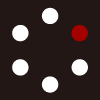
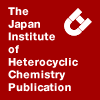
HETEROCYCLES
An International Journal for Reviews and Communications in Heterocyclic ChemistryWeb Edition ISSN: 1881-0942
Published online by The Japan Institute of Heterocyclic Chemistry
e-Journal
Full Text HTML
Received, 7th July, 2014, Accepted, 26th August, 2014, Published online, 28th August, 2014.
■ N-Ylides of 1,2,3-Triazoles and Tetrazoles – An Overview
Dietrich Moderhack*
Institute of Medicinal and Pharmaceutical Chemistry, Technical University, Braunschweig, Beethovenstrasse 55, D-38106, Germany
Abstract
This treatise surveys the preparative chemistry of the title ylides as it developed from the beginnings in the early 1980s.INTRODUCTION
Among heteroaromatic N-ylides – widely known as cycloimmonium ylides1–4 – azolium representatives constitute a major subdivision. While the first members became already known in the 1960s,1 derivatives of 1,2,3-triazoles and tetrazoles were not prepared until the 1980s. Since the ylides of these azoles did not receive adequate attention by recent reviewers,3,4 they will be featured in the present report. Structures to be dealt with are gathered in Chart 1, which exhibits four classes of 1,2,3-triazolium ylides (A–D) besides
three categories of tetrazolium ylides (E–G), moreover indicating the occurrence of a particular ylide type (α–δ; internal classification). The preparative access is outlined in Scheme 1. This graphic, however, does not display whether a given ylide constitutes an isolable material or an unstable intermediate. Such details (including the transformations of those species) will be disclosed in the chapters to the single classes.
1) N-YLIDES OF 1,2,3-TRIAZOLES
a) Class (A)
Following the classical Kröhnke route, treatment of the benzotriazolium salt (1a) with alkali carbonate produced the ylide (Aa) (Scheme 2).5 This β-type species (said to be stable in solution6) was not isolated and reacted with phenyl isocyanate and isothiocyanate to give the derivatives (Ab,c) in reasonable yield.5
Most interestingly, generation of Aa with triethylamine in the presence of benz- or p-tolualdehyde caused a 2 : 1 reaction to occur: After attack of the aldehyde on the ylide carbon the resultant adduct coupled to a second molecule of Aa to give the species (2a,b) which, through expelling 1,3-dimethylbenzotriazolium, furnished the poly-substituted 2,3-dihydrofurans (3a,b).6 – Beyond the unexpected aldehyde-mediated dimerization of Aa, this ylide is capable of undergoing cycloadditions with acetylenic esters. As this leads to the new ylide class (B), the process will be detailed in the appropriate chapter [see Section (1b)].
Much work has been spent on benzotriazolium ylides derived from the salts (1b-f) (Scheme 3).7,8 Two categories should be discerned: substrates having one phenacyl group (1b,c) and those bearing two such groups (1d-f). With 1b,c deprotonation takes place regiospecifically with formation of the ylides (Ad,e). These species were trapped with picryl chloride to afford the stable γ-type ylides (Af,g).7a By contrast, with the salts (1d-f) either side chain is susceptible to proton loss such as to give mixtures of ylides like Ah/Ah', Ai/Ai', and Aj/Aj', the composition of which is determined by the substituent at the Ar ligand. As expected, the chloro and nitro groups favour the formation of Ah and Aj, respectively, but the electron-releasing methoxy group should shift the equilibrium toward the isomeric ylide (Ai'). Yet, the authors, obviously misunderstanding the meaning of the σp-OMe constant (which was quoted without the minus sign!), failed to realize this, although their energy studies either uniformly7a,8a (AM1 method) or partly8a (PM3 method) point to the ylide (Ai') as the predominant species.9 Thus, the original ratio (75/25) of the products (Al/Al') obtained with picryl chloride7a,8a should read 25/75 instead. In a complementary (UV spectrometry-based) study of the pKa values of the two phenacyl groups of 1d-f the authors found: 9.71/10.53 (1d), 10.38/11.82 (1e), and 7.21/9.62 (1f).10 Regarding 1e, again their wrong notion of the σp-OMe constant (cf. above) led them to assign the lower value to the ylide (Ai) – an error that follows independently from the reported pKa values of 1-(4-methoxyphenacyl)-4-phenyl-4H-1,2,4-triazolium11 and 1-(4-methoxyphenacyl)pyridinium.12
Benzotriazolium salts like 1g have recently proved to be useful sources for symmetrical (E)-stilbenes (4) (Scheme 4).13 Under the influence of a strong base these salts undergo homocoupling, which commences with formation of the respective ylide (An). This species is attacked by a second molecule of 1g to give, after loss of 5, the intermediate (1h). The latter stabilizes via Hofmann elimination to provide, besides further 5, the desired olefin (4). As apparent from the list of examples prepared by this procedure, both electron-releasing and -withdrawing groups at the benzyl ligand have a detrimental effect on the yield.
While the aforementioned ylide preparations are two-step procedures that require isolation of a quaternary salt as the immediate precursor, this route is abridged in the case of the ylides (Ao,p) (Scheme 5).14 When the hydroxyquinone-substituted benzotriazole (6) was reacted with benzoyl as well as benzenesulfonyl chloride, the products of quaternization are acidic to such an extent as to deprotonate in situ once they were formed [for a similar approach to ylides of the class (E) and (G), cf. Section (2b) and (2c)].
