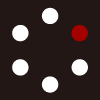
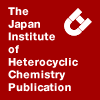
HETEROCYCLES
An International Journal for Reviews and Communications in Heterocyclic ChemistryWeb Edition ISSN: 1881-0942
Published online by The Japan Institute of Heterocyclic Chemistry
e-Journal
Full Text HTML
Received, 28th July, 2014, Accepted, 28th August, 2014, Published online, 11th September, 2014.
DOI: 10.3987/COM-14-S(K)92
■ Synthesis of Pyrrolidinofullerenes via Single Electron Transfer Reaction of Aryldienamines with C60
Naohiko Ikuma,* Hiroyuki Yamamoto, Ken Kokubo, and Takumi Oshima
Department of Applied Chemistry, Graduate School of Engineering, Osaka University, 2-1 Yamadaoka, Suita, Osaka 565-0871, Japan
Abstract
Variously aryl-substituted pyrrolidinofullerenes were synthesized via a single electron transfer (SET) reaction of diaryldienamines with C60 and the following consecutive 1,6-hydrogen shift and the [3 + 2] cycloaddition of the generated radical ion pair. The LUMO levels of pyrrolidinofullerenes were ca. 0.1 eV higher than C60, consequently suppressing the bisadduct formation. The phenyl –substituted pyrrolidinofullerene 2a representatively exhibited the protic acid-catalyzed intramolecular Friedel–Crafts cyclization and the DDQ induced oxidative reversion into C60.INTRODUCTION
Pyrrolidinofullerenes1–6 are useful fullerene derivatives in materials chemistry and for medicinal applications, as liquid crystals,5 light-converting substances,6 and chiral fullerene compounds.3,4 These fullerenes are easily synthesized from the [3 + 2] cycloaddition of azomethine ylides which were prepared from various precursors such as carbonyl compound/amino acid (Prato reaction),1,2 aziridine,1,3 lactone,1 and imine.4 In very recent communication, we have reported that morpholinodiphenyldienamine 1a underwent a single electron transfer (SET) reaction with C60 to give diarylpyrrolidinofullerene 2a via the subsequent 1,6-hydrogen shift and the [3 + 2] cycloaddition of the radical cation with C60 radical anion (Scheme 1).7 We also confirmed the reaction mechanism by DFT calculations as well as the radical-trapping experiment. In this paper, we report the synthesis of variously aryl-substituted pyrrolidinofullerenes by way of this SET/H-shift process. Moreover, we have examined the electronic properties and thermal stability (by DSC and TGA), TfOH catalyzed transformation, and DDQ induced oxidative degradation.
RESULTS AND DISCUSSION
As in the case of 2a, variously aryl-substituted pyrrolidinofullerenes 2b–h were synthesized as shown in Table 1. Dienamines 1b–h were prepared by the condensation of arylketones and cyclic amines with p-toluenesulfonic acid (TsOH) catalyst. Due to the lability, less volatile dienamines 1b–h were immediately used for the reaction with C60 without further purification and identification. These crude dienamines (ca. 25 equiv) were reacted with C60 in toluene at 90 ºC. The reaction was traced by HPLC (Buckyprep column, toluene eluent). Unfavorable contaminants such as enamines and unreacted arylketones appreciably neither inhibited the present cycloaddition nor brought about the side reactions. The reaction solution was evaporated and the residue was submitted for column chromatography to give pure monoadducts 2b–h in slightly lower yields (ca. 20–40 %) than 2a. Piperidinodienamine 1b reacted faster than morpholinodienamine 1a on account of higher electron-donating piperidine substituent, so that the isolated yield of 2b slightly decreased due to the formation of multiadducts. Similarly, the reaction of morpholinodienamines with donative aryl groups such as 4-alkylphenyl (1d–f) and thiophene 1h gave slightly lower yields because of the multiaddition. On the other hand, p-chlorophenyl-substituted dienamine 1c needed slightly longer reaction time because of lower electron-donating ability. The 1H/13C NMR spectra of these 1:1 adducts showed similar signals to those of 2a; appearances of methyl group and asymmetric morpholino/piperidino ring were only explained by the hydrogen shift of dienamino radical cation and [3 + 2] cycloaddition.
