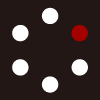
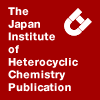
HETEROCYCLES
An International Journal for Reviews and Communications in Heterocyclic ChemistryWeb Edition ISSN: 1881-0942
Published online by The Japan Institute of Heterocyclic Chemistry
e-Journal
Full Text HTML
Received, 30th June, 2014, Accepted, 9th October, 2014, Published online, 17th October, 2014.
DOI: 10.3987/COM-14-S(K)69
■ Comparison of Photochemical Reactions of Aromatic Carbonyl Compounds with a Silyl Ketene Thioacetal and a Silyl Ketene Acetal
Gyeong Min Moon, Suk Hyun Lim, Dae Won Cho,* Sung Hong Kim, In Ok Lee, Ung Chan Yoon, and Patrick S. Mariano*
Department of Chemistry and Chemical Biology, University of New Mexico, Albuequerque, NM 87131, U.S.A.
Abstract
Photoaddition reactions of carbonyl compounds with silyl ketene thioacetals have been explored and the results are compared to those arising from investigations of analogus reactions with silyl ketene acetals. Observations made in this study show that photoirradiation of benzaldehyde (8) and benzophenone (9) and with the dimethyl substituted silyl ketene thioacetal (13) promotes reactions that take place predominantly via Paterno-Büchi type [2+2]-cycloaddition pathways to produce oxetanes. In addition, photoreactions between acetophenone (10) and p-cyanoacetophenone (11) and 13 occur via competitive sequential single electron transfer (SET)-desilylation or [2+2]-cycloaddition modes. Lastly, photochemical reaction of the highly electron deficient carbonyl compound, p-trifluoromethylacetophenone (12), and 13 gives rise to exclusive formation of β-hydroxythioester arising via the SET pathway. In contrast, photochemical reactions of all of these aromatic carbonyl compounds with the analogous dimethyl substituted silyl ketene acetal 14 take place predominantly via a sequential SET-desilylation pathway to form β-hydroxyesters. A comparison of these results reveals that replacement of an alkoxy by alkylthio group in the ketene derivatives brings about dramatic changes in chemoselectivities of the photoreactions with aryl-substituted ketones.INTRODUCTION
Previous studies have demonstrated that photochemical ground state reactions of α-trialkylsilyl electron donors taking place through sequential single electron transfer (SET) - desilylation mechanistic pathways (Scheme 1) serve as highly efficient and regioselective methods to generate α-carbon radicals.1-3 Specifically, radical cations generated by SET from α-trialkylsilyl donors to excited states of acceptors or ground states of oxidants typically undergo rapid solvent-assisted desilylation to produce α-donor substituted carbon center free radicals.4-8
The results of a number of studies have demonstrated that Lewis acid catalyzed Claisen/Mukaiyama type reactions of carbonyl compounds with silyl ketene acetals and silyl enol ethers9-14 are highly useful synthetic protocols.
Recently, we explored photochemical versions of Mukaiyama type addition reactions of these types of substrates (Scheme 2).15,16 The observations made in this effort show that oxetane 5 and β-hydroxyketones/ β-hydroxyesters 6 are produced by respective sequential [2+2]-cycloaddition and SET-desilylation pathways,17,18 in a manner that is mainly dependent on electron donating ability (i.e., oxidation potential) of the silyl ketene acetals and silyl enol ethers and solvent polarity.15,16,19 Specifically, when electron rich α-silyl donors and relatively polar solvents (i.e., MeCN) are used, photoreactions with aromatic carbonyl compounds occur to form β-hydroxyketone/ β-hydroxyesters 6 predominantly. However, oxetanes 5 are dominant photoproducts of photoreactions occurring between carbonyl compounds and electron deficient α-silyl substituted donor in less polar solvents (i.e., benzene). (Scheme 2)15,16
As a part of continuing investigations in this area, photoaddition reactions of aryl-substituted carbonyl compounds with the silyl ketene thioacetal 13 (Scheme 3) were explored. The mechanistic pathways followed in these processes, reflected in product distributions, were compared with those previously described15 for analogous photoreactions of aryl-substituted ketones with silyl ketene acetal 14 (Scheme 3). The results of this effort are described below.