A short entry of the 'reverse order' constitutes the direct insertion of the ylide function into an azole. This has been achieved with 1-ethyl-1,2,3-triazole and two 1-substituted benzotriazoles (Scheme 6). Treatment of these compounds with tetracyanoethylene oxide gave rise to the dicyanomethylides (Aq,r)15 and (As).17
The regioselectivity of this process is consistent with the rules that govern the quaternization of azoles; hence, an ylide of the class (B) cannot arise in this case. Whereas the procedure works also well with 1,2,4-triazoles,15 it fails with 2-methylbenzotriazole15 (and likewise tetrazoles18) because of insufficient nucleophilicity of these substrates. – For the chemical behaviour of Ar,s, see Section (1b).
b) Class (B)
Since ylides of the preceding class contain an azomethine imine unit, they were envisaged as candidates for 1,3-dipolar cycloadditions. Indeed, reactions of the derivative (Ar) with alkynes in an apolar solvent gave cycloadducts like 7a-c, according to the predicted regioselectivity (Scheme 7).19a But – apparently not recognized at first19a – these species eluded isolation: they underwent cleavage of the N–C(CN)2 bond to give members of a novel ylide class, viz. the compounds (Ba-c) [representing the δ1 type ('allylide')].19b Later, an intramolecular example of this kind of cycloaddition, i.e. As → 7d → Bd, was also reported.17
Surprisingly, cycloadducts obtained from the ylide (Aa), viz. the derivatives (8a-c), tend to ring-open in a different way: here fission of the triazole half-ring predominates (step b), followed by a new ring closure through engagement of the MeN unit and the benzoyl group to afford pyrazolo[1,5-a]quinoxalines like 9.6 Only with 8a cleavage of the pyrazole half-ring (step a) took place too (→ Be), but also here the tricycle (9a) was obtained as the major product. Extending the experiments to dimethyl butynedioate and ethyl phenylpropiolate, the analogues (9b,c) were the sole materials found. The structure of these unexpected heterocycles was confirmed by X-ray diffraction.6 – No direct access to B seems to have been reported till now, e.g., quaternization of 2H-1,2,3-triazoles (or -benzotriazoles) having an α-CH-acidic group at N(2).
c) Class (C)
The majority of cited members of this class belong to the α1 type (Chart 1). The search for these species commenced around 1990 in pursuit of previous work on the analogous N-aminides and N-oxides;20 their behaviour as 1,3-dipoles was the proper incentive. First efforts to generate C started from the N-methyl- (10a-h) and N-(silylmethyl)triazolium salts (10a',c') (Schemes 8).21,22 When the former were treated with triethylamine or butyllithium, the base removed the methyl group, but employing sodium ethoxide, proton abstration could be effected. Apart from 10h which was attacked at the six-membered ring to furnish 11,22
the salts (10a-g) reacted at the desired position to eventually give mixtures of the dihydro-1,2,4-triazines (13a-g) and the 1-aminoimidazoles (14a-g), the ratio of which depended on the substituents. Identical findings, i.e. 13a,c and 14a,c, resulted on treatment of the salts (10a',c') with cesium fluoride.22 On the other hand, the alkoxide-caused ring transformation of the phenanthrotriazolium salts (15a-e) only gave the dihydrotriazines (16a-e) (Scheme 8).23 A complementary study of the behaviour of 10a-e toward other bases including cyanide ion allowed additional insights into the formation of 13 and 14, in particular as regards the proportions (Schemes 9).24 These heterocycles, the structures of which had been confirmed by
the X-ray method,22 arose by a 6π-electrocyclic process of the triazatrienes (12a-g) (Scheme 8). The latter species were shown by ab initio 3-21G calculations to be thermodynamically preferred over the ylides (Ca-g) (the opposite applies to the related N-oxides, while the N-aminides are borderline).21 This meant: Does an ylide (C) form at all in the base-induced reactions? Considering that the trienes (12) can arise directly from 10 through a Hofmann-type degradation and, second, that interception of the ylides with 1,3-dipolarophiles failed, the occurrence of C appeared doubtful.21 Under divergent conditions, however, ylide formation did take place: (i) on decomposition of the above adducts (17), namely β-elimination of hydrogen cyanide,24 and (ii) on desilylation of the side chain of the specialized triazolium salts (10').25–28 This will be evidenced below (Scheme 10):
Treatment of the diphenyl-substituted triazolium salts (10a',b',d',e') with cesium fluoride in the presence of dimethyl/diethyl butynedioate gave the pyrrolotriazole derivatives (19a-h);25,26 accordingly, trapping of the ylide (Cb) that originated from 17b (cf. Scheme 9) led to 19b (27%).24 Side products of 19 are the above compounds (13) and (14). These materials result from ring opening of C – a reaction that could not be fully avoided, even if the dipolarophile was present before the cesium fluoride was added. Extending the alkyne-based experiments to the bicyclic ylides (Ch-k), which were generated from the salts (10i'-l'), the derivatives (19i-m) were isolated.27 All of these cycloadducts proved surprisingly stable, in striking contrast to those formed from the analogous N-oxides and N-aminides which rearrange in situ.20 Only prolonged heating in toluene effected degradation of 19; the process, however, took a different course in that the original ylide bond was retained and 1-aminopyrroles (20/21) were formed. While 19a-h extruded benzonitrile to afford 20a-h,25,26 double ring opening of the tricyclic substrates (19i-m) led to compounds like 21i-m, the side chain of which originates from the former six-membered ring.27 Of these materials, the derivatives (19e) and (20e) have been characterized by X-ray crystallography.25,26
While interception of the diphenyl-substituted ylides (Ca,b,d,e) worked well with alkynes, experiments using alkenes (namely acrylonitrile and ethyl acrylate) failed to afford the envisaged cycloadducts (22) (Scheme 11).26 Rather, starting from the triazoles (18a,b,e), a reaction cascade led to another kind of cycloadducts, viz. the pyrrolotriazines (24a-d). Here the authors observed that, quite remarkably, the in situ quaternization of 13 (to be followed by desilylation giving 23) did not take place in the absence of the dipolarophile, even if the quaternizing reagent was used in excess.
