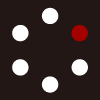
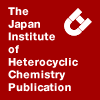
HETEROCYCLES
An International Journal for Reviews and Communications in Heterocyclic ChemistryWeb Edition ISSN: 1881-0942
Published online by The Japan Institute of Heterocyclic Chemistry
e-Journal
Full Text HTML
Received, 1st August, 2014, Accepted, 25th August, 2014, Published online, 27th August, 2014.
DOI: 10.3987/COM-14-S(K)101
■ Synthesis, Properties and Crystal Structures of 2,7,12,17-Tetraarylporphycenes
Daiki Kuzuhara,* Haruka Nakaoka, Takuya Okabe, Naoki Aratani, and Hiroko Yamada*
Graduate School of Materials Science, Nara Institute of Science and Technology, 8916-5 Takayama, Ikoma, Nara 630-0192, Japan
Abstract
We have synthesized 2,7,12,17-tetraarylporphycenes, which have phenyl (7a), p-trifluoromethylphenyl (7b) or p-methoxyphenyl (7c) groups, by McMurry coupling. The crystal structures revealed that 7a formed a herringbone-type arrangement, while 7b formed a slip-stacked structure with π-π stacking. The reduction potentials of these porphycenes depend on the attached aryl groups: –1.02 V (vs. ferrocene/ferrocenium cation) for 7b (Ar = p-C6H4CF3), –1.17 V for 7a (Ar = Ph) and –1.23 V for 7c (Ar = p-C6H4OMe), which are close to that of PC61BM (Ered = –1.08 V). These porphycenes, thus, are expected to behave as n-type semiconducting materials in OTFT and OPV devices.INTRODUCTION
Porphycene, a constitutional isomer of porphyrin, has two directly-linked bipyrrole units connected by two ethylene bridges.1 Compared with porphyrin, porphycene is more chemically stable because of removal of the highly reactive methine carbons and has a stabilized lowest unoccupied molecular orbital (LUMO) by dissolving the degeneracy of LUMO and LUMO+1 levels.2 Therefore, the highest occupied molecular orbital (HOMO)–LUMO difference of porphycene is smaller than that of porphyrin, which results in the intense and red-shifted visible/near infrared (NIR) absorption and NIR fluorescence of porphycene. These unique optical and electronic properties are suitable for photodynamic therapy (PDT)3, non-linear optics4 and protein mimic systems.5 We have succeeded in the preparation of tetrabenzoporphycenes (TBPc) from thermal-convertible precursors of bicyclo[2.2.2]octadiene (BCOD)-fused porphycene by retro Diels-Alder reaction under heating conditions.6 TBPc is a constitutional isomer of tetrabenzoporphyrin, which is known as an excellent organic semiconducting material for organic thin-film transistors (OTFTs) and organic photovoltaics (OPVs).7 We have fabricated p–n type devices consisting of the TBPc as the p-layer and [6,6]-phenyl-C61-butyric acid methyl ester (PC61BM) as the n-layer by a solution process and the power-conversion efficiency was 1.49%.7a Recently, we reported the synthesis of porphycene–diketopyrrolopyrrole conjugates which showed intense Q-bands in the NIR regions, indicating an electronic interaction between porphycene and diketopyrrolopyrrole moieties.8 From these results, we expect that porphycenes were attractive organic functional dyes for OTFT and OPV, in which the porphycene with the low-lying LUMO level could be used as n-type semiconducting materials for OTFT and OPV devices. In particular, non-fullerene n-type materials are strongly required for OPV devices. In this context, Jux and Guldi have used porphycene derivatives used as electron acceptor in the composites of graphene oxide and nano-graphene to generate a photocurrent.9 In the OTFT and OPV, the molecular packing and electronic properties of compounds directly influence the device performances. To date various 2,7,12,17-tetraarylporphycenes and their metal complexes have been reported,10,11 and their photophysical properties were well investigated to be applied to PDT as photosensitizer.12 However their crystal structures and the systematic substitution effects of aryl groups at para-position have been rarely investigated sufficiently. Herein, we describe the synthesis, crystal structures and electronic properties of a series of 2,7,12,17-tetraarylporphycenes with phenyl (7a), p-trifluorophenyl (7b) and p-methoxyphenyl (7c) groups, as candidates for new n-type semiconducting materials.
RESULTS AND DISCUSSION
2,7,12,17-Tetraphenylporphycene 7a was prepared according to the previously reported method.10 The synthetic route of 2,7,12,17-tetraaryporphycenes 7b and 7c is shown in Scheme 1. Diethyl 3-arylpyrrole-2,4-dicarboxylates 2b and 2c were synthesized from 4-trifluoromethylbenzaldehyde 1b and anisaldehyde 1c with ethyl isocyanate in 17% and 42% yields, respectively.13 Iodination of 2b and 2c at the pyrrolic α-position was performed with iodine and HIO3 in 1,2-dichloroethane under reflux conditions.6a In the case of 2b, iodination reaction smoothly proceeded to give 3b in 88% yield. When 2c was reacted under the same conditions, the yield of 3c was decreased to 66%, while iodination occurred at the pyrrolic α-position as well as the anisole moiety to give diiodo product 3c-I in 17% yield as a byproduct. The anisole moiety is well known to provide iodinated products by similar iodination conditions.14 The structure of 3c-I was confirmed by X-ray diffraction analysis (Figure 1).