For the application to electronic materials, we estimated the electron affinity of pyrrolidinofullerenes 2a–h. Cyclic voltammogram measurements showed ca. 0.1 V lower reduction potentials than that of C60, implying the pyrrolidino-fusion lowered the electrophilicity of fullerene core (Table 2). This lowering electron affinity of monoadducts will suppress further SET reaction giving bisadducts. However, these substituent effects are not so effective because of indirect inductive effect of the aryl substituents.
Thermal stability of 2a was evaluated by DSC (Figure 1a) and TGA (Figure 1b) measurements. One endothermic peak followed by exothermic plateau in DSC and drastic weight loss (ca. 13%) in TGA were observed around 260–270 ºC, suggesting melting with decomposition. This decomposition temperature is comparable with those of pyrrolidinofullerenes bearing BOC-group (>250 ºC),6b but lower than those of liquid pyrrolidinofullerenes with stable alkoxylphenyl groups (340–420 ºC).9 These previous studies indicate the pyrrolidino ring seems to be stable around 300 ºC unless the compound has less stable substituents such as BOC group. Thus we can consider the decomposition of 2a at 265 ºC is due to the elimination of methylstyrene moiety, in consistent with the weight loss of TGA (F.W. of CH=CMePh is 117, whereas M.W. of 2a is 1012). 10
We have carried out acid treatment of 2a because these pyrrolidinofullerenes inherently have basic site at the nitrogen of pyrrolidino moiety. However, by adding excess amount of TfOH (25 equiv), the amino linkage was retained but the intramolecular Friedel–Crafts type cyclization occurred to give spiro compound 3a (Scheme 2) as confirmed by 1H/13C NMR and 2D-HSQC/HMBC/NOESY correlation (Supporting Information). Similar to styrene which easily polymerizes with electrophilic initiator, phenylvinyl group of 2a may be protonated at the β-position to form relatively stable benzylic carbocation. This cationic center is attacked by adjoining phenyl ring to construct the stable dihydroindene ring.11 In this acid treatment, although pyrrolidino function can be protonated, the resulting quarterly ammonium ion seems to persist any further transformation. The reaction proceeded in various polar aromatic solvents such as o-DCB, and anisole (Table 3). Anisole and toluene provided slightly lower yield, probably because these solvents are likely to participate in the Friedel–Crafts reaction at the fullerene sphere.12,13
Pyrrolidinofullerenes are known to undergo the cycloreversion into azomethine ylide and C60 under the influence of oxidant such as Cu(II) and trapping dipolarophiles.14 Similarly, oxidation of 2a by DDQ led to the regeneration of C60 even without trapping agents (Scheme 3). Since the unsubstituted pyrrolidinofullerene 41 was not oxidized by DDQ, the oxidative abstraction of methine proton of pyrrolidine ring of 2a seems to be essential for the cycloreversion.
EXPERIMENTAL
General Synthetic Procedure of 2: The solution of cyclic amines (100 mmol), arylketone (100 mmol), and catalytic amount of p-toluenesulfonic acid (TsOH, <100 mg) in toluene (50 mL) was stirred at refluxing temperature (140 ºC) under nitrogen for 5–10 h using a Dean-Stark apparatus. After the removal of solvent, unreacted acetophenone and morpholine were almost removed in vacuo. The residue was diluted with pentane to precipitate TsOH, then filtrated and evaporated. Crude products 1b–h were employed in the next reaction without further purifications. The solution of C60 (100 mg, 0.14 mmol) and dienamines 1 (ca. 3.5 mmol, 25 equiv) in toluene (50 mL) was stirred in dark at 90 ºC under nitrogen. The reaction was traced by HPLC (Buckyprep, toluene eluent). After filtration, the solvent was removed in vacuo. The residue was chromatographed on silica gel to provide the monoadduct 2b–h.