In contrast to the diphenyl-substituted ylides (Ca,b,e) the tetramethylene and dimethyl derivatives (Ch,l) could be trapped with acrylonitrile (Scheme 12). The extent of this cycloaddition, however, was modest; mixtures of the endo- (25a,b) and exo-tetrahydropyrrolotriazoles (26a,b) arose, with the former isomers predominating. Major side products were the aminoimidazoles (14h) and (14l). Employing N-substituted maleimides as dipolarophiles, the ylides (Ch-i) furnished the tricyclic adducts (27a-c), (27e), and (27g-i), respectively, while from the congener (Cl) the analogues (27d,f,j) were obtained. All of these derivatives
display exo-stereochemistry – a finding that has been rationalized via the transition state. As with the experiments using acrylonitrile, major amounts of the respective aminoimidazoles (14) were formed.28
The occurrence of β-type ylides (C) has been invoked to explain the formation of triazines (31) during base-induced reactions starting from hydrazonoyl chlorides (28) and ethyl isocyanoacetate (Scheme 13).29 The multistep process commenced with the generation of the nitrilimines (29a-f) which combined with the isocyanide to form the triazolium structures (30a-f) – according to a principle detected in the 1980s.30 Owing to the acidic side chain, rapid proton transfer led to the ylides (Cm-r) which in turn ring-expanded to the triazines (31a-f) (cf. Scheme 8). Since the latter are highly prone to cycloadd to the nitrilimine (29), the bicycles (32b-f) were obtained instead. An exception to the formation of 30 constitutes the behaviour of the initial (i.e. linear) adduct of nitrilimine (29g) and isocyanide: Due to the electron-withdrawing nitro group, ring closure by N–N bond building was severely hampered so as to lead exclusively to the oxazole (33). In the experiments with 28a-f, however, analogues of (33) were observed in minor amounts only.29
Addendum: In pursuit of the work on α1-type ylides of class (C), several analogues have been studied. These systems are shown in Chart 2; their treatment, however, falls outside the scope of this review.
d) Class (D)
These ylides might be looked at as mere derivatives of class (A), but since a number of reactions proceed with triazole ring opening (due to the fused heterocycle), system (D) stands apart. Most attention has been paid to the behaviour towards electron-poor alkynes and alkenes, showing that the results strongly depend on the conditions. When the ylides (Da-c) – generated from 34a-c – reacted with an alkyl propiolate in a polar solvent, Michael adducts like De-i arose (Scheme 14); a pyrazolotriazole analogous to 7/8 (Scheme 7)
was excluded, inter alia because of the orange colour.37 These new ylides can add another alkyne unit to form dienic ylides like Dk-n (confirmed by X-ray analysis of Dk). Hence, starting from the salts (34a,b), the derivatives (Dk-m) occurred as side products of De,f,h. But applying dimethyl butynedioate, only 1:1 adducts like Dj and Do,p were found (the mesomeric structures of Do,p were suggested by spectroscopy). Turning to the quinoline- and isoquinoline-fused ylides (Dq) and (Dr), these species (made from 35a,b) reacted with methyl propiolate to give the adducts (Ds,t) – in close analogy to the process (Dc → Di).38
Experiments in a non-polar solvent, however, took a different course. Using methyl propiolate, the ylides (Da-d) and (Dq,r) were transformed into the indolizine derivatives (36a-d)39 and (39a,b),38 respectively (Scheme 15). This conversion was believed to proceed via the diazene (37) which arose by a concerted process involving cycloaddition and triazole fission. Subsequent extrusion of molecular nitrogen led to a diradical that was stabilized to the diene (38) which in turn cyclized.39 Still more surprising did appear the
reactions with dimethyl butynedioate (Scheme 16). The ylides (Da-c) took up this alkyne in a 1:2 ratio to yield the pyrazolopyridines (40a-c).40 Of the two mechanisms the authors put forward, the alternative that suggests the cyclazine (41) as the primary intermediate is presented here; after cycloaddition (→ 42) the sequence was terminated by triazole ring opening and dehydrogenation. The peculiar structure of the products was confirmed by X-ray diffraction of the derivative (40c). A deviating route, however, was followed by the ylides (Dq,r).41 While the major reaction was simple defunctionalization to leave the parent triazoloquinoline (59%) and -isoquinoline (63%), a side process led to compounds like 43a,b. Their formation is believed to involve 44 (a species analogous to 37). But instead of extruding nitrogen, the molecule split out diazoacetophenone to give the diradical (45) which cyclized to the final product.
Reactions of thiazole-fused ylides with dipolarophilic alkynes largely follow the pattern described above. Generated from the salt (46a), the ylide (Du) was reacted with methyl propiolate to afford – besides some ether (Y) – the dienes (48), which were formed via 47 (Scheme 17).42 Previously, the existence of such dienes, i.e. 38 (cf. Scheme 15), was postulated, but the species were not isolated.39 The formation of compound (49) was negligible and could not be enforced by heating of the isolated dienes. Employing dimethyl butynedioate, only one equivalent was taken up to convert the ylides (Du,v) via 50 into 51.42 While this reaction differs from the process (Da-c → 40a-c) (cf. Scheme 16), it resembles the conversion (Dq,r → 43a,b). Changing to acetonitrile, again linear addition occurred, as exemplified with the ylide (Dv). But instead of forming an analogue of adduct (Dj) (cf. Scheme 14), a multistep dimerization process with extrusion of the bicycle (X) and pyrone ring closure occurred to give the red-coloured ylide (Dw).42
Reactions with alkenes are on the whole more complicated. When the ester-functionalized ylides (Da,b) were treated with the acrylates (52a,b), the cyclobutanes (53a-c) and the pyridylacrylates (54a,b) were obtained – apart from major amounts of the product of simple hydrolysis (55) (Scheme 18).43 As a direct precursor to 53 and 54, the diradical (56) has been proposed; it either expelled the unit (H2C=CHCOR) to yield 54 or cyclized to 53. Note that the ester group in the compounds (54) originates from the reagent, not the ylide; thus, using methyl acrylate, both Da and Db gave the compound (54a). – Partly divergent results came from the phenacylide (Dc):43 (i) Employing methyl acrylate (52a), a complex mixture arose that contained the alkene (57), the derivative (54a), and several stereoisomers of the cyclobutane (53e); also compound (57) was explained as arising via species (56); and (ii) applying ethyl methacrylate (52b), the ylide (Dc) gave the cyclobutane (53d), some pyridylacrylate (54b), and a substantial quantity of the quaternary salt (58). The formation of the latter matches Kröhnke's classical 'acid-splitting'44 (not referred to by the authors43) – a kind of conversion also tetrazolium ylides can undergo [see Section (2c)].45
Regarding reactions with electrophiles, examples are available from the ylides (De-h) (Scheme 19).46 It was found that attack took place at the end of the side chain. Thus, protonation of the substrates (De-g) gave alkenes like 59a-c, with the Z isomers predominating. Similarly, acetic anhydride (plus mineral acid) converted the substrates (De,f) into the new ylides (Dx,y); these compounds occurred exclusively in the E form. On treating the ylides (De-h) with acetic anhydride and nitric acid, the nitryl cation also replaced the terminal ester group such as to form dinitro derivatives like Dz, Daa, and Dbb. The structure of these materials was not fully recognized by means of spectroscopy, but could be established via X-ray analysis of Dz; the crystal data, however, did not point to a particular charge localization.46
Known γ-type ylides of class (D) are limited to derivatives having a dicyanomethylide group (Scheme 20). Access to representatives like Dcc,dd,47 Dee,ff,38 and Dgg41 followed the procedure applied for class (A) [see Section (1a)] and turned out to be a straightforward process. Of these stable ylides, the derivatives (Dcc) and (Ddd) gave interesting EI mass spectra: While Dcc is characterized by stepwise loss of the ylide function, molecular nitrogen, and hydrogen to afford a (2-pyridyl)vinyl ion [m/z 104 (100%)], the congener (Ddd) undergoes triazole cleavage with formation of a three-membered ring followed by loss of nitrogen and a methyl hydrogen to give the [6-(2,2-dicyanovinyl)-2-pyridyl]methyl ion [m/z 168 (91%)]. It may be added that these findings, contrary to the authors' assumption,47 do not constitute the first MS data to have been reported on cycloimmonium ylides [cf. Section (2c): Tables within Schemes 25 and 28].