Subsequently, Ullmann coupling of 3b and 3c afforded 2,2’-bipyrroles 4b and 4c in 86% and 90% yields, respectively. Decarboxylation and formylation by Vilsmeier–Haack reaction gave 5,5-diformyl-2,2’-bipyrroles 6b and 6c, respectively. A McMurry coupling of 6b and 6c resulted in the formation of porphycenes 7b and 7c in 15% and 18% yields, respectively. These compounds were characterized by 1H-NMR spectroscopy, high-resolution MS spectrometry and X-ray diffraction analysis.
In the 1H-NMR spectra of 7b and 7c, the signals of pyrrolic β-protons were observed at 9.78 and 9.63 ppm as singlet peaks, and the N-H protons were observed at 3.92 ppm and 3.77 ppm. In general, the signals of N-H protons of porphyrins appear in the range of –2 to –5 ppm due to the effect of a strong diatropic ring current of porphyrin macrocycles. Despite the same 18 π-electron structure, the N-H protons of porphycenes is drastically downfield shifted compared to those of porphyrins because the NH•••N distances in the porphycene cavities are shorter than those of porphyrins and therefore NH protons of porphycenes form stronger hydrogen-bonding interactions with neighboring nitrogen atoms.
Single crystals of 7a and 7b suitable for X-ray diffraction analysis were obtained by vapor slow diffusion of 2-propanol into chlorobenzene solution (Figure 2). The crystal structures of 7a and 7b show a highly planar macrocyclic structure of porphycene core with mean plane deviation with respect to the macrocyclic atoms of 0.04 Å for 7a and 0.05 Å for 7b. The N-N distances are 2.635(3) and 2.843(2) Å for 7a, and 2.612(3), 2.608(3), 2.863(3) and 2.865(3) Å for 7c. These distances are similar to those of 2,7,12,17-tetrapropylporphycene at 2.831 and 2.615 Å.15 The dihedral angles between the porphycene macrocycle and attached aryl groups are 32.98º and 47.82º for 7a and 28.3º, 33.6º, 37.9º and 45.5º for 7b. These angles are totally different from the 5,10,15,20-tetraarylporphyrins (TPP).16 The aryl groups of TPP are positioned almost perpendicular because of steric hindrance between aryl groups and the porphyrin macrocycle. Similarly, 9,10,19,20-tetraphenylporphycene shows the aryl groups positioned perpendicular, where dihedral angles of phenyl groups and porphycene macrocycle are 79º and 90º.17 On the other hand, in the case of β-aryl porphyrins, the dihedral angles between the β-aryl-groups and porphyrin were reported as 46-53º.18 The distances between phenyl-carbon and porphycene-carbons are 1.47 Å (ave.) for both 7a and 7b which are shorter than those of TPP and 9,10,19,20-tetraphenylporphycene (1.50 Å). These structural features indicate that despite the introduction of the aryl groups at the 2,7,12,17-positions, high planarity is retained and the aryl groups exhibit effective conjugation to porphycene macrocycles. The crystal packing of 7a shows a herringbone type arrangement. The intermolecular distance of each porphycene planes is 3.409 Å which is slightly shorter than sum of van der Waals radii of carbon atoms. On the other hand, porphycene 7b is packed as a slip-stack structure. We found that the change of the crystal structures of 7b from 7a was induced by the C–F•••F–C interactions of the trifluorobenzene moieties between neighboring molecules.19 The alternate face-to-face distances of molecules are 3.308 Å and 3.357 Å which distances decreased from that of 7a by the introduction of the CF3 moieties. This means that the introduction of the substituents at the para-positions of the phenyl moieties could be control of the crystal structures of the porphycenes. In addition, these herringbone and slip stacked structures might introduce good semiconducting properties as OTFT and OPV to 7a and 7b.
The absorption spectra of 7a, 7b and 7c in CH2Cl2 are shown in Figure 3. The absorbance peaks of 7a were observed at 376 nm and 392 nm as split Soret bands, and 584, 625 and 656 nm as Q-bands. The absorption shape of 7b was similar to 7a and the peak tops are slightly red-shifted by 2 nm at the Soret and Q-bands. On the other hand, the Soret band of 7c became one broad peak at 380 nm and Q-bands appear at 591, 632 and 662 nm. The fluorescence spectra of 7a and 7b in CH2Cl2 were observed at 669 and 732 nm, and 670 and 730 nm with fluorescence quantum efficiencies (Φem) of 19% and 26%, respectively. The Stokes shifts were 13 nm for 7a and 12 nm for 7b. On the other hand, the fluorescence of 7c was largely quenched. The fluorescence peaks were observed at 681 and 739 nm and fluorescence quantum efficiency was only 4%. The Stokes shift of 7c was 19 nm, which was slightly bigger than that of 7a and 7b. The fluorescence lifetimes of 7a, 7b and 7c were measured by the time correlated single photon counting method. All compounds exhibited the single exponential decay. Fluorescence lifetimes of 7a and 7b were 3.38 and 4.31 ns, respectively, while the fluorescence lifetime of 7c was decreased to 0.72 ns. These results revealed that the optical properties of 7a and 7b were very similar, indicating little influence of the electron-withdrawing p-trifluorophenyl moiety on the absorption and emission properties of porphycene. In contrast, the p-methoxyphenyl group clearly affected the optical properties. Nonell has reported that meta-tetrahydroxyphenyl-porphycene has a 2.3 ns fluorescence lifetime and 8.4% fluorescence quantum yield in THF.20 These results indicate that fluorescence quenching of 7c may be ascribed to introductions of the electron-rich substituents at the para-positions. Therefore, the possible reason of the fluorescent quenching of 7c is the formation of the charge-transfer structures, although the substitutional effects of the electron donating groups are not clear.
