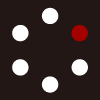
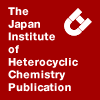
HETEROCYCLES
An International Journal for Reviews and Communications in Heterocyclic ChemistryWeb Edition ISSN: 1881-0942
Published online by The Japan Institute of Heterocyclic Chemistry
e-Journal
Full Text HTML
Received, 5th September, 2014, Accepted, 19th January, 2015, Published online, 26th January, 2015.
DOI: 10.3987/COM-14-13084
■ Design, Synthesis, and Biological Activity Evaluation of Thiazolidinones Containing Alkynyl and Alkenyl Furans for Disrupting Protein–Protein Interactions between HIF-1α and p300
Norihiko Takeda, Tomoyo Taguchi, Takeshi Nakajima,* and Mitsuyoshi Azuma
Senju Laboratory of Ocular Sciences, Senju Pharmaceutical Co., Ltd., 1-5-5, Minatojima-Minamimachi, Chuo-ku, Kobe, Hyogo, 650-0047, Japan
Abstract
Based on the structure of the potent hypoxia-inducible factor (HIF) inhibitor 1, a novel series of furanylidene-thiazolidinones 6 were designed and synthesized using Sonogashira or Suzuki–Miyaura cross-couplings, and subsequent Knoevenagel condensation. In particular, derivatives 6aA, 6cA, 6dA, 6hA, 6iA, and 6cC, bearing an alkynyl or alkenyl group on the furan ring, exhibited four- to five-fold higher activities than 1. These potent compounds will serve as leads for the development of novel small molecules for targeting the HIF-1α/p300 complex.Hypoxia-inducible factor 1 (HIF-1) is a transcriptional activator that functions as a master regulator of oxygen homeostasis.1 HIF-1 consists of an oxygen-sensitive α subunit and a constitutive β subunit.2 Under normoxic conditions, the α subunits on proline residues are hydroxylated by prolylhydroxylases, ubiquitylated in a von Hippel–Lindau protein-dependent manner, and undergo proteasomal degradation.3 In contrast, under hypoxic conditions, HIF-1α cannot be hydroxylated, because of the reduced molecular oxygen concentration, and translocates to the nucleus, where it dimerizes with the HIF-1 β subunit, and recruits coactivators p300/CBP, thereby inducing upregulating transcription of various hypoxia-responsive genes. These include various genes involved in the uptake and metabolism of glucose (GLUT1 and LDH), angiogenesis (VEGFA and PDGF), mitogenesis (TGFα), and erythropoiesis (EPO) in tumor biology.4 HIF-1 is therefore an attractive target for the suppression of hypoxia-mediated tumor biology, including angiogenesis, because of its central role in tumor biology. There are many reports on small-molecule inhibitors of HIF signaling. Early inhibitors for HIF signaling are indirect inhibitors such as heat shock protein 90 (HSP 90) and topoisomerase I inhibitors.5,6 Their lack of selectivities against HIF makes it difficult to correlate molecular and clinical responses.7 Kung’s group suggested that disruption of transcription factor HIF-1α interactions with transcriptional cofactors p300/CBP could potentially downregulate the expression of genes encoding VEGFA and its receptor VEGFR2, which are involved in the induction of new blood vessels (angiogenesis) in solid tumors.8 Small-molecule inhibitors targeting HIF-1α–p300 interaction have therefore attracted increasing levels of interest from medicinal and organic chemists over the past decade; for example, chetomin,9 KCN-1,10 KST012174,11 novobiocin,12 menadione,13 indole derivatives,14 and thiazolidinone compounds15 have been reported as inhibitors for blocking interactions between HIF-1α and p300.16,17
As a part of our program directed toward the development of new HIF inhibitors which have more potent activity, we describe the design, synthesis, and biological activity evaluation of novel alkynylfuran- and alkenylfuran-linked thiazolidinones as inhibitors for disrupting complex formation by HIF-1α–p300 interactions. The target molecules 2, containing alkynyl and alkenylfurans, were designed based on the structure of 115 (Figure 1). We considered the 5-furanylmethylene-2-thiazolidin-4-one motif to be a privileged structure of scaffold 1, and therefore modified the p-cyanophenyl group (region I) of 1 with various alkynyl and alkenyl groups. Additionally, the introduction of a hydrophilic group on the thiazolidinone nitrogen (region II) was also investigated. We report the synthesis, structure–activity relationships, and in vitro evaluation of a new series of 5-[(5-substituted 2-furanyl)methylene]-2-thioxazolidin-4-ones 2.
