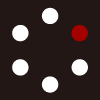
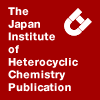
HETEROCYCLES
An International Journal for Reviews and Communications in Heterocyclic ChemistryWeb Edition ISSN: 1881-0942
Published online by The Japan Institute of Heterocyclic Chemistry
e-Journal
Full Text HTML
Received, 30th July, 2014, Accepted, 14th October, 2014, Published online, 23rd October, 2014.
DOI: 10.3987/COM-14-S(K)94
■ Lithium tert-Butoxide-Mediated Carboxylation Reactions of Unprotected Indoles and Pyrroles with Carbon Dioxide
Woo-Jin Yoo, Thanh V. Q. Nguyen, Montse Guiteras Capdevila, and Shū Kobayashi*
Department of Chemistry, The University of Tokyo, 7-3-1 Hongo, Bunkyo-ku, Tokyo 113-0033, Japan
Abstract
Unprotected indoles and pyrroles were found to undergo base-mediated carboxylation reactions under ambient pressure of carbon dioxide. It was found that this transition metal-free carboxylation reaction proceeded smoothly with the use of a large excess of LiOtBu.INTRODUCTION
The chemical incorporation of carbon dioxide (CO2) as a C1 source into organic compounds is desirable since CO2 is non-toxic, non-flammable, and a readily available gas. In particular, the carboxylation reaction of carbon-based nucleophiles with CO2 represents a facile method to access carboxylic acids and their derivatives. However, due to its thermodynamic and kinetic stability, most protocols relies on the use of reactive organometallic reagents and/or transition metal catalysts to facilitate the C-C bond forming reactions with CO2.1
The direct carboxylation of the carbon-hydrogen (C-H) bonds of arenes and heteroaromatics is attractive since the use of C-H bonds has the potential to streamline synthesis by circumventing the need for pre-activation of the nucleophile prior to the coupling event.2 The chemical fixation of CO2 with C-H bonds have been reported through the use of transition metal catalysts,3 Lewis acid-mediated Friedel-Crafts reactions,4 and base-mediated carboxylation of aromatic heterocycles bearing acidic C-H bonds.5
Indole-3-carboxylic acids are ubiquitous in bioactive natural products and pharmaceuticals.6 As such, there is interest in the development of new synthetic strategies to access these molecules efficiently and in a timely manner. Most synthetic protocols are either multi-step processes or relies on the use of pre-functionalized starting materials.7 As such, the direct carboxylation reaction of indole derivatives with readily available CO2 would represent a significant advance in this endeavour. Previously, Hattori and co-workers disclosed a dialkylaluminum chloride-mediated direct carboxylation reaction of indoles and pyrroles.4f However, the Friedel-Crafts carboxylation reaction suffered from narrow substrate scope, modest yields, and relatively high pressure of CO2. In order to address these limitations, we recently reported a simple protocol for the preparation of indole-3-carboxylic acid through a lithium tert-butoxide-mediated carboxylation reaction of indole derivatives under atmospheric pressure of CO2.5b In this paper, we will disclose a full account of the base-mediated carboxylation reaction.
RESULTS AND DISCUSSION
In order to develop an efficient synthetic protocol to access indole-3-carboxylic acids, we considered the use of electrophilic metal catalysts for the Friedel-Craft-type carboxylation reaction. Based on previous literature precedent on the use of Pd(OAc)2 as a catalyst for C-H bond functionalization of indole derivatives,8 we began our investigation by examining the carbonxylation reaction of indole under basic conditions with an atmospheric pressure of CO2 (Table 1).
When various bases were examined for the palladium-catalyzed C-3 carboxylation of indole (1a), we found that a weak carbonate base, such as K2CO3, provided the desired carboxylic acid 2a in trace amounts (entry 1). After screening several weak inorganic bases, we found that Cs2CO3 furnished 2a in a relatively good yield (entry 3). When strong bases were examined, LiOtBu was revealed to be an excellent base that facilitated the CO2 incorporating reaction in a high yield (entry 8). In addition, we investigated strong organic bases and found that these were not suitable additives (entries 9-10).
Although the optimization studies revealed the best reaction conditions for the direct carboxylation of indole (1a) under an atmospheric pressure of CO2, we found that these results were not reproducible. In order to understand this phenomenon, we profiled the carboxylation reaction and found that the yield of the desired indole-3-carboxylic acid (2a) decreased after a prolonged time period (Table 2, entries 1-6).