To investigate the electrochemical properties of 7a, 7b and 7c, cyclic voltammetry was measured in benzonitrile containing 0.1 M nBu4NPF6 as electrolyte at room temperature (Figure 4). All porphycenes showed one reversible oxidation and two reversible reductions in the voltage range of -1.7 to +1.0. The first reductive potentials were negative-shifted depending on the electronic nature of the arylsubstituents: -1.02 V (vs ferrocene/ferrocenium cation) for 7b (Ar = p-C6H4CF3), -1.17 V for 7a (Ar = Ph) and -1.23 V for 7c (Ar = p-C6H4OMe). The oxidation potential was also shifted by the effect of the attached aryl groups. These results indicated that the electronic properties of the porphycenes could be tuned by introducing substituents on the aryl groups. In addition, these reduction potentials are comparable to the typical n-type semiconductor PC61BM (Ered = -1.08 eV).21 The LUMO levels of 7b, 7a and 7c are estimated from the reduction potentials as 3.78, 3.63 and 3.57 eV by the ferrocene standard (4.8 eV). With these characteristics, 7a and 7b are expected to behave as the n-type semiconducting materials for OTFT and OPV.
In summary, we have succeeded in the preparation of 2,7,12,17-tetraarylporphycenes 7b and 7c with an electron-withdrawing trifluoromethyl group and an electron-donating methoxy group, respectively. The crystal structures of 7a and 7b showed highly planar structures with small dihedral angles between attached aryl groups and the macrocycles. This leads to the formation of π-π stacking with intramolecular distances within the van der Waals radii. The electrochemical properties of 7a, 7b and 7c indicated the low-lying LUMO levels were close to the PC61BM. These properties show the potential of the porphycenes to be applied to n-type organic electronics materials. The fabrication of OTFT and OPV devices with these porphycenes is now underway.
EXPERIMENTAL
1H-NMR and 13C-NMR spectra were recorded on a JNM-ECX 400 and JNM-Al 300 spectrometers using tetramethylsilane as an internal standard. ESI mass spectra were recorded on a JEOL JMS-MS T100LC spectrometer. EI mass spectra recorded on a JEOL JMS 700. UV-vis absorption spectra were recorded on a JASCO UV/VIS/NIR Spectrophotometer V-670. Fluorescence spectra and fluorescence quantum yields were obtained on a Hamamatsu Absolute PL Quantum Yield Measurement System C9920-02. CV and DPV measurements were conducted in a solution of 0.1 M nBu4NPF6 in dry benzonitrile at room temperature in an argon-filled cell. Scan rate was 100 mV s-1. A glassy carbon electrode and a Pt wire were used as working and counter electrodes, respectively. An Ag/AgNO3 electrode was used as a reference electrode, which was normalized with the half-wave potential of ferrocene/ferrocenium cation (Fc/Fc+) redox couple. TLC and gravity column chromatography were performed on Art. 5554 (Merck KGaA) plates and silica gel 60N (Kanto Chemical), respectively. All solvents and chemicals were reagent-grade quality, obtained commercially, and used without further purification except as noted. For spectral measurements, spectral-grade dichloromethane was purchased from Nacalai Tesque. The dry-benzonitrile was prepared by vacuum distillation with P2O5. X-Ray crystallographic data were recorded at 100 K on a Rigaku CCD detector (Saturn 724) mounted on a Rigaku rotating anode X−ray generator (MicroMax−007HF) using Mo−Kα radiation from the corresponding set of confocal optics. The diffraction data were processed with CrystalClear on a Rigaku program and the structures were solved by direct methods and refined on F2 by full−matrix least−squares using the CrystalStructure and SHELXL-97.22 CCDC: 1016106, 1016105 and 1016104 contain supplementary crystallographic data for 3c-I, 7a and 7b respectively. These data can be obtained free of charge from the Cambridge Crystallographic Data Centre via www.ccdc.cam.ac.uk/data_request/cif.Diethyl
Diethyl 3-(4-(trifluoromethyl)phenyl)-1H-pyrrole-2,4-dicarboxylate (2b)
To a solution of ethyl isocyanoacetate (10.9 mL, 100 mmol) and 1,8-diazabicyclo[5.4.0]undec-7-ene (DBU) (14.9 mL, 100 mmol) in THF (150 mL) was added dropwise a solution of p-trifluoromethylbenzaldehyde (6.7 mL, 50 mmol) in THF (50 mL) at 50 ºC under an argon atmosphere. After stirring for 10 h at the same temperature, the reaction was quenched with 1 M HCl. After the removal of the solvent, the residue was extracted with EtOAc. The extract was washed with water and brine, and then dried over Na2SO4. The crude product was purified by silica gel column chromatography (EtOAc:hexane = 3:7). Recrystallization from CHCl3/hexane gave 2b as an off-white solid. Yield: 18% (3.1 g, 8.8 mmol). 1H NMR (400 MHz, CDCl3) δ = 9.80 (brs, 1 H), 7.60 (AA’BB’, 2H), 7.60 (d, 2H, J = 3.7 Hz, pyrrolic α-H), 7.44 (AA’BB’, 2 H), 4.15-4.09 (q+q, 2H+2H, -CO2Et), 1.11 (t, 3H, J = 7.3 Hz, -CO2Et), 1.04 (t, 3H, J = 7.3 Hz, -CO2Et); 13C NMR (100 MHz, CDCl3) δ = 163.70, 160.83, 137.97, 130.78, 130.67, 129.31 (q, J = 33 Hz), 127.10, 124.50 (q, J = 271 Hz, CF3), 124.00 (q, J = 4 Hz), 121.26, 117.25, 60.90, 60.08, 14.04, 13.83; HRMS (ESI): m/z = 378.0929. calcd. for C17H16F3NO4Na: 378.0929 [M+Na]+.