Compound 2b. 1H-NMR: (600 MHz, CDCl3) δ 1.80 (s, 3H), 2.01−2.09 (m, 2H), 2.17−2.23 (m, 2H), 2.31−2.34 (dd, 1H, J = 6.8, 13.4 Hz), 2.71−2.74 (m, 2H), 3.61−3.63 (d, 1H, J = 10.9 Hz), 4.59−4.61 (dd, 1H, J = 3.0, 11.2 Hz), 7.25−7.27 (m, 2H), 7.30−7.33 (t, 1H, J = 7.5 Hz), 7.38−7.41 (t, 2H, J = 7.9 Hz), 7.45−7.48 (m, 1H), 7.46 (s, 1H), 7.59−7.61 (d, 2H, J = 7.3 Hz), 7.71−7.73 (d, 1H, J = 6.9 Hz), 8.23−8.24 (d, 1H, J = 8.0 Hz). 13C-NMR: (150 MHz, CDCl3) δ 20.73, 25.26, 25.88, 32.33, 47.77, 69.99, 73.41, 79.71, 81.97, 126.39, 127.41, 127.72, 127.75, 127.80, 128.25, 128.69, 128.86, 130.28, 135.81, 136.03, 136.53, 137.49, 138.51, 139.42, 139.49, 139.96, 140.25, 141.24, 141.60, 141.65, 141.75, 141.78, 141.93, 141.99, 142.08, 142.12, 142.15, 142.30, 142.33, 142.46, 142.53, 142.66, 142.69, 143.05, 143.15, 143.24, 144.35, 144.41, 144.62, 144.66, 144.83, 145.08, 145.12, 145.14, 145.16, 145.21, 145.23, 145.27, 145.52, 145.59, 145.60, 145.79, 145.80, 145.84, 146.10, 146.12, 146.15, 146.21, 146.32, 146.36, 146.92, 146.99, 147.25, 147.29, 147.35, 152.82, 154.50, 154.62, 155.79. MS (MALDI-MS): m/z = 1009.
Compound 2c. 1H-NMR: (600 MHz, CDCl3) δ 1.81 (s, 3H), 3.05−3.10 (dt, 1H, J = 3.5, 11.4 Hz), 3.40−3.42 (d, 1H, J = 11.0 Hz), 4.10−4.14 (dt, 1H, J = 2.6, 11.4 Hz), 4.22−4.26 (t, 1H, J = 10.3 Hz), 4.24−4.26 (d, 1H, J = 10.5 Hz), 4.75−4.77 (dd, 1H, J = 3.2, 10.1 Hz), 4.81−4.83 (dd, 1H, J = 3.1, 10.5 Hz), 7.30−7.32 (dd, 1H, J = 2.3, 8.5 Hz), 7.41−7.43 (m, 1H), 7.42−7.43 (d, 1H, J = 8.7 Hz), 7.44 (s, 1H), 7.48−7.50 (dd, 1H, J = 2.3, 8.5 Hz), 7.55−7.57 (m, 1H), 7.55−7.57 (d, 1H, J = 8.7 Hz), 7.66−7.68 (dd, 1H, J = 2.3, 8.5 Hz), 8.19−8.21 (dd, 1H, J = 2.3, 8.5 Hz) 13C-NMR: (150 MHz, CDCl3) δ 20.74, 47.86, 66.74, 67.48, 70.87, 71.82, 79.06, 82.13, 126.79, 127.59, 128.76, 128.85, 129.07, 131.23, 133.80, 133.81, 135.80, 136.12, 136.54, 137.36, 138.06, 138.92, 139.49, 139.75, 140.35, 141.33, 141.54, 141.60, 141.65, 141.80, 141.94, 141.96, 142.03, 142.09, 142.23, 142.29, 142.30, 142.33, 142.47, 142.59, 142.71, 142.74, 143.09, 143.28, 144.30, 144.33, 144.47, 144.53, 144.71, 145.16, 145.19, 145.22, 145.24, 145.28, 145.33, 145.34, 145.42, 145.60, 145.68, 145.87, 145.93, 146.15, 146.16, 146.19, 146.28, 146.34, 146.38, 146.48, 146.58, 147.30, 147.38, 152.07, 152.94, 153.31, 153.37. MS (MALDI-MS): m/z = 1079.