Investigating the behaviour of the dicyanomethylides (Dcc-ff) toward electron-poor alkynes, it was found that Dcc,dd as well as Dee,ff gave two classes of compounds, depending on the reagent (Scheme 21). While methyl propiolate yielded indolizines like 61a,b47 and 63a,b,38 dimethyl butynedioate gave rise to quinolizines like 62a,b47,48 and 64a,b.41 Both reactions proceed via species (60) or its benzologues; note that the formation of 62a – divergent from 62b – requires irradiation.48 The route to the compounds (61) and (63) resembles the path to 36 and 39 (cf. Scheme 15); the formation of 62 and 64, however, is owing to the C(CN)2 group, if one compares the products (40) and (43) from Da-c and Dq,r and the same alkyne (cf. Scheme 16). – Experiments performed with the ylide (Dgg) did not produce identifiable materials.41
Photochemical investigations have been conducted with both the isolable ylides (Dcc-ff) and the transient congeners (Da-c,q,r) (Scheme 22).48 Not unexpectedly, most of these substrates suffered loss of the ylide
function to leave the parent heterocycles (67a,b) and (68a,b). But an interesting exception was observed with the ester-functionalized ylides (Da,b). These materials gave alkylidenehydrazones like 66a,b; their formation was rationalized to proceed via a diaziridine stage (65). Complementary photoreactions were performed in the presence of alkynes that had been used before under thermal conditions. Employing methyl propiolate, the behaviour of the two series (Da-c,q,r) and (Dcc-ff) differed in that the former did not lose the ylide group, but gave mixtures of compounds known from preceding experiments (Table 1). Reversely, using dimethyl butynedioate, the ylides (Da-c,q,r) were defunctionalized throughout, whereas the majority of the dicyano congeners, viz. the substrates (Dcc-ee), were transformed into the quinolizines (62a,b) and (64a), respectively. Of these products, the derivative (62a) was not obtained under thermal conditions (cf. preceding paragraph).48
2) N-YLIDES OF TETRAZOLES
a) Introductory studies
Prior to preparation of the first ylides of this series it has been observed that the tetrazolium salt (69a) in basic deuterium oxide underwent H/D exchange at the 3-methyl group (in addition to position 5) to afford the species (69'a), supposedly with involvement of the ylide (Ga) (Scheme 23).49 Later, a similar result was obtained with the salt (69b), whereas the 4-methyl group of the isomer (70) was found unaffected.50 These different kinetic acidities were rationalized by comparing classical resonance structures (Chart 3).51 Considering the delocalization of the anionoid lone pair in the ylides (Ea), (Fa), and (Ga), the latter ylide
is preferred energetically, since structure (Ga/iii) has both negative charges on the more electronegative nitrogen atoms, whereas Ea/iii and Fa/iii each have one formal negative charge on carbon. Moreover, the comparison reveals that the electron-withdrawing influence exerted by the three tetrazolium systems decreases in the order 1-R-tetrazolium-3-yl > 3-R-tetrazolium-1-yl > 1-R-tetrazolium-4-yl. This gradation can likewise be inferred from: (i) an inspection of INDO51 and CNDO/252 atomic charges for 1,3- and 1,4-dimethyltetrazolium cations including the ylides (Fa) and (Ga),51 and (ii) DFT calculations of Ea, Fa, and Ga (Chart 3; see columns on right).53,54 Extending these studies to phenacyltetrazolium salts (71–73), kinetic and also thermodynamic acidities were found fully in line with the above results; in addition, both kinds of data show a close correlation (Scheme 24).45
b) Class (E)
Applying the classical Kröhnke method, treatment of the tetrazolium salts (71b-k) with alkali carbonate produced the corresponding β-type ylides (Ec-l) (Scheme 25).55 Save the derivative (Ec), all compounds were obtained as solids; their stability decreases with the N-substituent becoming smaller. An essential feature that distinguishes these ylides from their analogues of class (F) and (G) is the presence of crystal water; it apparently stabilizes the negative charge, as attempts of dehydration resulted in decomposition. Also aprotic solvents are detrimental, which limits the investigation of certain spectroscopic phenomena such as the (negative) solvatochromism typical of the 'ylide band' [see Section (2c)].