RESULTS AND DISCUSSION
Photochemical reactions were carried out by using Pyrex glass filtered light (>290 nm) in independent benzene solutions (200 mL) containing the carbonyl compounds 8-12 (33 mM) and silyl ketene thioacetal 13 (66 mM) for time periods that bring about 61-77% conversions of the ketones. The concentrated photolysates were then subjected to silica gel chromatography to obtain the photoadducts, whose structures were assigned by comparing their NMR spectroscopic properties with those of structurally similar substances generated in previous studies.15,16
The product distributions arising from photoreactions occurring between ketones 8 and 9 and ketene derivative in benzene solutions are displayed in Schemes 4 and Table 1. Firstly, irradiation of a benzene solution containing benzaldehyde 8 and ketene thioacetal 13 for 3 h leads to formation of β-hydroxythioester 15a (18%), generated through a SET-desilylation mechanistic pathway, along with a mixture of the diasteromeric oxetanes 16a and 16b (50%), arising through [2+2]-cycloaddition processes. In the photoreaction of benzophenone 9 and 13, instead, both oxetane 17 (31%) and benzpinacol 1815 (40%), formed by respective [2+2]-cycloaddition and photoreduction pathways, are produced as predominant products (Scheme 4 and Table 1). These observations indicate that the excited states of carbonyl compounds 8 and 9 react with silyl ketene thioacetal 13 by a classical [2+2]-cycloaddition pathway predominantly. In addition, photoreduction of 9 is common process occurring in ketone rather than aldehyde photochemistry.15
A comparison of the product distributions of reactions between aryl-ketones 8 and 9 and silyl ketene thioacetal 13 and the corresponding acetal 14 reveals the existence of an interesting trend. In previous studies,15 the results of which are displayed in Scheme 5 and Table 1, it was found that photochemical reactions of benzaldehyde with silyl ketene acetal 14 gives rise to predominant formation of β-hydroxyester 19 (72%) or its silyl ether derivative 20 (14%). In addition, photoreaction of benzophenone 9 with 14 leads to generation of 21 (11%), 23 (27%) and 25 (3%), and 18 (40%), which arise from the operation of respective SET-desilylation, [2+2]-cycloaddition and photoreduction pathways. A comparison of these product distributions with those coming from reactions of the same aryl-ketones with silyl ketene thioacetal 13 clearly shows that the nature of the reaction pathways followed is governed by the electronic properties of ketene derivative. Specifically, while photoreactions of carbonyl compounds 8 and 9 with silyl ketene thioacetal [2+2]-cycloaddtion 13 take place predominantly through [2+2]-cycloaddition routes, those with the silyl ketene acetal 14 occur principally via sequential SET-desilylation pathway. Moreover, even though the absolute efficiencies of the photoreactions presented above were not determined, monitoring irradiation times vs percent conversions (Table 1) shows that the silyl ketene thioacetal 13 reacts more efficiently than silyl ketene acetal analog 14 in reactions with these ketones. This reactivity trend also exists for photoaddition reaction of acetophenone derivatives 10 and 11 with silyl substituted donors 13 and 14 (see below).
The products and yields of photoreactions occurring between acetophenone derivatives 10-12 and 13 and 14 in benzene were also determined (Schemes 6 and 7,15 and Table 2). Photoreaction of actophenone 10 with silyl ketene thioacetal 13 yields pinacol 31 (38%) as the major product along with nearly equal amounts of β-hydroxythioester 26 (19%) and oxetanes 29a and 29b (20%) formed through SET-desilylation and [2+2]-cycloaddition routes, respectively. However, photoirradiation of a benzene solution containing 10 and silyl ketene acetal 14 leads to predominant production of oxetane 34 (64%), which is readily converted to β-hydroxyester 32 by treatment with acid, along with oxetanes 35a and 35b (11%) and pinacol 3115 (3%).
