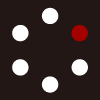
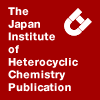
HETEROCYCLES
An International Journal for Reviews and Communications in Heterocyclic ChemistryWeb Edition ISSN: 1881-0942
Published online by The Japan Institute of Heterocyclic Chemistry
e-Journal
Full Text HTML
Received, 27th August, 2014, Accepted, 10th September, 2014, Published online, 19th September, 2014.
DOI: 10.3987/COM-14-S(K)109
■ Synthesis and Structural Analysis of Nine-Membered Enyne Nitrogen Heterocycles
Kazunobu Igawa, Takeshi Kawabata, Kazuhiro Uehara, and Katsuhiko Tomooka*
Institute for Materials Chemistry and Engineering (IMCE), Kyushu University, 6-1, Kasuga-koen, Kasuga, Fukuoka 816-8580, Japan
Abstract
An efficient approach to the nine-membered enyne nitrogen heterocycle 3 is presented. 1H NMR analysis reveals that both 3a (R = H, X = Ts) and 3b (R = Me, X = Ts) exhibit significant labile planar chirality in solution at ambient temperature. The X-ray analysis of 3b shows that the enantiotopic faces of the alkene are differentiated by the alkyne moiety in the solid state.Medium-sized heterocycles containing unsaturated carbon-carbon bonds have attracted significant interest among both synthetic and structural organic chemists because of their unique conformational and chemical properties, made possible by the deformation and strain in their unsaturated carbon-carbon bonds.1 Recently, we reported the novel 3-aza-5-[7]orthocyclophene 1, containing a deformed (E)-alkene in the ansa chain, that showed stable planar chirality along with unique alkene reactivity owing to its strained cyclic structure.2 We later reported the synthesis and stereochemical analysis of the cyclophyne analogue 2, containing an alkyne instead of the (E)-alkene of 1.3 Cyclophyne 2 was found to possess significant labile planar chirality at ambient temperature.
In connection with our research on the chemistry of medium-sized nitrogen heterocycles,4 we became interested in the novel nine-membered enyne cyclic sulfonamide 3 (X = Ts), a congener of 2 containing a (Z)-alkene instead of a benzene ring. Herein, we report the synthesis and stereochemical analysis of 3. Scheme 1 depicts the retrosynthetic strategy. We planned to construct the strained nine-membered skeleton of 3 by C–N bond formation via the intramolecular Mitsunobu reaction of i,5,6 which can be prepared by the Seyferth-Gilbert alkynylation of aldehyde iii or its equivalent using the Ohira-Bestmann reagent,7 followed by hydroxymethylation.
The synthesis of 3a (R = H) began from our previously reported ε-lactam 4a (Scheme 2).4b DIBAL reduction of 4a gave hemiaminal 5a, a tautomer of aldehyde iii (R = H, X = Ts). The reaction of 5a with the Ohira-Bestmann reagent afforded enyne 6a in 90% yield (two steps from 4a). Hydroxymethylation of the terminal alkyne in 6a was performed by an acylation and reduction sequence in 74% yield over two steps. The resulting 7a was subjected to an intramolecular Mitsunobu reaction; treating 7a with DEAD and PPh3 in THF at 0 °C to rt under high-dilution conditions (0.01 M) successfully provided the desired cyclic enyne amide 3a in good yield (80%).8
The intramolecular Mitsunobu reaction approach was also applicable to the synthesis of methyl-substituted analogue 3b (R = Me) (Scheme 3). The requisite key intermediate aldehyde 5b [≡ iii (R = Me, X = Ts)] was prepared from nerol in three steps: (i) Mitsunobu reaction with methyl N-(p-toluenesulfonyl)carbamate (93%), (ii) group-selective epoxidation of the trisubstituted alkene moiety with mCPBA (96%), and (iii) oxidative cleavage of the epoxide with orthoperiodic acid (97%). Similarly to the synthesis of 7a from 5a, alkynylation of 5b followed by hydroxymethylation provided 7b, albeit in moderate chemical yield. The intramolecular Mitsunobu reaction of 7b proceeded smoothly and provided cyclic enyne sulfonamide 3b in excellent yield (91%).9 These results clearly show the efficiency of C–N bond formation by the Mitsunobu reaction in the construction of the strained cyclic skeletons of these nitrogen heterocycles.