Expectedly, the new ylides exhibit appreciable reactivity towards electrophiles. For practical reasons, the labile substrate (Ec) was treated in situ: joint action of (neat) acid anhydrides and excess base on the salt (71b) gave the crystalline γ-type ylides (Em) or (En); note that acetic anhydride, to a minor extent, can acylate the 5-methyl group instead of the ylide function – with the consequence of forming small amounts of pyrrolotetrazoles.55,56 In contrast to Ec, the stable ylides (Ed-l) were reacted after their isolation and, again using neat reagents, were transformed into the products (Eo-t); only the ketene thioacetal (74) was made directly from the respective salt (71g).55
Regarding the scope of the Kröhnke route, ylides could not be prepared from salts having 5-H for 5-Me (such as 71a) or having 1-phenyl for 1-alkyl (such as 71l). Substrates of this kind, instead of giving Eb or Eu, fragmented into molecular nitrogen and a carbodiimide (75) or were hydrolyzed to phenyl azide and N-phenacylacetamide (76),45 i.e. they followed reaction patterns that had previously been observed with related structures (cited in ref.45). Likewise, attempts to obtain ylides bearing bulkier groups at C(5) such as propyl and cyclohexyl remained unrewarded (cf. below).55
Complementary experiments with the salts (71) were carried out with concentrated ammonia (instead of potassium carbonate) (Scheme 26).57,58 Surprisingly, it was found that this reagent not only acts as a base, but also as a nucleophile. As a consequence, ylides (E) and/or bicycles of the type (77) were obtained, depending on the substituents. From the list of examples it is apparent that salts (71) having small R rests favour the formation of 77 (see a,i,j), regardless of the size of the R' residue. However, when the R ligand is becoming bulkier, the ylides (E) will arise (see d-h), while the yields of the bicycles (77) gradually decrease (see b-f). Ylides (E) also predominate in the case of substituted phenacyl groups (see i,j). These observations were rationalized as follows: (i) The folded geometry of 77, which was studied by the X-ray method performed with 77a, implies that sterically demanding substituents are hindered when attached to N(3), but not when residing at the bridgehead C(3a); (ii) on the other hand, ylides (E) having larger R residues or substituted phenacyl groups are sparingly soluble in water and separate once the base had been added, thereby reducing the stock of 71 for the (much slower) production of 77; the latter explains why the ylides (Ei,j) predominate, whilst, arguing sterically, the formation of 77g,h should be preferred.
In addition to the plethora of ylides (E) shown in Scheme 25, two representatives exist that distinguish from those examples either by structure and/or way of access (Scheme 27).
(i) In conjunction with the synthesis of azolium and azinium dinitromethylides the tetrazolium derivative (Ex) has been made [for an analogue of class (G), see Section (2.c)].60 The method implies a destructive nitration of the acetonyl side chain of the starting compound (71o), which occurred in a nitrating medium containing sufficient water. The two nitro groups were sequentially introduced via addition of the nitryl cation to the enolic intermediates followed by hydrolysis of the acetyl group. Later, employing substrates having an uncharged cycle, the mechanism has been studied in more detail.61 In contrast to the ylide (Ex), a 5-unsubstituted congener like Ey could not be obtained, even at 0–20 °C. However, heating the reaction mixture at 50–70 °C, the respective salt (71p) was converted into an oxadiazole like 78.62 Its formation partly resembles the process (71a → 75) (cf. Scheme 25); yet, the linear species which resulted from loss of dinitrogen underwent cyclization involving one nitro group to give an N-oxide that was deoxygenated.
(ii) Methylation of the tetrazolyl-substituted malonaldehyde (79) with methyl fluorosulfate to be followed by treatment with a weak base gave the diformylmethylide (Ez) 63 (according to ref.63c an unstable oil). Usually, quaternization of 1H-tetrazoles leads to isomers, which in the present case might have produced also some ylide (F). In addition to the ylide (Ez), the preparation of its N-ethyl analogue was claimed, but without giving experimental details and characterization data.63a,c
c) Classes (F) and (G)
Investigations of ylides (F) have been conducted as part of a comparative study including the isomers (G) (Scheme 28).45 It seems therefore appropriate to treat both classes jointly.
Following the classical Kröhnke route, action of potassium carbonate on the salts (72a-d) and (73a-d) afforded the ylides (Fa-d) and (Gc-f) in excellent yield. These compounds crystallize in anhydrous form, in contrast to their β-type congeners (Ed-l) (cf. Scheme 25). Derivatives having an unsubstituted ring carbon are stable as well. Considering the stronger electron-withdrawing influence exerted by the azolium system in G [cf. Section (2a)], reactions of the ylides (F) with electrophiles should proceed more readily. This is borne out as follows:
(i) While aroylation of the salts (72a) and (73a) under Schotten-Baumann conditions gave the ylides (Fe) and (Gg) in similar yield, the formation of Fe occurred much more rapidly; accordingly, experiments with the nitrophenacyl salts (72d) and (73d) succeeded only with the former (→ Ff); (ii) employing phenyl isocyanate, the conversion of the ylides (Fa,c) into the carbamoylated derivatives (Fg,h) was distinctly faster (about four times) than the analogous process (Gc,e → Gh,i); (iii) attempts to thiocarbamoylate the ylides (Fd) and (Gf) failed with the latter.
Alkylation studies with methyl iodide or benzyl bromide revealed that desired products could be obtained only from the isomers (F), i.e. salts of the type (80). But since the derivative (80b) was accompanied by 2-methyltetrazole (81) and the α-bromodihydrochalcone (82a), an inherent lability of the C–N bond of 80 became apparent. This weakness turned out to be even more pronounced with salts that were expected from: benzyl bromide and (a) Fd / (b) Ge / (c) Gf, because in these cases only mixtures of (a) 81 + 82b / (b) 83 + 82a / (c) 83 + 82b were isolated.
Most significant is a comparison of Kröhnke's 'acid splitting', i.e. the action of alkali hydroxide on the pairs of isomers (Fa/Gc), (Fc/Ge), and (Fd/Gf) (Scheme 29): it was found that all compounds (G) have a much higher proclivity for giving the benzoate salt (69a). This nicely matches the results in Section (2a).
A prominent spectroscopic evidence for the stronger electron-withdrawal of the tetrazolium moiety in system (G) was provided by the less pronounced (negative) solvatochromism of the visible absorption band (intramolecular charge-transfer band) of the phenacylide (Gc) compared to the ylide (Fa) (Chart 4); a plot of the transition energies ET of these i.c.t. bands against the empirical solvent polarity Z revealed linear relationships.45 Later, similar findings were made with the isosteric N-aminides (84) and (85).64
Comparing the lowest energy geometries of the acetylides (Fac) and (Gac) [including that of (Eac)], it is most noteworthy that only the derivative (Gac) shows a fully planar structure (Figure 1).53,54 This has also been reported for the corresponding acetylaminides (N instead of CH) which, like Eac–Gac, exhibit a Z configured functional group throughout.65
Finally, outside the foregoing comparative study two scattered ylides of the class (G) deserve mention (Scheme 30): Along with the preparation of analogous ylides (E) (cf. Scheme 27), the compounds (Gj)60 and (Gk)63 have been made; their properties are essentially those of the isomers (Ex) and (Ez).