When the strong electron withdrawing cyano group resides at the para-position of acetophenone, as in 11, the photoreduction process forming 31 that is prevalent in the reaction of 10 is completely eliminated. Specifically, SET-desilylation and [2+2]-cycloaddition processes take place competitively in the photoreaction between 11 and 13 to form β-hydroxythioester 27 (24%) and oxetane 30 (36%). Compared to that between 11 and 13, photochemical reaction of 11 and 14 in benzene gives rise to formation of chiefly β-hydroxyesters 32 and 33 (80%) along with a minor amount of diol 36 (5%). Importantly, no oxetane products are generated in this process. Interestingly, the acetophenone derivative 12, which contains a strongly electron withdrawing trifluoromethyl group at the para-position of the aryl ring, undergoes photoreaction with the silyl ketene thioacetal 13 by way of an SET-desilylayion pathway exclusively to produce β-hydroxythioester 28 (52%).
Observations made in the studies described above provide qualitative information about the mechanisms and efficiencies of photoreactions occurring between aryl-ketones and substrates 13 and 14. A comparison of photoproduct distributions generated in these processes show that replacement of alkylthio by an alkoxy group in the ketene derivative causes a dramatic change in the major excited state reaction pathway followed. While photochemical reactions of the aryl-substituted ketones with silyl ketene acetal 14 take place predominantly through a sequential SET-desilylation route, reactions with silyl ketene thioacetal 13 occur by a classical [2+2]-cycloaddition pathway preferentially. Information about the source of this difference is found in observations made in our earlier studies16 of photoreactions of carbonyl compounds with variously methyl substituted silyl ketene acetals. In that effort, we observed that oxetane formation via a [2+2]-cycloaddtion pathway becomes more dominant than the sequential SET-desilylation process in photoreactions of aryl-ketones with less methyl-substituted silyl ketene acetals, such as CH2=C(OSiMe3)(OMe), in nonpolar solvents like benzene. We attributed this trend to the less favorable thermodynamics (ΔGoSET)16 and thus rates of SET to the triplet excited states of the aryl-ketones caused by removal of electron donor methyl groups from the silyl ketene acetal skeleton. The source of the different mechanistic behavior of reactions of 13 and 14 with the excited state of aryl-ketones might also be related to SET thermodynamics. For example, the change from reactions of 13 occurring predominantly via [2+2]-cycloaddition to SET-desilylation brought about by electron withdrawing group substitution on the acetophenone ring system is likely a consequence of an increase in the excited state reduction potential of the aryl-ketone substrate which makes SET from 13 more thermodynamically favorable. Although further studies are necessary in order to gain more convincing evidence for this conclusion, a possibility exists that the classical [2+2]-cycloaddtion, oxetane forming pathway is more prominent in photochemical reactions of the silyl ketene thioacetal 13 than its acetal analog 14 is a result of the higher oxidation potential of 13 which would cause SET to the aryl-ketone triplets sates to be less thermodynamically favored. Further studies are underway to gain more clear insight into the factors that govern the chemoselectivities of photochemical reactions of silyl ketene acetals and related thioacetal with carbonyl compounds.
EXPERIMENTAL
General Procedure 1H and 13C NMR (200 MHz) spectra were recorded using CDCl3 solutions and chemical shifts are reported in parts per million relative to CHCl3 peak (7.24 ppm for 1H NMR and 77.0 ppm for 13C NMR) as an internal standard. Preparative photochemical reactions were conducted using an apparatus consisting of a 450 W Hanovia medium vapor pressure mercury lamp surrounded by a Uranium glass filter in a water-cooled quartz immersion well surrounded by the solution being irradiated. The photolysis solutions were purged with nitrogen before and during irradiation. The photolysates were concentrated under reduced pressure giving residues, which were subjected to silica gel column chromatography. All of carbonyl substrates used in the photoreactions derived from commercial sources and silyl ketene thioacetal 13 was prepared by using a well-known synthetic procedure.20 All new compounds characterized in this study were isolated as oils in >90% purity (by NMR analysis) unless noted otherwise.
Irradiation of a benzene solution containing benzaldehyde 8 and thioacetal 13. A solution of benzaldehyde 8 (640 mg, 6 mmol) and dimethyl substituted silyl ketene thioacetal 13 (2.6 g, 12 mmol) in 180 mL of benzene was irradiated for 3 h (ca. 75% conversion of 8). Concentration of photolysate gave a residue, which was subjected to silica gel column chromatography (EtOAc: n-hexane = 1: 40) giving 206 mg (18%) of 15a, 581 mg (39%) of 16a and 149 mg (11 %) of 16b.