Based on these results, we hypothesized that 3-carboxylated indole 2a underwent a competing decarboxylative side reaction,9 and that the origin of the reproducibility problem might have been derived from this process. When we increased the amount of the base, the decarboxylation process was effectively suppressed and the carboxylated product 2a could be reliably obtained in excellent yields (entry 7). Finally, control studies revealed that Pd(OAc)2 was not necessary, and that the direct C-H carboxylation of indole (1a) found in this study was a base-mediated process.
With the optimized conditions in hand, we examined the substrate scope for the LiOtBu-mediated carboxylation of indole derivatives 1a-p under an atmospheric pressure of CO2 (Table 3).
When 5-substituted indoles 1a-g were subjected to our optimized reaction conditions, both electron-rich and -poor groups were tolerated, with the exception of an amino group (1d), to provide the desired carboxylated product in excellent yields. With 4-substituted indoles 1h-i were utilized, the CO2 fixation hardly proceeded and low chemical yields were obtained. Interestingly, when these 4-substitued ethers were replaced with a hydroxyl group, the carboxylation occurred smoothly to provide indole-3-carboxylic acid 2j in a good yield. Indoles with substitution on the 7-position (1k-1l) were also found to be amenable for the direct carboxylation reaction to furnish carboxylic acids 2k-l in good yields. Although 2-methyl indole 1m could undergo carboxylation in a good yield, electron-withdrawing substituents diminished the nucleophilic addition of indoles 1n-o to CO2. Finally, when an N-substituted indole 1p was utilized as a substrate, the desired product was not obtained. This result suggests that the free NH is essential, and that the initial deprotonation of the NH bond by LiOtBu is an important step for the carboxylation reaction.
With the discovery of a successful CO2 fixation reaction of indole derivatives using LiOtBu as a base, we examined the possibility of extending these conditions to induce the direct carboxylation of pyrroles 3a-d (Table 4).
Initially, when we subjected pyrrole (3a) to our previously optimized reaction conditions for the carboxylation reaction with indoles, the desired carboxylated product 4a was not detected (entry 1). This was due to the fact that under these conditions, pyrrole decomposed. However, when an electron-withdrawing substituent, such as a methyl ester, was introduced to the pyrrole core, the desired 2-carboxylated product 4b was obtained in a low yield (entry 2). Similarly, pyrrole 3c, bearing an imine, underwent carboxylation to provide 4c in a moderate yield (entry 3). When 2-phenylpyrrole (3d) was utilized as a substrate, a mixture of mono- and di-carboxylated products was obtained in a good yield (entry 4).
In summary, we developed a base-mediated carboxylation protocol for the efficient carboxylation of indole and pyrrole derivatives with broad substrate scope under an atmospheric pressure of CO2. It was found that the key for this CO2 fixation relies on the use of a large excess of base to prevent the reversible decarboxylation process. The direct carboxylations of other heteroaromatic C-H bonds are currently under investigation in our laboratory.
EXPERIMENTAL
General information: 1H and 13C NMR spectra were recorded on a JEOL ECX-500 and a JEOL ECX-600 in CDCl3. Chemical shifts were reported in parts per million (ppm) from tetramethylsilane using the solvent resonance as the internal standard. IR spectra were measured on a JASCO FT/IR-610 spectrometer. High-resolution mass spectrometry was carried out using a JEOL JMS-T100TD (DART). Preparative thin-layer chromatography (PTLC) was carried out using Wakogel B-5F from Wako Pure Chemical Industries, Ltd.
Reagents: Unless stated otherwise, commercial reagents were used as received. All organic solvents were purified by distillation under dry argon atmosphere. 7-Bromoindole (1g),10 4-benzyloxy-1H-indole (1i),11 1H-benzo[g]indole (1k),11 2-imino pyrrole 3c,12 and 2-phenylpyrrole (3d)13 were prepared according to literature procedures.