Compound 2d. 1H-NMR: (600 MHz, CDCl3) δ 1.81 (s, 3H), 2.34 (s, 3H), 2.41 (s, 3H), 3.07−3.11 (dt, 1H, J = 3.4, 11.4 Hz), 3.45−3.47 (d, 1H, J = 10.9 Hz), 4.10−4.14 (dt, 1H, J = 2.8, 11.4 Hz), 4.22−4.24 (d, 1H, J = 11.1 Hz), 4.22−4.26 (d, 1H, J = 10.9 Hz), 4.79−4.81 (t, 1H, J = 7.9 Hz), 4.79−4.82 (t, 1H, J = 7.6 Hz), 7.10−7.11 (d, 1H, J = 8.1 Hz), 7.26−7.29 (m, 2H), 7.46 (s, 1H), 7.54−7.56 (d, 2H, J = 8.2 Hz), 7.59−7.61 (dd, 1H, J = 1.9, 8.1 Hz), 8.10−8.12 (dd, 1H, J = 1.9, 8.1 Hz). 13C-NMR: (150 MHz, CDCl3) δ 20.70, 21.15, 21.25, 47.84, 66.89, 67.35, 71.00, 71.90, 79.50, 82.54, 126.04, 126.18, 127.43, 129.27, 129.32, 129.37, 130.13, 135.57, 136.30, 136.43, 136.82, 137.34, 137.43, 137.60, 138.70, 139.35, 139.60, 140.24, 141.30, 141.49, 141.61, 141.66, 141.71, 141.92, 141.97, 142.00, 142.09, 142.25, 142.27, 142.32, 142.40, 142.53, 142.63, 142.64, 142.66, 143.02, 143.09, 143.22, 144.30, 144.36, 144.57, 144.62, 144.68, 145.08, 145.10, 145.13, 145.8, 145.21, 145.25, 145.26, 145.49, 145.64, 145.68, 145.81, 145.87, 146.07, 146.10, 146.21, 146.30, 146.35, 146.80, 147.05, 147.27, 152.81, 153.70, 153.84, 153.93. MS (MALDI-MS): m/z = 1039.
Compound 2e. 1H-NMR: (600 MHz, CDCl3) δ 1.19−1.21 (t, 3H, J = 7.6 Hz), 1.28−1.30 (t, 3H, J = 7.6 Hz), 1.813 (s, 3H), 2.61−2.65 (q, 2H, J = 7.6 Hz), 2.69−2.73 (q, 2H, J = 7.6 Hz), 3.08−3.12 (dt, 1H, J = 3.5, 11.4 Hz), 3.47−3.49 (d, 1H, J = 11.0 Hz), 4.10−4.15 (dt, 1H, J = 2.3,11.4 Hz), 4.23−4.26 (t, 1H, J = 11.0 Hz), 4.22−4.24 (d, 1H, J = 11.1 Hz), 4.80−4.81 (d, 1H, J = 10.5 Hz), 4.80−4.81 (d, 1H, J = 10.3 Hz), 7.10−7.11 (dd, 1H, J = 1.7, 8.1 Hz), 7.29−7.31 (m, 3H), 7.49 (s, 1H), 7.58−7.60 (m, 2H), 7.61−7.62 (dd, 1H, J = 2.0, 8.1 Hz), 8.13−8.14 (dd, 1H, J = 1.9, 8.1 Hz). 13C-NMR: (150 MHz, CDCl3) δ 15.53, 15.60, 20.66, 28.47, 28.54, 47.82, 66.89, 67.34, 71.01, 71.89, 79.55, 82.58, 125.99, 126.22, 127.51, 128.00, 128.03, 128.14, 128.23, 129.04, 130.16, 135.50, 136.31, 136.31, 136.64, 136.84, 137.35, 138.61, 139.22, 139.34, 139.59, 140.23, 141.29, 141.59, 141.62, 141.65, 141.67, 141.71, 141.92, 141.97, 142.00, 142.09, 142.25, 142.26, 142.32, 142.52, 142.62, 142.63, 142.66, 143.02, 143.22, 143.91, 143.98, 144.30, 144.37, 144.59, 144.63, 144.67, 145.07, 145.10, 145.13, 145.14, 145.21, 145.24, 145.25, 145.49, 145.63, 145.68, 145.81, 145.86, 146.06, 146.09, 146.10, 146.19, 146.30, 146.35, 146.80, 146.84, 147.05, 147.27, 147.31, 152.81, 153.71, 153.85, 153.95. MS (MALDI-MS): m/z = 1067.