Sulfonamide 3b afforded a crystal suitable for X-ray analysis, which revealed that the alkyne moiety is located on the outside of the plane of the alkene.10 Hence, the enantiotopic faces of the alkene are differentiated (Figure 1), and 3b possesses planar chirality in the solid state.
Moreover, the alkyne moiety is significantly bent, with C2–C3–C4 and C3–C4–C5 bond angles of 166° and 163°, respectively.
The 1H NMR spectrum of 3a in CDCl3 at ambient temperature showed a single set of geminal methylene protons alpha to the nitrogen atom. In contrast, a similar analysis of 3b showed two sets of nonequivalent geminal methylene protons alpha to the nitrogen atom, suggesting that the aforementioned chiral conformations are maintained to some extent, even in solution and on the NMR time scale. The activation free energy for the racemization of 3a and 3b were estimated by DFT calculations as ΔG‡ = 9.9 kcal mol–1, and ΔG‡ = 12.3 kcal mol–1 at 25 °C, respectively (Figure 2).11 From these data, the half-lives of the optical activity (t1/2) of 3a and 3b at 25 °C were calculated as 2 × 10–6 s and 5 × 10–5 s, respectively. These results show that i) 3a and 3b do not have isolable enantiomers in a practical sense, ii) the planar chirality of 3a and 3b is significantly less stable than that of cyclophyne analogue 2a (X = Ts) (ΔG‡ = 15.1 kcal mol–1, t1/2 = 6 × 10–3 s at 25 °C), and iii) the substituent R on the alkene moiety has a certain level of influence on the stereochemical stability of 3.12
In summary, we have described an efficient approach to the nine-membered enyne nitrogen heterocycles 3a and 3b. The newly synthesized compounds showed fairly labile planar chirality in solution at ambient temperature; their enantiomers are unisolable in a practical sense. Further detailed studies on the relationship between the structures and stereochemical stability of these enyne heterocycles and their synthetic applications are in progress.
ACKNOWLEDGEMENTS
This research was supported by JSPS KAKENHI Grant Number 24350048, and MEXT Project of Integrated Research on Chemical Synthesis.
References
1. General reviews for medium-sized cyclic compounds containing unsaturated carbon-carbon bonds, see: (a) M. Nakagawa, 'The Chemistry of the Carbon-Carbon Triple Bond,' part 2, ed. by S. Patai, John Wiley & Sons, New York, 1978, pp. 636-654; (b) M. Nakazaki, K. Yamamoto, and K. Naemura, Top. Curr. Chem., 1984, 125, 1; CrossRef (c) Y. Tobe and M. Sonoda, 'Modern Cyclophane Chemistry,' ed. by R. Gleiter and H. Hopf, Wiley-VCH, Weinheim, 2004, Chap. 1.
2. K. Tomooka, C. Iso, K. Uehara, M. Suzuki, R. Nishikawa-Shimono, and K. Igawa, Angew. Chem. Int. Ed., 2012, 51, 10355. CrossRef
3. K. Igawa, K. Kawabata, R. Ni, and K. Tomooka, Chem. Lett., 2013, 42, 1374. CrossRef
4. (a) K. Tomooka, M. Suzuki, M. Shimada, S. Yanagitsuru, and K. Uehara, Org. Lett., 2006, 8, 963; CrossRef (b) K. Tomooka, M. Suzuki, K. Uehara, M. Shimada, and T. Akiyama, Synlett, 2008, 2518; CrossRef (c) K. Tomooka, T. Akiyama, P. Man, and M. Suzuki, Tetrahedron Lett., 2008, 49, 6327; CrossRef (d) K. Tomooka, K. Uehara, R. Nishikawa, M. Suzuki, and K. Igawa, J. Am. Chem. Soc., 2010, 132, 9232; CrossRef (e) K. Tomooka, M. Shimada, K. Uehara, and M. Ito, Organometallics, 2010, 29, 6632; CrossRef (f) K. Tomooka, M. Suzuki, M. Shimada, R. Ni, and K. Uehara, Org. Lett., 2011, 13, 4926. CrossRef