15a: 1H NMR (CDCl3) 0.97 (t, 3H, J = 7.3 Hz), 1.12 (s, 3H), 1.22 (s, 3H), 1.55-1.66 (m, 2H), 2.86 (t, 3H, J = 7.3 Hz), 4.94 (s, 1H), 7.20-7.34 (m, 5H); 13C-NMR 13.3, 17.5, 18.9, 22.6, 30.6, 54.3, 78.6, 127.5, 127.6, 128.2, 139.8, 207.8; IR (neat, cm-1) 3500 (br, OH), 1670 (C=O); HRMS (FAB) m/z 253.1262 (C14H21O2S requires 253.1262).
16a: 1H-NMR (CDCl3) -0.06 (s, 9H), 1.03 (t, 3H, J = 7.3 Hz), 1.42 (s, 3H), 1.67 (s, 3H), 1.49-1.75 (m, 2H), 2.67 (t, 3H, J = 7.3 Hz), 5.67 (s, 1H), 7.33-7.36 (m, 5H); 13C-NMR 1.4, 13.8, 22.5, 25.0, 26.4, 30.5, 89.4, 89.5, 91.7, 127.3, 127.5, 137.8; HRMS (FAB) m/z 325.1657 (C17H29O2SSi requires 325.1658).
16b: 1H-NMR (CDCl3) 0.27 (s, 9H), 0.77 (t, 3H, J = 7.3 Hz), 1.65 (s, 3H), 1.69 (s, 3H), 1.50-1.76 (m, 2H), 2.65 (t, 3H, J = 7.3 Hz), 5.61 (s, 1H), 7.25-7.48 (m, 5H); 13C-NMR 1.8, 13.8, 22.2, 24.6, 25.6, 29.5, 89.7, 90.7, 91.9, 128.2, 128.6, 137.4; HRMS (FAB) m/z 325.1655 (C17H29O2SSi requires 325.1658).
Irradiation of benzene solution containing benzophenone 9 and thioacetal 13. A solution of benzophenone 9 (1.1 g, 6 mmol) and dimethyl substituted silyl ketene thioacetal 13 (2.6 g, 12 mmol) in 180 mL of benzene was irradiated for 2.5 h (ca. 73% conversion of 9). Concentration of photolysate gave a residue, which was subjected to silica gel column chromatography (EtOAc: n-hexane = 1: 40) giving trace of 15, 550 mg (31%) of 17 and 333 mg (40%) of 18.
15b: 1H NMR (CDCl3) 0.94 (t, 3H, J = 7.3 Hz), 1.12 (s, 3H), 1.22 (s, 3H), 1.55-1.66 (m, 2H), 2.86 (t, 3H, J = 7.3 Hz), 4.94 (s, 1H), 7.20-7.34 (m, 5H); 13C-NMR 13.3, 17.5, 18.9, 22.6, 30.6, 54.3, 78.6, 127.5, 127.6, 128.2, 139.8, 207.8; IR (neat, cm-1) 3500 (br, OH), 1670 (C=O); HRMS (FAB) m/z 253.1262 (C14H21O2S requires 253.1262).
17: 1H-NMR (CDCl3) 0.01 (s, 9H), 0.67 (t, 3H, J = 7.3 Hz), 1.17 (s, 3H), 1.62 (s, 3H), 1.41-1.70 (m, 2H), 2.26 (t, 2H, J = 7.3 Hz), 7.16-7.64 (m, 5H); 13C-NMR 1.8, 13.7, 21.9, 25.4, 26.0, 29.6, 88.8, 94.1, 95.0, 126.4, 126.6, 127.1, 128.0, 142.5, 144.1; HRMS (FAB) m/z 401.1956 (C23H33O2SSi requires 401.1971).
Irradiation of a benzene solution containing acetophenone 10 and thioacetal 13. A solution of acetophenone 10 (700 mg, 6 mmol) and dimethyl substituted silyl ketene thioacetal 13 (2.6 g, 12 mmol) in 180 mL of benzene was irradiated for 4 h (ca. 71% conversion of 10). Concentration of photolysate gave a residue, which was subjected to silica gel column chromatography (EtOAc: n-hexane = 1: 40) giving 320 mg (19%) of 26, 243 mg (11%) of 29a, 199 mg (9%) of 29b and 150 mg (38%) of 31.
