrossRef
10. a) K. Shen, Y. Fu, J.-N. Li, L. Liu, and Q.-X. Guo, Tetrahedron, 2007, 63, 1568; CrossRef b) F. G. Bordwell, Acc. Chem. Res., 1988, 21, 456. CrossRef
11. V. K. Ahluwalia, K. K. Arora, and G. Kaur, Synth. Commun., 1987, 17, 1441. CrossRef
12. R. J. Abraham and M. Reid, J. Chem. Soc., Perkin Trans. 2, 2002, 1081. CrossRef
13. G. Vernin, C. Siv, and J. Metzger, J. Heterocycl. Chem., 1978, 15, 1361. CrossRef
14. R. Gompper and U. Heinemann, Angew. Chem., 1981, 93, 297. CrossRef
15. C. Bauder, Eur. J. Org. Chem., 2010, 6207. CrossRef