Compound 2f. 1H-NMR: (600 MHz, CDCl3) δ 0.82−0.84 (t, 3H, J = 7.1 Hz), 0.88−0.91 (t, 3H, J = 7.2 Hz), 1.18−1.39 (m, 16H), 1.84 (s, 3H), 2.57−2.60 (t, 2H, J = 7.4 Hz), 2.64−2.67 (t, 2H, J = 7.8 Hz), 3.09−3.13 (dt, 1H, J = 3.5, 11.4 Hz), 3.48−3.49 (d, 1H, J = 11.1 Hz), 4.10−4.14 (dt, 1H, J = 2.3, 11.4 Hz), 4.22−4.24 (d, 1H, J = 11.1 Hz), 4.22−4.26 (t, 1H), 4.80−4.81 (d, 1H, J = 10.6 Hz), 4.80−4.82 (d, 1H, J = 10.7 Hz), 7.08−7.09 (dd, 1H, J = 1.5, 8.1 Hz), 7.26−7.27 (m, 1H), 7.46−7.48 (t, 1H, J = 7.3 Hz), 7.50 (s, 1H), 7.56−7.59 (d, 2H, J = 8.4 Hz), 7.60−7.62 (dd, 1H, J = 1.8, 8.1 Hz), 7.96−7.98 (m, 1H), 8.12−8.14 (dd, 1H, J = 1.9, 8.1 Hz). 13C-NMR: (150 MHz, CDCl3) δ 14.15, 20.61, 22.63, 22.67, 28.54, 29.08, 31.16, 31.47, 31.71, 31.75, 35.45, 35.64, 47.80, 66.90, 67.36, 71.00, 71.89, 79.59, 82.63, 125.86, 126.12, 127.44, 128.32, 128.58, 128.66, 133.11, 135.45, 136.32, 136.72, 136.85, 137.13, 137.37, 138.56, 139.34, 139.58, 140.24, 141.29, 141.50, 141.59, 141.63, 141.69, 141.93, 141.98, 142.00, 142.09, 142.26, 142.31, 142.53, 142.62, 142.64, 142.66, 142.73, 143.02, 143.23, 144.31, 144.37, 144.60, 144.65, 144.67, 145.07, 145.11, 145.12, 145.15, 145.22, 145.25, 145.50, 145.64, 115.69, 145.82, 145.86, 146.06, 146.10, 146.11, 146.20, 146.31, 146.35, 146.81, 146.87, 147.05, 147.27, 147.32, 152.80, 153.71, 153.86, 153.95. MS (MALDI-MS): m/z = 1179.
Compound 2g. 1H-NMR: (600 MHz, CDCl3) δ 1.93 (s, 3H), 3.15−3.19 (dt, 1H, J = 3.3, 11.2 Hz), 3.54−3.56 (d, 1H, J = 11.0 Hz), 4.15−4.19 (dt, 1H, J = 2.4, 11.4 Hz), 4.26−4.30 (t, 1H, J = 11.6 Hz), 4.27−4.29 (d, 1H, J = 11.5 Hz), 4.83 −4.87 (t, 1H, J = 11.4 Hz), 4.84 −4.87 (t, 1H, J = 8.9 Hz), 7.32−7.35 (t, 1H, J = 7.4 Hz), 7.36−7.39 (dt, 1H, J = 7.4 Hz), 7.42−7.44 (t, 2H, J = 7.5 Hz), 7.46−7.48 (t, 2H, J = 7.5 Hz), 7.58−7.60 (dd, 1H, J = 1.9, 8.2 Hz), 7.61 (s, 1H), 7.63−7.64 (dd, 2H, J = 1.2, 8.4 Hz), 7.66−7.67 (dd, 1H, J = 1.2, 8.4 Hz), 7.70−7.71 (m, 2H), 7.75−7.78 (m, 3H), 7.82−7.84 (dd, 1H, J = 1.9, 8.2 Hz), 8.33−8.34 (dd, 1H, J = 1.9, 8.2 Hz). 13C-NMR: (150 MHz, CDCl3) δ 20.75, 47.96, 66.90, 67.49, 71.05, 71.92, 79.53, 82.51, 126.71, 126.98, 127.06, 127.23, 127.38, 127.45, 128.08, 128.82, 128.86, 130.59, 135.69, 136.29, 136.77, 137.43, 138.65, 138.76, 139.43, 139.67, 140.31, 140.34, 140.38, 140.55, 140.71, 141.31, 141.64, 141.67, 141.78, 141.95, 141.99, 142.03, 142.10, 142.25, 142.28, 142.35, 142.55, 142.66, 142.67, 142.70, 143.04, 143.06, 143.10, 143.25, 144.33, 144.37, 144.57, 144.60, 144.69, 145.12, 145.17, 145.19, 145.23, 145.27, 145.30, 145.50, 145.67, 145.84, 145.89, 146.11, 146.13, 146.16, 146.23, 146.34, 146.38, 146.72, 146.75, 146.90, 147.29, 147.34, 152.59, 153.56, 153.64, 153.66. MS (MALDI-MS): m/z = 1163.