Diethyl 5-iodo-3-(4-(trifluoromethyl)phenyl)-1H-pyrrole-2,4-dicarboxylate (3b)
A mixture of 2b (3.0 g, 8.4 mmol), iodine (2.3 g, 9.3 mmol), HIO3 (0.37 g, 2.1 mmol), AcOH (10 mL) and H2O (1.5 mL) in 1,2-dichloroethane (25 mL) was refluxed for 10 h. After cooling to room temperature, the reaction was quenched with an aqueous NaHSO3. The separated organic layer was washed with water, saturated aqueous NaHCO3 and brine, and then dried over Na2SO4. The crude product was recrystallized from CHCl3/hexane to give 3b as a colorless needle. Yield: 88% (3.6 g, 7.4 mmol). 1H NMR (400 MHz, CDCl3) δ = 9.93 (brs, 1 H,), 7.59 (AA’BB’, 2H), 7.36 (AA’BB’, 2H), 4.13 (q, 2H, J = 7.3 Hz, -CO2Et), 4.05 (q, 2H, J = 7.3 Hz, -CO2Et), 1.02 (t, 3H, J = 7.3 Hz, -CO2Et), 0.96 (t, 3H, J = 7.3 Hz, -CO2Et); 13C NMR (100 MHz, CDCl3) δ = 162.88, 159.91, 138.22, 131.73, 130.33, 129.50 (q, J = 33 Hz), 125.25, 124.42 (q, J = 271 Hz, CF3), 124.08 (q, J = 4 Hz), 120.95, 78.03, 61.44, 60.88, 13.86, 12.94; HRMS (ESI): m/z = 503.9896. calcd. for C17H15F3NO4 Na: 503.9895 [M+Na]+.
Tetraethyl 4,4'-bis(4-(trifluoromethyl)phenyl)-1H,1'H-[2,2'-bipyrrole]-3,3',5,5'-tetracarboxylate (4b)
A mixture of 3b (3.4 g, 7.0 mmol) and copper powder (3.0 g) in toluene (20 mL) was refluxed for 12 h under an argon atmosphere. After cooling to room temperature, the precipitate was filtrated off on Celite pad and washed well with toluene. The filtrate was concentrated under a reduced pressure. The crude product was recrystallized from CH2Cl2/hexane to give 4b as white solids. Yield: 90% (2.2 g, 3.2 mmol). 1H NMR (400 MHz, CDCl3) δ = 14.40 (brs, 2H, -NH), 7.63 (AA’BB’, 4H), 7.39 (AA’BB’, 4H), 4.17 (q, 4H, J = 7.3 Hz, -CO2Et), 4.03 (q, 4H, J = 7.3 Hz, -CO2Et), 1.01 (t, 6H, J = 7.3 Hz, -CO2Et), 0.73 (t, 6H, J = 7.3 Hz, -CO2Et); 13C NMR (100 MHz, CDCl3) δ = 167.29, 160.15, 139.84, 132.89, 130.14, 129.43, 129.26 (q, J = 33 Hz, -CF3), 124.50 (q, J = 271 Hz, CF3), 124.14 (q, J = 4 Hz), 120.75, 113.94, 61.25, 60.40, 13.80, 13.60; HRMS (ESI): m/z = 731.1804. calcd. for C34H30F3N2O8Na: 731.1804 [M+Na]+.
4,4'-Bis(4-(trifluoromethyl)phenyl)-2,2'-bipyrrole (5b)
A mixture of 4b (2.12 g, 3.0 mmol) and NaOH (0.6 g, 15 mmol) in ethylene glycol (35 mL) was heated to 170 ºC for 1.5 h under an argon atmosphere. After cooling to room temperature, the reaction mixture was diluted with water. The appeared precipitate was corrected by filtration and washed with water to give desired product 5b (0.54 g) as white solids. The filtrate was extracted with CHCl3 and the combined organic layer was washed with water and brine, and then dried over Na2SO4. After removal of the solvent, the crude product was recrystallization from CH2Cl2/hexane to give 5b as gray solids. Total yield was 59% (0.74 g, 1.77 mmol). 1H NMR (400 MHz, DMSO-d6) δ = 11.39 (brs, 2H, -NH), 7.71 (AA’BB’, 4H), 7.62 (AA’BB’, 4H), 7.39 (m, 2H), 6.75 (m, 2H). 13C NMR spectrum was not recorded because of poor solubility; HRMS (EI): m/z = 420.1061. calcd for C22H14F6N2 : 420.1046 [M]+.