CONCLUSION
Reviewing the rich material on ylides (A–G) that has been accumulated in three decades, specific features emerged in great number. The most prominent ones are summarized as follows: (i) ylides (A) are capable of rearranging to allylides of class (B) via elusive cycloadducts; (ii) unsubstituted methylides (C) exhibit dichotomous reactivity towards ring expansion and cycloaddition; (iii) heteroring-fused ylides (D) are synthons for other heterocycles by virtue of a relatively weak N–N bond; (iv) isomeric tetrazolium ylides (E), (F), and (G) show properties that nicely reflect the different electronic influence exerted by the single ring systems.
However, despite much progress certain desiderata exist: (i) direct access to ylides (B) by depronotation of appropriate triazolium salts; (ii) generation (including cycloaddition reactions) of ylides (E) that are unsubstituted at C(5); (iii) approach to ylides (H–J) (below) by overcoming: a) the general inaccessibility of (monocyclic) 1,2-substitution patterns, and b) the difficulty of getting a 2,3-pattern with ligands other than aromatic.
ACKNOWLEDGEMENT
Thanks are due to Dr. L. Preu of this Institute for discussions and calculations of the ylides (Ai/Ai').
References
1. I. Zugrăvescu and M. Petrovanu, 'Chimia N-ilidelor,' Editura Academiei Republicii Socialiste România, Bucharest, 1974; English translation: 'N-Ylid Chemistry,' McGraw-Hill, London, 1976.
2. G. Surpateanu and A. Lablache-Combier, Heterocycles, 1984, 22, 2079. CrossRef
3. Y. Karzazi and G. Surpateanu, Heterocycles, 1999, 51, 863. CrossRef
4. P. Woisel, G. G. Surpateanu, and G. Surpateanu, Targets Heterocycl. Syst., 2001, 5, 461.
5. M. T. Gandasegui and J. Alvarez-Builla, Heterocycles, 1990, 31, 1801. CrossRef
6. A. R. Katritzsky, B. Yang, J. Jiang, and P. J. Steel, Heterocycles, 1995, 41, 765. – By contrast, an experiment with Aa and dimethyl butynedioate attempted in ref.5 did not give identifiable products. CrossRef
7. a) N. D. Miron, P. Woisel, G. G. Surpateanu, G. Vergoten, L. Depature, and G. Surpateanu, Can. J. Chem., 2003, 81, 350. – For related studies on Ad-g (theoretical, in part preparative), cf.; CrossRef b) N. D. Miron, G. Surpateanu, I. E. Mardare, and P. I. Dron, Stud. Cerc. Stiint. Chim. Ing. Chim. Bioteh. Ind. Aliment. Univ. Bacau, 2001, 2 (1-2), 21 (Chem. Abstr., 2003, 139, 21802); c) N. D. Miron and G. Surpateanu, 'Actes du Colloque Franco-Romain de Chimie Appliquée, 2nd,' Bacau (Romania) 2002, p. 167 (Chem. Abstr., 2003, 138, 303769); d) N. D. Miron, G. Surpateanu, I. E. Mardare, and P. I. Dron, Stud. Cerc. Stiint. Chim. Ing. Chim. Bioteh. Ind. Aliment. Univ. Bacau, 2001, 2 (1-2), 19 (Chem. Abstr., 2004, 140, 42095); e) N. D. Miron, G. Surpateanu, I. E. Mardare, and P. I. Dron, 'Actes du Colloque Franco-Romain de Chimie Appliquée, 2nd,' Bacau (Romania) 2002, p. 95 (Chem. Abstr., 2004, 140, 4992). – For a conformational study of 1b,c (including the analogue having Ar = Ph), see; f) N. D. Miron, G. Surpateanu, D. Nistor, I. E. Mardare, and P. I. Dron, Stud. Cerc. Stiint. Chim. Ing. Chim. Bioteh. Ind. Aliment. Univ. Bacau, 2002, 3 (1-2), 17 (Chem. Abstr., 2003, 139, 36109).
8. a) G. G. Surpateanu and N. D. Miron, Ann. West Univ. Timisoara, Ser. Chem., 2003, 12, 115 (Chem. Abstr., 2005, 142, 74116). – For another preparative study on Ak/Ak', see; b) N. D. Miron, D. I. Nistor, I. Bucu, and G. Surpateanu, Anal. Univ. Ovidius Const., Ser. Chim., 2005, 16 (2), 208 (Chem. Abstr., 2009, 151, 33493).
9. The authors' AM1 calculations show that Ai' is favoured by 0.127a and 0.23 kcal/mol.8a Regarding their PM3 studies, the results are not uniform, showing Ai' energetically both higher (0.62)7a and lower (0.69 kcal/mol)8a than Ai. This discrepancy led us to recalculate Ai and Ai', whereupon we found the latter isomer to be clearly lower in energy (1.25 kcal/mol). Duplication of the above AM1 computation gave a smaller difference between both species (0.37 kcal/mol), which is consistent with the former findings7a,8a (L. Preu and D. Moderhack, unpublished results).
10. N. D. Miron, G. G. Surpateanu, I. Badea, F. Cazier, and G. Surpateanu, Croat. Chem. Acta, 2006, 79, 553.
11. I. Badea, G. G. Surpateanu, P. Woisel, G. Vergoten, and G. Surpateanu, New J. Chem., 2002, 26, 1658. CrossRef
12. W. G. Phillips and K. W. Ratts, J. Org. Chem., 1970, 35, 3144. CrossRef
13. X. Xiao, D. Lin, S. Tong, H. Luo, Y. He, and H. Mo, Synlett, 2011, 1731. CrossRef
14. A. L. Romanyuk, B. L. Litvin, and N. I. Ganushchak, Zh. Obshch. Khim., 2002, 72, 304; Russ. J. Gen. Chem., 2002, 72, 280. CrossRef
15. E. Diez-Barra, C. Pardo, and J. Elguero, J. Org. Chem., 1982, 47, 4409. CrossRef
16. M. Begtrup, J. Elguero, E. Diez-Barra, and C. Pardo, Magn. Res. Chem., 1985, 23, 111. CrossRef
17. G. Seitz and R. Tegethoff, Chem.-Ztg., 1991, 115, 256. – Formally, the ylide (Bd) belongs to the δ2 category.
18. Exemplified with 1-methyl-1H-tetrazole: A. Lembcke, 'Tetrazolium-N-phenacylide – Synthese und Eigenschaften,' Dissertation, Technical University of Braunschweig (Germany), 1985.