5. For general review for Mitsunobu reaction, see: O. Mitsunobu, Synthesis, 1981, 1. CrossRef
6. Construction of the nine-membered nitrogen heterocycle skeletons via C–N bonds formation using double Mitsunobu reaction have been reported by Fukuyama and colleagues in the total synthesis of alkaloids, see: (a) Y. Kaburagi, H. Tokuyama, and T. Fukuyama, J. Am. Chem. Soc., 2004, 126, 1024; CrossRef (b) N. Shimada, Y. Abe, S. Yokoshima, and T. Fukuyama, Angew. Chem. Int. Ed., 2012, 51, 11824. CrossRef
7. (a) S. Ohira, Synth. Commun., 1989, 19, 561; CrossRef (b) S. Müller, B. Liepold, G. J. Roth, and H. J. Bestmann, Synlett, 1996, 521; CrossRef (c) G. J. Roth, B. Liepold, S. Müller, and H. J. Bestmann, Synthesis, 2004, 59. CrossRef
8. 3a: a colorless crystal; IR (crystal using a diffuse reflector) cm–1: 2939, 2227, 1597, 1157, 1098, 657; 1H NMR (CDCl3, 300 MHz) δ: 7.69 (d, J = 8.1 Hz, 2H), 7.31 (d, J = 8.1 Hz, 2H), 5.82-5.73 (m, 1H), 5.62-5.53 (m, 1H), 3.90 (s, 2H), 3.81 (d, J = 8.1 Hz, 2H), 2.43 (s, 3H), 2.34-2.28 (m, 2H), 2.17-2.10 (m, 2H); 13C NMR (CDCl3, 75 MHz) δ: 143.6, 135.8, 131.7, 129.9, 128.0, 127.3, 94.6, 83.4, 44.2, 39.7, 27.0, 21.6, 19.1.
9. 3b: a colorless crystal; IR (crystal using a diffuse reflector) cm–1: 2923, 2234, 1597, 1447, 1348, 1160, 1092, 657; 1H NMR (CDCl3, 300 MHz) δ: 7.68 (d, J = 8.4 Hz, 2H), 7.31 (d, J = 8.4 Hz, 2H), 5.54 (dt, J = 1.2, 8.1 Hz, 1H), 4.20-3.40 (br, 4H), 2.43 (s, 3H), 2.40-2.15 (br, 4H), 1.75 (s, 3H); 13C NMR (CDCl3, 75 MHz) δ: 143.4, 135.5, 135.2, 129.8, 127.1, 125.9, 95.1, 83.4, 45.4, 39.7, 32.1, 24.5, 21.7, 18.2.
10. Deposition number CCDC-1020694 for compound 3b. Selected crystallographic data: triclinic, P–1 (No. 2), a = 6.8112(11) Å, b = 8.1570(13) Å, c = 13.487(3) Å, α = 79.760(11), β = 79.401(10), γ = 82.719(11), V = 721.4(2) Å3, Z = 2, R1 = 0.0682, wR2 = 0.1998. Free copies of the data can be obtained via http://www.ccdc.cam.ac.uk/conts/retrieving.html (or from the Cambridge Crystallographic Data Centre, 12 Union Road, Cambridge, CB2 1EZ, UK; Fax: +44 1223 336033; e-mail: deposit@ccdc.cam.ac.uk).
11. DFT calculations were performed at B3LYP/6-311G(2d,2p) level of theory with Gaussian 09 package. Frisch, M. J. et al. Gaussian 09, Revision C.01; Gaussian, Inc.: Wallingford CT, 2010.
12. The DFT calculations show that the transition state for the racemization of 2 and 3 involves the flipping of the benzene ring or Z-alkene moiety, respectively (TS-2 and TS-3). The energy barrier for racemization of 2 is higher than that of 3, due to the substantial 1,3-steric repulsion between the C6 and C9 methylene protons and the Ha or Hb protons of benzene ring. On the other hand, the flipping of alkene of 3a (R = H) can proceed without such steric repulsion. Racemization of 3b (R = Me) needs a slightly higher energy than that of 3a, to overcome the 1,2-steric repulsion between the C6 proton and the methyl group.