4,4'-Bis(4-(trifluoromethyl)phenyl)-1H,1'H-[2,2'-bipyrrole]-5,5'-dicarbaldehyde (6b)
To a solution of bipyrrole 5b (0.42 g, 1.0 mmol) and DMF (5.0 mL) in CH2Cl2 (15 mL) was slowly added POCl3 (0.37 mL, 4.0 mmol) at room temperature under an argon atmosphere. The reaction mixture was refluxed for 2 h. After cooling to room temperature, aqueous NaOAc was carefully added. The resulting mixture was refluxed for another 1 h. The organic layer was separated and the solvent was concentrated under a reduced pressure. The crude product was rinsed with MeOH to give 6b as greenish yellow solids. Yield: 93% (0.44 g, 0.93 mmol). 1H NMR (400 MHz, DMSO-d6) δ = 12.61 (brs, 2H, NH-), 9.67 (s, 2H, -CHO), 8.04 (AA’BB’, 4H), 7.74 (AA’BB’, 4H), 7.20 (s, 2H). 13C NMR spectrum was not recorded because of poor solubility; HRMS (EI): m/z = 476.0959. calcd for C24H14F6N2O2 : 476.0956 [M]+.
2,7,12,17-Tetra(p-trifluoromethylphenyl)-porphycene (7b)
TiCl4 (2.0 mL, 18 mmol) was added dropwise to a THF (75 mL) solution containing Zn dust (2.46 g) and CuCl (0.15 g, 1.5 mmol) at room temperature under an argon atmosphere, and then the mixture was refluxed for 2 h. Subsequently a solution of 6b (357 mg, 0.75 mmol) in THF (100 mL) was added dropwise to the boiling reaction mixture. The mixture was stirred at same temperature for another 1 h. After cooling to 0 ºC, an aqueous 10% K2CO3 solution (200 mL) was added dropwise. The precipitate was filtrated off with Celite pad and was washed with CHCl3. The combined organic layer was then dried with Na2SO4, and the solution was removed under a reduced pressure. The crude product was purified by alumina column chromatography (CHCl3) and then silica gel column chromatography (CH2Cl2). Recrystallization from CH2Cl2/MeOH provided 7b as a purple powder. Yield: 15% (49 mg, 0.055 mmol). 1H NMR (400 MHz, CDCl3) δ = 9.92 (s, 4H, meso), 9.78 (s, 4H, β), 8.47-8.44 (AA’BB’, 8H), 8.11-8.08 (AA’BB’, 8H), 3.92 (brs, 2H, -NH); 13C NMR spectrum was not recorded because of poor solubility; HRMS (MALDI-TOF) : m/z = 887.2026. calcd for C48H27N4F12 : 887.2039 [M + H]+.
Diethyl 3-(4-methoxyphenyl)-1H-pyrrole-2,4-dicarboxylate (2c)
To a solution of ethyl isocyanoacetate (10.9 mL, 100 mmol) and DBU (14.9 mL, 100 mmol) in THF (150 mL) was added dropwise a solution of 4-anisaldehyde 1c (6.0 mL, 49.6 mmol) in THF (50 mL) at 60 ºC under an argon atmosphere. After stirring for 4 h at the same temperature, the reaction was quenched with 1 M HCl (100 mL). After removal of the solvent, the residue was extracted with EtOAc. The extract was washed with 1 M HCl, water and brine, and then dried over Na2SO4. After removal of the solvent, the crude product was purified by silica gel column chromatography (CH2Cl2) to give 2c as yellow viscous oil. Yield: 33% (5.19 g, 16.4 mmol). 1H NMR (400 MHz, CDCl3) δ = 10.05 (brs, 1H, -NH), 7.54 (d, 1H, J = 3.1 Hz, pyrrolic α-H), 7.28 (AA’BB’, 2H), 6.99 (AA’BB’, 2H), 4.18-4.12 (q+q, 2H+2H, -CO2Et), 3.83 (s, 3H, -OMe), 1.17 (t, 3H, J = 7.3 Hz, -CO2Et), 1.10 (t, 3H, J = 7.3 Hz, -CO2Et); 13C NMR (100 MHz, CDCl3) δ = 164.14, 161.31, 158.89, 132.44, 131.53, 127.38, 125.89, 120.91, 116.87, 112.54, 60.64, 59.87, 55.28, 14.26, 14.07; HRMS (ESI): m/z = 340.11609. calcd. for C17H19NO5Na : 340.11724 [M+Na]+.
Diethyl 5-iodo-3-(4-methoxyphenyl)-1H-pyrrole-2,4-dicarboxylate (3c)
A solution of 2c (5.60 g, 17.7 mmol), iodine (4.50 g, 17.7 mmol), HIO3 (0.82 g, 4.65 mmol), AcOH (23 mL) and H2O (3.0 mL) in 1,2-dichloroethane (56 mL) was refluxed for 18 h. After cooling to room temperature, the reaction was quenched with aqueous NaHSO3. The separated organic layer was washed with water, saturated aqueous NaHCO3 and brine, and then dried over Na2SO4. The solution was removed under a reduced pressure. The crude product was purified by silica gel column chromatography (CH2Cl2). As a result, the crude product was isolated to 3c and 3c-II. Finally, 2c and 2c-I were recrystallized from CHCl3/hexane, respectively.