19. a) E. Díez-Barra, C. Pardo, J. Elguero, and J. Arriau, J. Chem. Soc., Perkin Trans. 2, 1983, 1317; CrossRef E. Díez-Barra, J. Elguero, and C. Pardo, Heterocycles, 1984, 22, 1335. CrossRef
20. For a short review, see: R. N. Butler and D. F. O'Shea, Heterocycles, 1994, 37, 571. CrossRef
21. R. N. Butler, J. P. Duffy, D. Cunningham, P. McArdle, and L. A. Burke, J. Chem. Soc., Chem. Commun., 1990, 882. CrossRef
22. R. N. Butler, J. P. Duffy, D. Cunningham, P. McArdle, and L. A. Burke, J. Chem. Soc., Perkin Trans. 1, 1992, 147. CrossRef
23. R. N. Butler, J. M. McMahon, P. D. McDonald, C. S. Pyne, S. Schambony, P. McArdle, and D. Cunningham, J. Chem. Soc., Perkin Trans. 1, 1997, 1047. CrossRef
24. R. N. Butler, E. C. McKenna, J. M. McMahon, K. M. Daly, D. Cunningham, and P. McArdle, J. Chem. Soc., Perkin Trans. 1, 1997, 2919. CrossRef
25. R. N. Butler, P. D. McDonald, P. McArdle, and D. Cunningham, J. Chem. Soc., Perkin Trans. 1, 1994, 1653. CrossRef
26. R. N. Butler, P. D. McDonald, P. McArdle, and D. Cunningham, J. Chem. Soc., Perkin Trans. 1, 1996, 1617. CrossRef
27. R. N. Butler and D. C. Grogan, J. Chem. Res. (S), 1997, 428.
28. R. N. Butler and L. M. Wallace, J. Chem. Soc., Perkin Trans. 1, 2001, 1778. CrossRef
29. D. Moderhack, A. Daoud, and P. G. Jones, Monatsh. Chem., 2002, 133, 1165. CrossRef
30. D. Moderhack and M. Lorke, Heterocycles, 1987, 26, 1751; CrossRef – For further studies, see: D. Moderhack, M. Lorke, L. Ernst, and D. Schomburg, Chem. Ber., 1994, 127, 1633; CrossRef D. Moderhack and A. Daoud, J. Heterocycl. Chem., 2003, 40, 625. CrossRef
31. R. N. Butler, M. O. Cloonan, P. McArdle, and D. Cunningham, J. Chem. Soc., Perkin Trans. 1, 1999, 1415. CrossRef
32. R. N. Butler and A. Fox, J. Chem. Soc., Perkin Trans. 1, 2001, 394. CrossRef
33. R. N. Butler, K. M. Daly, J. M. McMahon, and L. A. Burke, J. Chem. Soc., Perkin Trans. 1, 1995, 1083. CrossRef
34. R. N. Butler, M. O. Cloonan, J. M. McMahon, and L. A. Burke, J. Chem. Soc., Perkin Trans. 1, 1999, 1709. CrossRef
35. R. N. Butler and M. O. Cloonan, Bull. Soc. Chim. Belg., 1997, 106, 515; R. N. Butler, M. O. Cloonan, G. M. Smyth, P. McArdle, and D. Cunningham, ARKIVOC, 2003, vii, 244. CrossRef
36. R. N. Butler, M. O. Cloonan, P. McArdle, and D. Cunningham, J. Chem. Soc., Perkin Trans. 1, 1998, 1295; CrossRef R. N. Butler, G. M. Smyth, M. O. Cloonan, P. McArdle, and D. Cunningham, Chem. Commun., 2001, 1950; R. N. Butler, G. M. Smyth, P. McArdle, and D. Cunningham, J. Chem. Soc., Perkin Trans. 1, 2002, 2851. CrossRef
37. B. Abarca, R. Ballesteros, F. Mojarrad, M. R. Metni, S. Garcia-Granda, E. Perez-Carreño, and G. Jones, Tetrahedron, 1991, 47, 5277. CrossRef
38. B. Abarca, R. Ballesteros, and N. Houari, Tetrahedron, 1997, 53, 12765. CrossRef
39. B. Abarca, R. Ballesteros, and M. R. Metni, Heterocycles, 1992, 33, 203. CrossRef
40. B. Abarca, R. Ballesteros, M. R. Metni, G. Jones, D. J. Ando, and M. B. Hursthouse, Tetrahedron Lett., 1991, 32, 4977. CrossRef
41. B. Abarca, R. Ballesteros, N. Houari, and A. Samadi, Tetrahedron, 1998, 54, 3913. CrossRef
42. B. Abarca, R. Ballesteros, M. J. Del Rincón-Guaita, and G. Jones, Heterocycles, 1994, 38, 2017. CrossRef
43. B. Abarca and R. Ballesteros, Heterocycles, 1993, 35, 851. CrossRef
44. F. Kröhnke, Angew. Chem., 1953, 65, 605. CrossRef
45. D. Moderhack and A. Lembcke, J. Chem. Soc., Perkin Trans. 1, 1986, 1157 (see also p. 2009). CrossRef
46. B. Abarca, R. Ballesteros, M. R. Metni, G. Jones, D. J. Ando, and M. B. Hursthouse, Heterocycles, 1992, 34, 1005. CrossRef
47. B. Abarca, R. Ballesteros, A. Muñoz, and G. Jones, Tetrahedron, 1996, 52, 10519. CrossRef
48. B. Abarca, R. Ballesteros, and N. Houari, ARKIVOC, 2000, iii, 282. CrossRef
49. W. P. Norris and R. A. Henry, Tetrahedron Lett., 1965, 1213. CrossRef
50. T. Isida, S. Fujimori, K. Nabika, K. Sisido, and S. Kozima, Bull. Chem. Soc. Jpn., 1972, 45, 1246. CrossRef
51. M. A. Schroeder and R. A. Henry, 'Quantum Mechanical Studies on Chemical Reactivity and Ballistic Chemistry. VII. Semiempirical Molecular Orbital Calculations and Experimental Studies on Relative Chemical Reactivities of Isomeric Tetrazole Derivatives, and Their Relationship to the Explosive Properties of Some Tetrazole Derivatives,' U. S. Army Ballistic Research Laboratory Technical Report ARBRL-TR-02371 (AD A107288), Aberdeen Proving Ground, Maryland, 1981 (Chem. Abstr., 1982, 97, 22882).