Representative procedure for the direct C-H carboxylation of indole derivatives 1a-p: In a dried two-necked test tube was charged with LiOtBu (160 mg, 2.00 mmol) and indole 1a (23.4 mg, 0.4 mmol). The reaction vessel was evacuated under high vacuum and the atmosphere was replace with a balloon of CO2. Then DMF (2 mL) was added and the mixture was stirred for 24 h at 100 °C. Then the result mixture was cooled and carefully quenched with a solution of HCl (2 N) and extracted with EtOAc (5x). The combined organic layers were washed with water (2x), brine (1x) and dry over MgSO4. The dried organics were concentrated under reduce pressure and the residue was purified by preparative TLC (hexane:acetone = 1:1) to afford the desired product 2a (153.0 mg, 95%) as a white solid.
Indole-3-carboxylic acid (2a). 1H NMR (DMSO-d6, 400 MHz): δ 11.87 (bs, 1H), 11.7 (bs, 1H), 7.99-7.92 (m, 2H), 7.43-7.38 (m, 1H), 7.18-7.06 (m, 2H).
The spectra data of carboxylated indoles 2a-n obtained in this study were in accordance with reported data.5b
For the derivativation of indole-3-carboxylic acids 2b and 2k: Following the above procedure, the crude reaction mixture was diluted with MeOH and cooled to 0 °C. Then SOCl2 (36 µL, 0.5 mmol) was added and stirred for 3 h at room temperature. Additional MeOH was added and the reaction mixture was heated to reflux for 6 h. The resulting mixture was quenched with H2O and extracted with EtOAc (3x). The combined organic layers were dried over MgSO4 and concentrated under reduced pressure. The resulting crude residue was purified by preparative TLC (hexane:EtOAc = 7:3) to afford the desired products 5a-b.
Methyl 5-methylindole-3-carboxylate (5a). 1H NMR (CDCl3, 400 MHz): δ 8.68 (bs, 1H), 7.98 (s, 1H), 7.77 (s, 1H), 7.20 (d, 1H, J = 6.4 Hz), 6.99 (d, 1H, J = 8.2 Hz), 3.84 (s, 3H), 2.40 (s, 3H).
Methyl benzo[g]indole-3-carboxylate (5b). 1H NMR ((CD3)2CO, 500 MHz): δ 11.8 (bs, 1H), 8.36 (d, 1H, J = 9.7 Hz), 8.25 (d, 1H, J = 9.2 Hz), 8.05 (d, 1H, J = 2.3 Hz), 7.97 (d, 1H, J = 8.05), 7.66 (d, 1H, J = 6.4 Hz) 7.56 (t, 1H, J = 6.9 Hz), 7.46 (t, 1H, J = 7.4 Hz), 3.90 (s, 3H).
The spectra data of derivatized carboxylated indoles 5a-b obtained in this study were in accordance with reported data.5b
Representative procedure for the direct C-H carboxylation of pyrrole derivatives 3a-d: In a dried two-necked test tube was charged with LiOtBu (80.0 mg, 1.00 mmol) and 2-phenylpyrrole 3d (28.6 mg, 0.2 mmol). The reaction vessel was evacuated under high vacuum and the atmosphere was replace with a balloon of CO2. Then DMF (1 mL) was added and the mixture was stirred for 24 h at 100 °C. Next, the reaction mixture was cooled to room temperature, charged with iodomethane (60 µL, 1.0 mmol), and was stirred for 2 h at 60 °C. Then the result mixture was cooled and carefully quenched a solution of HCl (2 N) and extracted with EtOAc (5x). The combined organic layers were washed with water (2x), brine (1x) and dry over MgSO4. The dried organics were concentrated under reduce pressure and the residue was purified by preparative TLC (hexane:EtOAc = 7:3) to afford the desired products 4d (16.0 mg, 37%) and 4e (25.0 mg, 46%) as yellow oils.
Methyl 1-methyl-5-phenyl-1H-pyrrole-2-carboxylate (4d). 1H NMR (CDCl3, 500 MHz): δ 7.33-7.43 (m, 5H), 6.99 (d, J = 4.0 Hz, 1H), 6.17 (d, J = 4.0 Hz, 1H), 3.85 (s, 3H), 3.80 (s, 3H).
Dimethyl 1-methyl-5-phenyl-1H-pyrrole-2,4-dicarboxylate (4e). 1H NMR (CDCl3, 500 MHz): δ 7.29-7.39 (m, 5H), 6.46 (d, J = 1.7 Hz, 1H), 3.84 (s, 3H), 3.78 (s, 3H), 3.67 (s, 3H).