3c: Yield: 62% (4.85 g, 10.9 mmol); 1H NMR (400 MHz, DMSO-d6) δ = 12.88 (brs, 1H, -NH), 7.09 (AA’BB’, 2H), 6.86 (AA’BB’, 2H), 4.00 (q, 2H, J = 7.3 Hz, -CO2Et), 3.92 (q, 2H, J = 7.3 Hz, -CO2Et), 3.76 (s, 3H, -OCH3), 1.1 (t, 3H, J = 7.3 Hz, -CO2Et), 0.92 (t, 3H, J = 7.3 Hz, -CO2Et); 13C NMR (100 MHz, DMSO-d6) δ =163.58, 159.77, 158.80, 132.95, 131.61, 126.68, 124.61, 120.85, 112.82, 81.94, 60.27, 59.89, 55.56, 14.39, 14.22; HRMS (ESI): m/z = 466.01273. calcd. for C17H18NO5Na: 466.0.1230 [M+Na]+.
3c-I: yield: 17% (1.76 g, 3.1 mmol), 1H NMR (400 MHz, DMSO-d6) δ = 12.97 (brs, 1H, -NH-), 7.57 (m, 1H), 7.17 (m, 1H), 6.94 (m, 1H), 4.06 (q, 2H, J = 7.3 Hz, -CO2Et), 3.98 (q, 2H, J = 7.3 Hz, -CO2Et), 3.84 (s, 3H, -OMe), 1.07 (t, 3H, J = 7.3 Hz, -CO2Et), 0.99 (t, 3H, J = 7.3 Hz, -CO2Et); 13C NMR (100 MHz, DMSO-d6) δ = 163.37, 159.70, 157.26, 140.71, 132.04, 131.19, 128.79, 124.81, 120.48, 110.40, 84.60, 82.48, 60.41, 59.96, 56.91, 14.45, 14.31; HRMS (ESI): m/z = 591.90938. calcd. for C17H17I2NO5Na : 591.91051 [M+Na]+. CCDC No.
Tetraethyl 4,4'-bis(4-methoxyphenyl)-1H,1'H-[2,2'-bipyrrole]-3,3',5,5'-tetracarboxylate (4c)
A mixture of 3c (4.51 g, 10.2 mmol) and Cu (4.33 g) in toluene (21 mL) was refluxed for 20 h under an argon atmosphere. The precipitate was filtered off on Celite pad and washed with CH2Cl2. The filtrate was concentrated under a reduced pressure. The crude product was purified by silica gel column chromatography (CH2Cl2) and recrystallization from CH2Cl2/MeOH to give 4c as white solids. Yield: 90% (2.90 g, 4.59 mmol). 1H NMR (400 MHz, CD2Cl2) δ = 14.00 (brs, 2H, -NH), 7.17 (AA’BB’, 4H), 6.89 (AA’BB’, 4H), 4.15 (q, 4H, J = 7.3 Hz, -CO2Et), 4.04 (q, 4H, J = 7.3 Hz, -CO2Et), 3.83 (s, 6H, -OMe), 1.14 (t, 6H, J = 7.3 Hz, -CO2Et), 0.82 (t, 6H, J = 7.3 Hz, -CO2Et); 13C NMR (100 MHz, CD2Cl2) δ = 168.22, 160.63, 159.25, 134.34, 131.32, 129.38, 127.99, 120.77, 114.51, 112.85, 61.54, 60.87, 55.64, 14.18, 13.48; HRMS (ESI): m/z = 655.22676. calcd. for C34H36N2O10Na: 655.22544 [M+Na]+.
4,4'-Bis(4-methoxyphenyl)-2,2'-bipyrrole (5c)
A mixture of 4c (2.53 g, 4.0 mmol) and NaOH (1.04 g, 26.0 mmol) in ethylene glycol (52 mL) was heated at 170 ºC for 2 h under an argon atmosphere. The reaction mixture was diluted with cold water. The appeared precipitate was filtrated and washed with water, hexane and MeOH. The residue was dried in vacuo with P2O5 to give product as gray solids. This product was used for the next step without further purification. Yield: 92% (1.28 g, 3.7 mmol). 1H NMR (400 MHz, DMSO-d6) δ = 11.00 (brs, 2H, -NH), 7.41 (AA’BB’, 4H), 7.06 (m, 2H), 6.87 (AA’BB’, 4H), 6.54 (m, 2H), 3.75 (s, 6H, -OMe); 13C NMR (400 MHz, DMSO-d6) δ = 156.84, 128.55, 126.67, 125.24, 123.60, 113.92, 113.54, 100.23, 54.89; HRMS (ESI) m/z = 345.16030. calcd for C22H21N2O2: 345.16061 [M+H]+.