26: 1H-NMR (CDCl3) 0.95 (t, 3H, J = 7.3 Hz), 1.19 (s, 3H), 1.17 (s, 3H), 1.40-1.60 (m, 2H), 1.60 (s, 3H), 2.81 (t, 3H, J = 7.3 Hz), 4.75 (s, 1H), 7.22-7.46 (m, 5H); 13C-NMR 13.3, 21.7, 21.9, 22.4, 24.9, 30.9, 56.0, 77.6, 126.7, 127.1, 127.2, 143.3, 210.6; IR (neat, cm-1) 3470 (br, OH), 1640 (C=O); HRMS (FAB) m/z 267.1419 (C15H23O2S requires 267.1419).
29a: 1H-NMR (CDCl3) -0.04 (s, 9H), 1.07 (t, 3H, J = 7.3 Hz), 1.24-1.30 (m, 2H), 1.57 (s, 3H), 1.67 (s, 3H), 1.79 (s, 3H), 2.67 (t, 2H, , J = 7.3 Hz), 7.27-7.33 (m, 5H); 13C-NMR 1.65, 13.8, 22.6, 26.1, 26.6, 27.2, 30.5, 88.4, 92.5, 93.0, 125.7, 126.1, 127.0, 144.5; HRMS (FAB) m/z 339.1814 (C18H31O2SSi requires 339.1814).
29b: 1H-NMR (CDCl3) 0.33 (s, 9H), 0.81 (t, 3H, J = 7.3 Hz), 1.24-1.30 (m, 2H), 1.51 (s, 3H), 1.57 (s, 3H), 1.70 (s, 3H), 2.81 (t, 3H, J = 7.3 Hz), 7.25-7.48 (m, 5H); 13C-NMR 2.1, 13.9, 22.4, 26.1, 26.7, 27.3, 29.6, 89.6, 90.6, 92.9, 125.4, 126.9, 127.3, 144.2; HRMS (FAB) m/z 339.1816 (C18H31O2SSi requires 339.1814).
Irradiation of benzene solution containing p-cyanoacetophenone 11 and thioacetal 13. A solution of p-cyanoacetophenone 11 (870 mg, 6 mmol) and dimethyl substituted silyl ketene thioacetal 13 (2.6 g, 12 mmol) in 180 mL of benzene was irradiated for 2 h (ca. 61% conversion of 11). Concentration of photolysate gave a residue, which was subjected to silica gel column chromatography (EtOAc: n-hexane = 1: 40) giving 256 mg (24%) of 27 and 474 mg (36%) of 30.
27: 1H-NMR (CDCl3) 0.95 (t, 3H, J = 7.3 Hz), 1.16 (s, 3H), 1.22 (s, 3H), 1.42 (s, 3H), 1.54-1.64 (m, 2H), 2.81 (t, 2H, J = 7.3 Hz, 6.5 Hz), 4.75 (s, 1H), 7.52-7.62 (m, 5H); 13C-NMR 13.4, 21.7, 21.9, 22.5, 24.8, 31.1, 55.8, 77.9, 110.9, 118.9, 128.2, 131.2, 148.9, 210.5; HRMS (FAB) m/z 292.1373 (M+1, C16H22NO2S requires 292.1371).
30: 1H-NMR (CDCl3) 0.32 (s, 9H), 0.81 (t, 3H, J = 7.3 Hz), 1.49 (s, 3H), 1.53 (s, 3H), 1.50-1.60 (m, 2H), 1.66 (s, 3H), 2.24 (t, 2H, J = 7.3 Hz), 7.56-7.63 (m, 5H); 13C-NMR 2.2, 13.8, 22.5, 26.6, 27.0, 27.4, 30.8, 77.2, 90.6, 92.4, 110.9, 119.1, 126.2, 131.1, 149.7; HRMS (FAB) m/z 364.1769 (M+1, C19H30NO2SSi requires 364.1767).