The spectra data of carboxylated pyrroles 4b-d obtained in this study were in accordance with reported data.14,15
ACKNOWLEDGEMENTS
This work was partially supported by a Grant-in-Aid for Science Research from the Japan Society for the Promotion of Science (JSPS), The University of Tokyo, the Japan Science and Technology Agency (JST), and the Ministry of Education, Culture, Sports, Science and Technology (MEXT), Japan. We would like to thank Dr. X. Du for his assistance in the early stages of this work.
References
1. For representative reviews on the use CO2 for chemical transformations, see: (a) T. Sakakura, J.-C. Choi, and H. Yasuda, Chem. Rev., 2007, 107, 2365; CrossRef (b) K. Huang, C.-L. Sun, and Z.-J. Shi, Chem. Soc. Rev., 2011, 40, 2435; CrossRef (c) M. Cokoja, C. Bruckmeier, B. Rieger, W. A. Herrmann, and F. E. Kühn, Angew. Chem. Int. Ed., 2011, 50, 8510; CrossRef (d) Y. Tsuji and T. Fujihara, Chem. Commun., 2012, 48, 9956; CrossRef (e) X. Cai and B. Xie, Synthesis, 2013, 45, 3305. CrossRef
2. For representative reviews on C-H bond functionalization, please see: (a) J. Yamaguchi, A. D. Yamaguchi, and K. Itami, Angew. Chem. Int. Ed., 2012, 51, 8960; CrossRef (b) D. Y.-K. Chen and S. W. Youn, Chem. Eur. J., 2012, 18, 9452; CrossRef (c) S. H. Cho, J. Y. Kim, J. Kwak, and S. Chang, Chem. Soc. Rev., 2011, 40, 5068; CrossRef (d) C. Liu, H. Zhang, W. Shi, and A. Lei, Chem. Rev., 2011, 111, 1780; CrossRef (e) C. S. Yeung and V. M. Dong, Chem. Rev., 2011, 111, 1215. CrossRef
3. (a) I. I. F. Boogaerts and S. P. Nolan, J. Am. Chem. Soc., 2010, 132, 8858; CrossRef (b) I. I. F. Boogaerts, G. C. Fortman, M. R. L. Furst, C. S. J. Cazin, and S. P. Nolan, Angew. Chem. Int. Ed., 2010, 49, 8674; CrossRef (c) L. Zhang, J. Cheng, T. Ohishi, and Z. Hou, Angew. Chem. Int. Ed., 2010, 49, 8670; CrossRef (d) K. Ito, H. Tamashima, N. Iwasawa, and H. Kusama, J. Am. Chem. Soc., 2011, 133, 3716; CrossRef (e) H. Inomata, K. Ogata, S.-I. Fukuzawa, and Z. Hou, Org. Lett., 2012, 14, 3986. CrossRef
4. (a) J. F. Norris and J. E. Wood III, J. Am. Chem. Soc., 1940, 62, 1428; CrossRef (b) Y. Suzuki, T. Hattori, T. Okuzawa, and S. Miyano, Chem. Lett., 2002, 31, 102; CrossRef (c) G. A. Olah, B. Török, J. P. Joschek, I. Bucsi, P. M. Esteves, G. Rasul, and G. K. S. Prakash, J. Am. Chem. Soc., 2002, 124, 11379; CrossRef (d) K. Nemoto, H. Yoshida, Y. Suzuki, N. Morohashi, and T. Hattori, Chem. Lett., 2006, 35, 820; CrossRef (e) T. Iijima and T. Yamaguchi, J. Mol. Catal. A: Chem., 2008, 295, 52; CrossRef (f) K. Nemoto, S. Onozawa, N. Egusa, N. Morohashi, and T. Hattori, Tetrahedron Lett., 2009, 50, 4512; CrossRef (g) K. Nemoto, H. Yoshida, N. Egusa, N. Morohashi, and T. Hattori, J. Org. Chem., 2010, 75, 7855; CrossRef (h) K. Nemoto, S. Onozawa, M. Konno, N. Morohashi, and T. Hattori, Bull. Chem. Soc. Jpn., 2012, 85, 369. CrossRef