Compound 2h. 1H-NMR: (600 MHz, CDCl3) δ 2.06 (s, 3H), 3.13–3.17 (dt, 1H, J = 3.3, 11.1 Hz), 3.79 (br, 1H), 4.09–4.13 (dt, 1H, J =2.1, 11.6 Hz), 4.20–4.23 (m, 2H), 4.70-4.73 (dd, 1H, J = 3.1, 10.1 Hz), 4.78–4.80 (dd, 1H, J =3.3, 10.6 Hz), 7.01 (br, 1H), 7.08–7.10 (dd, 2H, J = 3.5, 5.0 Hz), 7.23–7.24 (dd, 1H, J = 1.1, 3.6 Hz), 7.27–7.28 (d, 1H, J = 4.9 Hz), 7.33 (br, 1H), 7.75 (s, 1H). 13C-NMR: (150 MHz, CDCl3) δ 20.11, 29.68, 66.74, 70.66, 71.56, 76.57, 82.99, 123.98, 124.86, 127.80, 136.35, 136.55, 137.26, 138.70, 139.62, 140.25, 141.31, 141.41, 141.55, 141.59, 141.77, 141.93, 142.03, 142.13, 142.26, 142.39, 142.55, 142.60, 142.68, 143.05, 143.23, 144.30, 144.36, 144.46, 144.56, 144.69, 145.07, 145.14, 145.24, 145.33, 145.53, 145.67, 145.83, 145.87, 146.04, 146.08, 146.13, 146.26, 146.33, 146.39, 146.57, 147.29, 147.35, 147.79, 153.19, 153.47. MS (MALDI-MS): m/z = 1024 ([M+H]).
Compound 3a by TfOH acidification. To the solution of 2a (5 mg, 0.0049 mmol) in chlorobenzene, trifluoromethanesulfonic acid (TfOH, 18 mg, 0.12 mmol) was dropwisely added. The solution was stirred 5 h at 80 ºC. The reaction was quenched by adding water, and extracted with chlorobenzene. The solution was concentrated in vacuo. The product was purified by column chromatography, to give 3a (3.30 mg, 59%). 1H-NMR: (600 MHz, CDCl3) δ 1.93 (s, 3H), 2.82−2.83 (d, 1H, J = 10.3 Hz), 3.08−3.12 (dt, 1H, J = 4.9, 11.2 Hz), 3.38−3.41 (d, 1H, J = 14.7 Hz), 3.97−4.01 (dt, 1H, J = 2.5, 11.2 Hz), 3.98−4.01 (d, 1H, J = 14.7 Hz), 4.20−4.22 (d, 1H, , J = 10.6 Hz), 4.21−4.24 (t, 1H, J = 10.6 Hz), 4.43−4.45 (dd, 1H, J = 3.4, 10.3 Hz), 4.81−4.83 (dd, 1H, J = 3.4, 10.6 Hz), 6.99−7.02 (t, 1H, J = 7.3 Hz), 7.04−7.07 (t, 2H, J = 7.8 Hz), 7.19−7.21 (d, 2H, J = 7.2 Hz), 7.42−7.45 (dt, 1H, J = 1.2, 7.7 Hz), 7.46−7.47 (d, 1H, J = 7.7 Hz), 7.51−7.54 (dt, 1H, J = 1.2, 7.7 Hz), 7.85−7.87 (d, 1H, J = 7.7 Hz). 13C-NMR: (150 MHz, CDCl3) δ 33.77, 44.88, 45.52, 51.08, 67.06, 71.39, 71.96, 80.26, 85.07, 126.08, 127.03, 127.23, 128.23, 128.75, 129.36, 136.38, 136.59, 136.75, 139.10, 139.53, 139.76, 139.99, 140.63, 141.32, 141.37, 141.47, 141.69, 141.81, 141.86, 141.98, 141.99, 142.05, 142.14, 142.38, 142.54, 142.58, 142.76, 143.14, 144.00, 144.22, 144.50, 144.58, 144.85, 145.08, 145.12, 145.16, 145.22, 145.28, 145.30, 145.52, 145.56, 145.82, 145.88, 145.93, 146.00, 146.15, 146.21, 146.26, 146.61, 146.80, 147.15, 147.2, 148.82, 150.65, 153.48, 153.75, 153.93, 154.43.