Irradiation of a benzene solution containing p-trifluoromethylacetophenone 12 and thioacetal 13. A solution of p-trifluoromethylacetophenone 12 (790 mg, 6 mmol) and dimethyl substituted silyl ketene thioacetal 13 (2.6 g, 12 mmol) in 180 mL of benzene was irradiated for 1.5 h (ca. 77% conversion of 12). Concentration of photolysate gave a residue, which was subjected to silica gel column chromatography (EtOAc: n-hexane = 1: 40) giving 560 mg (52%) of 28. 1H-NMR (CDCl3) 0.94 (t, 3H, J = 7.3 Hz), 1.18 (s, 3H), 1.24 (s, 3H), 1.61 (s, 3H), 1.53-1.63 (m, 2H), 2.81 (t, 3H, J = 7.3 Hz), 4.71 (s, 1H), 7.56 (s, 5H); 13C-NMR 13.3, 21.7, 21.9, 22.4, 24.9, 31.1, 55.9, 77.8, 124.1, 124.2, 127.8, 128.0, 147.5, 210.6; HRMS (FAB) m/z 335.1296 (M+1, C16H22F3O2S requires 335.1293).
ACKNOWLEDGEMENTS
We deeply congratulate the 77th birthday of professor Isao Kuwajima and this study is dedicated to professor Isao Kuwajima. This study was supported by 2011 Yeungnam University Research Grant (211A356004 for DWCho) and National Research Foundation of Korea Grant funded by the Korean Government (2012R1A1A2007158 for UCYoon).
References
1. J. Yoshida, T. Maekawa, T. Murata, S. Matsunaya, and S. Isoe, J. Am. Chem. Soc., 1990, 112, 1962; CrossRef J. Yoshida, S. Matsunaga, T. Murata, and S. Isoe, Tetrahedron, 1991, 47, 615. CrossRef
2. B. E. Cooper and W. J. Owen, J. Organomet. Chem., 1971, 29, 33; CrossRef C. G. Pitt, J. Organomet. Chem., 1973, 61, 49; CrossRef X. M. Zang, S.-R. Yeh, S. Hong, M. Freccero, A. Albini, D. E. Falvey, and P. S. Mariano, J. Am. Chem. Soc., 1994, 116, 4211. CrossRef
3. Z. Su, P. S. Mariano, D. E. Falvey, U. C. Yoon, and S. W. Oh, J. Am. Chem. Soc., 1998, 120, 10676; CrossRef D. W. Cho, U. C. Yoon, and P. S. Mariano, Acc. Chem. Res., 2011, 44, 204. CrossRef
4. T. Hirao, C. Morimoto, T. Takada, and H. Sakurai, Tetrahedron, 2001, 57, 5073; CrossRef T. Maruyama, Y. Mizuno, I. Shimizu, S. Suga, and J. Yoshida, J. Am. Chem. Soc., 2007, 129, 1902. CrossRef
5. . CrossRef
6. P. J. Jervis, B. M. Kariuki, and L. R. Cox, Tetrahedron Lett., 2008, 49, 2514; CrossRef N. Shindoh, H. Tokuyama, and K. Takasu, Tetrahedron Lett., 2007, 48, 4749; CrossRef Y. Huang and K. D. Moeller, Tetrahedron, 2006, 62, 6536. CrossRef
7. N. Asao, C. S. Chan, K. Takahashi, and Y. Yamamoto, Tetrahedron, 2005, 61, 11322; CrossRef T. K. Sarkar, A. Hazra, P. Gangopadhyay, N. Panda, Z. Slanina, C.-C. Lin, and H.-T. Chen, Tetrahedron, 2005, 61, 1155; CrossRef C. Fernandez-Rivas, M. Mendez, and A. M. Echavarren, J. Am. Chem. Soc., 2000, 122, 1221; CrossRef H. Huang and J. S. Panek, Org. Lett., 2003, 5, 1991. CrossRef