5. (a) O. Vechorkin, N. Hirt, and X. Hu, Org. Lett., 2010, 12, 3567; CrossRef (b) W.-J. Yoo, M. Guiteras Capdevila, X. Du, and S. Kobayashi, Org. Lett., 2012, 14, 5326. CrossRef
6. (a) Z. Yang, A. Ney, B. A. Cromer, H.-L. Ng, M. W. Parker, and J. W. Lynch, J. Neurochem., 2007, 100, 758; CrossRef (b) Y. S. Boriskin, I. A. Leneva, E.-I. Pécheur, and S. J. Polyak, Curr. Med. Chem., 2008, 15, 997; CrossRef (c) K. Hagiwara, M. Himuro, M. Hirama, and M. Inoue, Tetrahedron Lett., 2009, 50, 1035. CrossRef
7. (a) Y. Ito, K. Kobayashi, and T. Saegusa, J. Am. Chem. Soc., 1997, 99, 3532; CrossRef (b) T. Fukuda, Y. Mine, and M. Iwao, Tetrahedron, 2001, 57, 975; CrossRef (c) K. Fogassy, K. Kovács, G. M. Keserű, L. Tőke, and F. Faigl, J. Chem. Soc., Perkin Trans. 1, 2001, 1039; CrossRef (d) C. M. Park, S. Y. Kim, W. K. Park, N. S. Park, and C. M. Seong, Bioorg. Med. Chem. Lett., 2008, 18, 3844. CrossRef
8. For palladium-catalyzed direct C3 functionalization of indole derivatives under basic conditions, see: (a) M. Bandini, A. Melloni, and A. Umani-Ronchi, Org. Lett., 2004, 6, 3199; CrossRef (b) D. R. Stuart and K. Fagnou, Science, 2007, 316, 1172; CrossRef (c) Z. Zhang, Z. Hu, Z. Yu, P. Lei, H. Chi, Y. Wang, and R. He, Tetrahedron Lett., 2007, 48, 2415; CrossRef (d) F. Bellina, F. Benelli, and R. Rossi, J. Org. Chem., 2008, 73, 5529; CrossRef (e) G. Cusati and L. Djakovitch, Tetrahedron Lett., 2008, 49, 2499; CrossRef (f) Y. Gu and X.-M. Wang, Tetrahedron Lett., 2009, 50, 763; CrossRef (g) L. Ackermann and S. Barfusser, Synlett, 2009, 808; CrossRef (h) G. Yan, C. Kuang, Y. Zhang, and J. Wang, Org. Lett., 2010, 12, 1052; CrossRef (i) P. Y. Choy, C. P. Lau, and F. Y. Kwong, J. Org. Chem., 2011, 76, 80; CrossRef (j) Z. Wang, K. Li, D. Zhao, J. Lan, and J. You, Angew. Chem. Int. Ed., 2011, 50, 5365; CrossRef (k) A. D. Yamaguchi, D. Mandal, J. Yamaguchi, and K. Itami, Chem. Lett., 2011, 40, 555. CrossRef
9. For representative examples of decarboxylation of indole carboxylic acids, see: (a) E. Piers and R. K. Brown, Can. J. Chem., 1962, 40, 559; CrossRef (b) G. B. Jones and B. J. Chapman, J. Org. Chem., 1993, 58, 5558; CrossRef (c) M. Rudzki, A. Alcald-Aragonés, W. I. Dzik, N. Rodríguez, and L. Gooßen, J. Synthesis, 2012, 44, 184; (d) A. A. Vandersteen, S. O. C. Mundle, and R. Kluger, J. Org. Chem., 2012, 77, 6505. CrossRef
10. G. Bartoli and G. Palmiere, Tetrahedron Lett., 1989, 16, 2129. CrossRef
11. P. B. Smart, C. R. Oslund, A. L. Walsh, and H. M. Gelb, J. Med. Chem., 2006, 49, 2858. CrossRef
12. A. K. Upadhyaya and K. N. Mehrotra, J. Chem. Soc., Perkin Trans. 2, 1988, 957. CrossRef
13. F. Jafarpour, S. Rahiminejadan, and H. Hazrati, J. Org. Chem., 2010, 75, 3109. CrossRef
14. P. Barker, P. Gendler, and H. Rapoport, J. Org. Chem., 1978, 43, 4849. CrossRef
15. US Pat., 6 919 359, 2005.