4,4'-Bis(4-methoxyphenyl)-1H,1'H-[2,2'-bipyrrole]-5,5'-dicarbaldehyde (6c)
To a solution of 5c (1.20 g, 3.5 mmol) in DMF (50 mL) was slowly added POCl3 (1.6 mL, 17 mmol) at 0 °C under an argon atmosphere. The reaction mixture was refluxed for 2 h. After cooling to room temperature, aqueous NaOAc (4.0 g in 54 mL) was carefully added. The mixture was refluxed for another 1 h. The solution was poured into cold water and then the appeared precipitate was filtrated and rinsed with water, hexane and MeOH. The residue was dried in vacuo with P2O5 to give 6c as yellowish green solids. Yield: 87% (1.21 g, 3.0 mmol). 1H NMR (400 MHz, DMSO-d6) δ = 12.34 (brs, 2H, -NH), 9.57 (s, 2H, -CHO), 7.53 (AA’BB’, 4H), 7.12 (s, 2H), 7.05 (AA’BB’, 4H), 3.81 (s, 6H, -OMe); 13C NMR (400 MHz, DMSO-d6) δ = 178.42, 158.97, 136.22, 130.05, 129.93, 128.73, 125.47, 114.21, 109.92, 55.10; HRMS (ESI): m/z = 401.15013. calcd for C24H21N2O4: 401.15010 [M+H]+.
2,7,12,17-Tetra(p-methoxyphenyl)-porphycene (7c)
TiCl4 (2.80 mL, 25 mmol) was added dropwise to a THF (100 mL) solution containing Zn dust (3.33 g) and CuCl (0.20 g, 2.0 mmol) at room temperature under an argon atmosphere, and then the mixture was refluxed for 3 h. Subsequently a solution of 6c (373 mg, 0.93 mmol) in THF (150 mL) was added dropwise to the boiling reaction mixture. The mixture was stirred at same temperature for another 1 h. After cooling to 0 ºC, an aqueous 10% K2CO3 solution (120 mL) was added dropwise. The precipitate was filtrated off on Celite pad and washed with CH2Cl2. The combined organic layer was then dried with Na2SO4, and the solution was removed under reduced pressure. The crude product was purified by alumina column chromatography (CH2Cl2) and then silica gel column chromatography (hexane/CH2Cl2 = 9:1 to 1:1). Recrystallization from CHCl3/MeOH provided 7c as purple powders. Yield: 15% (51 mg, 0.07 mmol). 1H NMR (400 MHz, CDCl3) δ = 9.90 (s, 4H), 9.63 (s, 4H) 8.28 (AA’BB’, 8H), 7.36 (AA’BB’, 8H), 4.06 (s, 12H, -OMe), 3.77 (brs, 2H, -NH); 13C NMR spectrum was not recorded because of poor solubility; HRMS (ESI): m/z = 757.27907. calcd for C48H38N4O4Na : 757.27948 [M+Na]+.
ACKNOWLEDGEMENTS
This work was supported by a Grant-in-Aid (No. 25288092 to H.Y.), the Green Photonics Project in NAIST and the program for promoting the enhancement of research universities in NAIST sponsored by the Ministry of Education, Culture, Sports, Science and Technology, Japan. We acknowledge S. Katao for X-ray diffraction analysis and the Nippon Synthetic Chem. Ind. (Osaka, Japan) for the gift of ethyl isocyanoacetate, which was used for the preparation of the starting pyrroles.
References
1. E. Vogel, M. Köcher, H. Schmickler, and J. Lex, Angew. Chem., Int. Ed. Engl., 1986, 25, 257. CrossRef
2. J. Waluk, M. Müller, P. Swiderek, M. Köcher, E. Vogel, G. Hohlneicher, and J. Michl, J. Am. Chem. Soc., 1991, 113, 5511. CrossRef
3. a) M. García-Díaz, D. Sanchez-Gárcía, J. Soriano, M. L. Sagristá, M. Mora, Á. Villanueva, J. C. Stockert, M. Cañete, and S. Nonell, Med. Chem. Commun., 2011, 2, 616; CrossRef b) X. Ragàs, D. Sanchez-Gárcía, R. Ruiz-González, T. Dai, M. Agut, M. R. Hamblin, and S. Nonell, J. Med. Chem., 2010, 53, 7796; CrossRef c) C. Richert, J. M. Wessels, M. Müller, M. Kisters, T. Benninghaus, and A. E. Goetz, J. Med. Chem., 1994, 37, 279; d) V. Gottfried, R. Davidi, C. Averbuj, and S. Kimel, J. Photochem. Photobio. B, 1995, 30, 115. CrossRef
4. (a) J. Arnbjerg, A. Jimeńez-Banzo, M. J. Paterson, S. Nonell, J. I. Borrell, O. Christiansen, and P. R. Ogilby, J. Am. Chem. Soc., 2007, 129, 5188; CrossRef (b) T. Sarma, P. K. Panda, P. T. Anusha, and S. V. Rao, Org. Lett., 2011, 13, 188; CrossRef (c) S. V. Rao, T. S. Prashant, D. Swine, T. Sarma, P. K. Panda, and S. P. Tewari, Chem. Phys. Lett., 2011, 514, 98. CrossRef