8. W.-D. Z. Li, and J.-H. Yang, Org. Lett., 2004, 6, 1849; CrossRef J. Beignet, J. Tiernan, C. H. Woo, B. M. Kariuki, and L. R. Cox, J. Org. Chem., 2004, 69, 6341; CrossRef W. R. Judd, S. Ban, and J. Aube, J. Am. Chem. Soc., 2006, 128, 13736; CrossRef L. Chabaud, P. James, and Y. Landais, Eur. J. Org. Chem., 2004, 15, 3173; CrossRef J. D. Huber, N. R. Perl, and J. L. Leighton, Angew. Chem. Int. Ed., 2008, 120, 3079. CrossRef
9. T. Sato, Y. Wakahara, J. Otera, H. Nozaki, and S. Fukuzumi, J. Am. Chem. Soc., 1991, 113, 4028; CrossRef S. Fukuzumi, T. Suenobu, M. Fujitsuka, O. Ito, T. Tonoi, S. Matsumoto, and K. Mikami, J. Organomet. Chem., 1999, 574, 32; CrossRef S. Fukuzumi, M. Fujita, J. Otera, and Y. Fujita, J. Am. Chem. Soc., 1992, 114, 10271; CrossRef S. Fukuzumi and M. Fujita, J. Org. Chem., 1993, 58, 5405. CrossRef
10. M. Abe, M. Ikeda, Y. Shirodai, and M. Nojima, Tetrahedron Lett., 1996, 37, 5901; CrossRef K. Mizuno, N. Takahashi, T. Nishiyama, and H. Inoue, Tetrahedron Lett., 1995, 36, 7463; CrossRef S. Rotzoll, E. Ullah, C. Fischer, D. Michalik, A. Spannenberg, and P. Langer, Tetrahedron, 2006, 62, 12084. CrossRef
11. N. N. Saha, V. N. Desai, and D. D. Dhavale, Tetrahedron, 2001, 57, 39. CrossRef
12. T. Mukaiyama, K. Banno, and K. Narasaka, J. Am. Chem. Soc., 1974, 96, 7503; CrossRef T. Mukaiyama, Org. React., 1982, 28, 203; T. Mukaiyama, S. Kobayashi, M. Tamura, and Y. Sagawa, Chem. Lett., 1987, 3, 491. CrossRef
13. N. Giuseppone, P. Van De Weghe, M. Mellah, and J. Collin, Tetrahedron, 1998, 54, 13129. CrossRef
14. A. Iida, S. Nakazawa, T. Okabayashi, A. Horii, T. Misaki, and Y. Tanabe, Org. Lett., 2006, 8, 5215; CrossRef A. Iida, J. Osada, R. Nagase, T. Misaki, and Y. Tanabe, Org. Lett., 2007, 9, 1859. CrossRef
15. U. C. Yoon, M. J. Kim, J. J. Moon, S. W. Oh, H. J. Kim, and P. S. Mariano, Bull. Korean Chem. Soc., 2002, 23, 1218. CrossRef
16. D. W. Cho, H.-Y. Lee, S. W. Oh, J. H. Choi, H. J. Park, P. S. Mariano, and U. C. Yoon, J. Org. Chem., 2008, 73, 4539; CrossRef S. W. Oh, J. Y. Kim, D. W. Cho, J. H. Choi, and U. C. Yoon, Bull. Korean Chem. Soc., 2007, 28, 629; CrossRef H. J. Park, U. C. Yoon, H. -Y. Lee, D. W. Cho, and P. S. Mariano, J. Org. Chem., 2012, 77, 10304. CrossRef
17. G. Jones, ‘Organic photochemistry’ Vol. 5, ed. by A. Padwa, Marcel Dekker, New York, 1981, Chapter I; A. G. Griesbeck, M. Fiege, S. Bondock, and M. S. Gudipati, Org. Lett., 2002, 2, 3623. CrossRef
18. W. Adam and V. R. Stegmann, Synthesis, 2001, 1203; CrossRef G. Büchi, C. G. Inman, and E. S. Lipinsky, J. Am. Chem. Soc., 1954, 76, 4327; CrossRef T. Bach, Synthesis, 1998, 683. CrossRef
19. M. Abe, Y. Shirodai, and M. Nojima, J. Chem. Soc., Perkin Trans. 1, 1998, 3253. CrossRef
20. Y. Kita, N. Shibata, T. Tohjo, and N. Yoshida, J. Chem. Soc., Perkin Trans. 1, 1992, 1795. CrossRef