52. M. A. Schroeder and R. C. Makino, Tetrahedron, 1973, 29, 3469. CrossRef
53. D. Moderhack, unpublished results.
54. Gaussian 98, Revision A.9, M. J. Frisch, G. W. Trucks, H. B. Schlegel, G. E. Scuseria, M. A. Robb, J. R. Cheeseman, V. G. Zakrzewski, J. A. Montgomery, Jr., R. E. Stratmann, J. C. Burant, S. Dapprich, J. M. Millam, A. D. Daniels, K. N. Kudin, M. C. Strain, O. Farkas, J. Tomasi, V. Barone, M. Cossi, R. Cammi, B. Mennucci, C. Pomelli, C. Adamo, S. Clifford, J. Ochterski, G. A. Petersson, P. Y. Ayala, Q. Cui, K. Morokuma, D. K. Malick, A. D. Rabuck, K. Raghavachari, J. B. Foresman, J. Cioslowski, J. V. Ortiz, A. G. Baboul, B. B. Stefanov, G. Liu, A. Liashenko, P. Piskorz, I. Komaromi, R. Gomperts, R. L. Martin, D. J. Fox, T. Keith, M. A. Al-Laham, C. Y. Peng, A. Nanayakkara, M. Challacombe, P. M. W. Gill, B. Johnson, W. Chen, M. W. Wong, J. L. Andres, C. Gonzalez, M. Head-Gordon, E. S. Replogle, and J. A. Pople, Gaussian, Inc., Pittsburgh PA, 1998.
55. D. Moderhack and D.-O. Bode, J. Chem. Soc., Perkin Trans. 1, 1992, 1483. CrossRef
56. D. Moderhack, D. Decker, and B. Holtmann, J. Chem. Soc., Perkin Trans. 1, 2001, 720. CrossRef
57. a) D. Moderhack, D.-O. Bode, and D. Schomburg, Chem. Ber., 1993, 126, 129; CrossRef b) D. Moderhack, D.-O. Bode, and D. Schomburg, '100 Jahre HN3 – Das Erbe von Theodor Curtius,' International Sym¬posium under the auspices of Chemische Gesellschaft zu Heidelberg and Gesellschaft Deutscher Chemiker (GDCh), Heidelberg, Germany, 1990; Abstracts of Papers, poster 19.
58. Preceding examples of using ammonia for the preparation of ylides are cited in ref.57a.
59. D.-O. Bode, '1,5-Dialkyltetrazolium-4-phenacylide – Untersuchungen zur Darstellung und Reaktivi¬tät,' Dissertation, Technical University of Braunschweig (Germany), 1991.
60. V. V. Semenov, S. A. Shevelev, and L. G. Mel'nikova, Mendeleev Commun., 1993, 58. CrossRef
61. V. V. Semenov, S. A. Shevelev, A. B. Bruskin, M. I. Kanishchev, and A. T. Baryshnikov, Izv. Akad. Nauk, Ser. Khim., 2009, 2014; Russ. Chem. Bull. Int. Ed., 2009, 58, 2077. CrossRef
62. A. R. Katritzky, G. L. Sommen, A. V. Gromova, R. M. Witek, P. J. Steel, and R. Damavarapu, Khim. Geterotsikl. Soedin., 2005, 127; Chem. Heterocycl. Compd., 2005, 41, 111. CrossRef
63. a) V. Král, Z. Arnold, V. V. Semenov, S. A. Shevelev, and A. A. Fainzil'berg, Izv. Akad. Nauk SSSR, Ser. Khim., 1983, 955; Bull. Acad. Sci. USSR, Div. Chem. Sci., 1983, 32, 865; CrossRef b) V. Král, V. V. Semenov, M. I. Kanishchev, and Z. Arnold, 'Proceedings of the IXth Symposium on Chemistry of Heterocyclic Compounds,' Bratislava 1987, p. 358; c) V. Král, V. V. Semenov, M. I. Kanishchev, Z. Arnold, S. A. Shevelev, and A. A. Fainzil'berg, Collect. Czech. Chem. Commun., 1988, 53, 1519. CrossRef
64. M. Noreiks, 'Tetrazolium-N-aminide – Synthese und Eigenschaften (mit einem Beitrag zur Phenylcarbamoylierung von 1,2,4-Triazolium-Analoga),' Dissertation, Technical University of Braunschweig (Germany), 2006.
65. D. Moderhack and M. Noreiks, Heterocycles, 2004, 63, 2605. CrossRef
66. E. M. Kosower, J. Am. Chem. Soc., 1958, 80, 3253. CrossRef
67. C. Reichardt, Angew. Chem., 1965, 77, 30; CrossRef Angew. Chem., Int. Ed. Engl., 1965, 4, 29. CrossRef
68. H.-J. Timpe and H. G. O. Becker, Chimia, 1972, 26, 473.
69. Instead of the proper system (J) there is only a remote analogue having a C-linked tetrazolide ring at N(3): a) R. Kuhn and H. Kainer, Angew. Chem., 1953, 65, 442; CrossRef b) V. P. Shchipanov, K. I. Krashina, and A. A. Skachilova, Khim. Geterotsikl. Soedin., 1973, 1570; Chem. Heterocycl. Compd. (USSR), 1973, 9, 1423. CrossRef