5. a) T. Matsuo, D. Murata, Y. Hisaeda, H. Hori, and T. Hayashi, J. Am. Chem. Soc., 2007, 129, 12906; CrossRef b) T. Hayashi, D. Murata, M. Makino, H. Sugimoto, T. Matsuo, H. Sato, Y. Shiro, and Y. Hisaeda, Inorg. Chem., 2006, 45, 10530; CrossRef c) T. Matsuo, T. Tsuruta, K. Maehara, H. Sato, Y. Hisaeda, and T. Hayashi, Inorg. Chem., 2005, 44, 9391; CrossRef d) T. Matsuo, H. Dejima, S. Hirota, D. Murata, H. Sato, T. Ikegami, H. Hori, Y. Hisaeda, and T. Hayashi, J. Am. Chem. Soc., 2004, 126, 16007; CrossRef e) T. Hayashi, H. Dejima, T. Matsuo, H. Sato, D. Murata, and Y. Hisaeda, J. Am. Chem. Soc., 2002, 124, 11226. CrossRef
6. a) D. Kuzuhara, H. Yamada, S. Mori, T. Okujima, and H. Uno, J. Porphyrins Phthalocyanines, 2011, 15, 930; CrossRef (b) D. Kuzuhara, H. Yamada, K. Yano, T. Okujima, S. Mori, and H. Uno, Chem. Eur. J. 2011, 17, 3376; CrossRef c) D. Kuzuhara, J. Mack, H. Yamada, T. Okujima, N. Ono, and N. Kobayashi, Chem. Eur. J., 2009, 15, 10060. CrossRef
7. a) H. Saeki, O. Kurimoto, H. Nakaoka, M. Misaki, D. Kuzuhara, H. Yamada, K. Ishida, and Y. Ueda, J. Mater. Chem. C, 2014, 2, 5357; CrossRef (b) H. Saeki, M. Misaki, D. Kuzuhara, H. Yamada, and Y. Ueda, Jpn. J. Appl. Phys., 2013, 52, 111601; CrossRef (c) H. Saeki, O. Kurimoto, M. Misaki, D. Kuzuhara, H. Yamada, and Y. Ueda, Appl. Phys. Express, 2013, 6, 035601. CrossRef
8. T. Okabe, D. Kuzuhara, M. Suzuki, N. Aratani, and H. Yamada, Org. Lett., 2014, 16, 3508. CrossRef
9. (a) W. Brenner, J. Malig, R. D. Costa, D. M. Guldi, and N. Jux, Adv. Mater., 2012, 25, 2314; CrossRef (b) R. D. Costa, J. Malig, W. Brenner, N. Jux, and D. M. Guldi, Adv. Mater., 2013, 25, 2600. CrossRef
10. S. Nonell, N. Bou, J. I. Borrell, J. Teixidó, A. Villanueva, A. Juarranz, and M. Cañete, Tetrahedron Lett., 1995, 36, 3405. CrossRef
11. a) W. Brenner, J. Malig, C. Oelsner, D. M. Guldi, and N. Jux, J. Porphyrins Phthalocyanines, 2012, 16, 651; CrossRef b) G. Anguera, M. C. Llinàs, X. Batllori, and D. Sanchez-Gárcía, J. Porphyrins Phthalocyanines, 2011, 15, 865; CrossRef c) O. Arad, N. Rubio, D. Sanchez-Gárcía, J. I. Borrell, and S. Nonell, J. Porphyrins Phthalocyanines, 2009, 13, 651; d) M. Stępień, B. Donnio, and J. L. Sessler, Chem. Eur. J., 2007, 13, 6853. CrossRef
12. N. Rubio, V. Martínez-Junza, J. Estruga, J. I. Borrell, M. Mora, M. L. Sagristá, and S. Nonell, J. Porphyrins Phthalocyanines, 2009, 13, 99. CrossRef
13. M. Suzuki, M. Miyoshi, and K. Matsumoto, J. Org. Chem., 1974, 39, 1980. CrossRef
14. S. Adimurthy, G. Ramachandraish, P. K. Ghosh, and A. V. Bedekar, Tetrahedron Lett., 2003, 44, 5099. CrossRef
15. E. Vogel, M. Balci, K. Pramod, P. Koch, J. Lex, and O. Ermer, Angew. Chem., Int. Ed. Engl., 1987, 26, 928. CrossRef
16. K. Kano, K. Fukuda, H. Wakami, R. Nishiyabu, and R. F. Pasternack, J. Am. Chem., Soc., 2000, 122, 7494. CrossRef
17. K. S. Anju, S. Ramakrishnan, A. P. Thomas, E. Suresh, and A. Srinivasan, Org. Lett., 2008, 10, 5545. CrossRef
18. a) Y. Yamamoto, S. Tokuji, T. Tanaka, H. Yorimitsu, and A. Osuka, Asian J. Org. Chem., 2013, 2, 320; b) Y. Kawamata, S. Tokuji, H. Yorimitsu, and A. Osuka, Angew. Chem. Int. Ed., 2011, 50, 8867. CrossRef
19. R. J. Baker, P. E. Colavita, D. M. Murphy, J. A. Platts, and J. D. Wallis, J. Phys. Chem. A, 2012, 116, 1435. CrossRef
20. M. García-Díaz, D. Sánchez-Gárcía, J. Soriano, M. L. Sagristà, M. Mora, Á. Villanueva, J. C. Stockert, M. Cañete, and S. Nonell, Med. Chem. Commun., 2011, 2, 616. CrossRef
21. F. B. Kooistra, V. D. Mihailetchi, L. M. Popescu, D. Kronholm, P. W. Blom, and J. C. Hummelen, Chem. Mater., 2006, 18, 3068. CrossRef
22. SHELXL-97, program for refinement of crystal structures from diffraction data, University of Göttingen, Göttingen, Germany; "A short history of SHELX". G. M. Sheldrick, Acta Cryst., 2008, A64, 112.