Oxidation of pyrrolidinofullerene 2a. DDQ (22.4 mg, 0.10 mmol) was added in the chlorobenzene solution of 2a (10.0 mg, 0.098 mmol). The mixture was slightly warmed at 50 ºC with stirring 72 h. After cooling to rt, water and chlorobenzene were added to the solution. The organic layer was separated and evapolated. The residue was purified by column chromatography, to give pristine C60 (6.1 mg, 85%).
ACKNOWLEDGEMENTS
We thank Kyoko Inoue for the NMR measurements. This work was partly supported by a Grant-in-Aid for Young Scientist (B) (No. 24750039) from JSPS and by Health Labor Sciences Research Grants from MHLW.
References
1. a) M. Maggini, G. Scorrano, and M. Prato, J. Am. Chem. Soc., 1993, 115, 9798; CrossRef b) M. Prato and M. Maggini, Acc. Chem. Res., 1998, 31, 519. CrossRef
2. Y. Zhao, L. Gan, D. Zhou, C. Luo, and C. Huang, Chin. Sci. Bull., 1997, 42, 1360. CrossRef
3. A. Bianco, M. Maggini, and G. Scorrano, J. Am. Chem. Soc., 1996, 118, 4072. CrossRef
4. S. Filippone, E. E. Maroto, A. Martín-Domenech, M. Suárez, and N. Martín, Nature Chem., 2009, 1, 578. CrossRef
5. a) S. Campidelli, R. Deschenaux, J.-F. Eckert, D. Guillon, and J.-F. Nierengarten, Chem. Commun., 2002, 656; CrossRef J. Lenoble, N. Maringa, S. Campidelli, B. Donnio, D. Guillon, and R. Deschenaux, Org. Lett., 2006, 8, 1851. CrossRef
6. a) H. Imahori, K. Hagiwara, T. Akiyama, M. Aoki, S. Taniguchi, T. Okada, M. Shirakawa, and Y. Sakata, Chem. Phys. Lett., 1996, 263, 545; CrossRef b) P. A. Troshin, A. S. Peregudov, S. I. Troyanov, and R. N. Lyubovskaya, Russ. Chem. Bull., 2009, 57, 887. CrossRef
7. N. Ikuma, H. Yamamoto, K. Kokubo, and T. Oshima, Chem. Lett., 2014, 43, 1648. CrossRef
8. R. S. Ashraf, M. Shahid, E. Klemm, M. Al-Ibrahim, and S. Sensfuss, Macromol. Rapid Commun., 2006, 27, 1454. CrossRef
9. T. Michinobu, T. Nakanishi, J. P. Hill, M. Funahashi, and K. Ariga, J. Am. Chem. Soc., 2006, 128, 10384. CrossRef
10. MALDI-MS of 2a showed the fragment peak due to the elimination of methylstyrene (M–117) as shown in ref. 7.
11. K. Kokubo, S. Tochika, M. Kato, Y. Sol, and T. Oshima, Org. Lett., 2008, 10, 3335. CrossRef
12. a) G. A. Olah, I. Bucsi, and C. Lambert, J. Am. Chem. Soc., 1991, 113, 9388; CrossRef b) A. Iwashita, Y. Matsuo, and E. Nakamura, Angew. Chem. Int. Ed., 2007, 46, 3513. CrossRef
13. Although pristine fullerene was inactive with TfOH, fullerene derivatives having basic sites reacted with TfOH, followed by arylation of aromatic solvents. See: M. Nambo, Y. Segawa, and K. Itami, J. Am. Chem. Soc., 2011, 133, 2402. CrossRef
14. a) N. Martín, M. Altable, S. Filippone, A. Martín-Domenech, L. Echegoyen, and C. M. Cardona, Angew. Chem. Int. Ed., 2006, 45, 110; CrossRef b) S. Filippone, M. I. Barroso, A. Martín-Domenech, S. Osuna, M. Solà, and N. Martín, Chem. Eur. J., 2008, 14, 5198. CrossRef