The synthesis of target compounds 6 is summarized in Scheme 1. A novel series of thiazolidinones 6aA–fA, containing 5-alkynylfurans, was synthesized by Sonogashira cross-coupling, followed by Knoevenagel condensation. Thiazolidinones 6hA and 6iA, bearing 5-alkenylfurans, were synthesized using Suzuki–Miyaura cross-coupling and subsequent Knoevenagel condensation. Ethyl-substituted 6jA was synthesized by condensation of commercially available 4j with 5A in the presence of a catalytic amount of piperidine. The Knoevenagel condensation of 5B-D led to 6cB, 6hB, 6cC, and 6cD, bearing a hydrophilic group. Finally, the synthesis of Boc-β-alanine-tethered 6gA was achieved through condensation of 6fA with Boc-β-alanine-OH, using EDCI and HOBt in the presence of Et3N.
In this study, we designed and synthesized a series of 5-[(5-substituted 2-furanyl)methylene]-2-thioxazolidin-4-ones 6. All these compounds were evaluated in a binding assay against the interactions between HIF-1α and p300 (Table 1). First, substitution of 6 in region I with various alkynyl, alkenyl, and alkyl groups was examined. Initial replacement of the aromatic ring of 1 with a trimethylsilylalkynyl group resulted in increased inhibitory activity of HIF-1α–p300, with an IC50 value of 0.41 µM compared with 2.0 µM of compound 1 (entries 1 and 15). In our modified assay, the IC50 value of 1 was similar to the previous report.15 Inhibition by the triethylsilylalkynyl analog 6bA was slightly decreased by introducing a bulky group in region I, whereas the tert-butyl derivative 6cA and cyclopropylalkynyl derivative 6dA were similarly potent HIF-1α inhibitors, with IC50 values of 0.48 and 0.59 µM, respectively (entries 2–4). In contrast, gem-dimethylhydroxypropynyl derivative 6eA, hydroxypropynyl derivative 6fA, and Boc-β-alanine-tethered derivative 6gA, which introduced hydroxyl groups and Boc-β-alanine units at the propargylic position, were inefficient in improving the inhibitory activity (entries 5–7). Interestingly, the replacement of alkynyl groups in region I by tert-butyl and cyclopropylalkenyl groups led to an improvement in the HIF-1α inhibitory activity, compared with that of 1. 5-[(5-Alkenyl-2-furanyl)methylene]-2-thioxazolidin-4-ones 6hA and 6iA, possessing tert-butyl and cyclopropylalkenyl groups, showed good inhibitory activities, with IC50 values of 0.36 and 0.59 µM, respectively (entries 8 and 9). The ethyl-substituted 6jA had significantly decreased activity (entry 10). We therefore concluded that the introduction of multiple bonds with π-orbitals in region I is favorable for blocking complex formation by interactions between HIF-1α and p300, and led to a four- to five-fold increase in inhibitory activity compared with 1. Given the improved potency of the alkynyl and alkenyl analogs, we decided to replace the benzyl group in region II with a hydrophilic group. We selected 2-pyridinylmethyl, (4-methylpiperazinyl)methyl, and N,N-dimethylaminomethyl as the hydrophilic group at R2, and investigated the effect of t-butylalkynyl and t-butylalkenyl substituents at R1. Derivatives 6cB, 6hB, and 6cC displayed good IC50 values of 0.68, 0.62, and 0.58 µM, respectively (entries 11-13). When the nitrogen-containing heterocyclic group at R2 was replaced by its acyclic group, the inhibitory activity of 6cD was slightly reduced (entry 14). We therefore disclosed that the introduction of multiple bonds in region I improved the inhibitory activity against HIF-1α–p300 interactions, and that substitution of a nitrogen-containing heterocyclic group in region II can be accomplished without loss of inhibitory activity.
In conclusion, we designed and synthesized a new series of alkynylfuran- and alkenylfuran-containing thiazolidinones as novel HIF inhibitors for disrupting complex formation by HIF-1α–p300 interactions. Several potent HIF inhibitors with alkynyl and alkenyl substituents at the 5-position of the furan ring were discovered. Most of these derivatives had good inhibitory activities for blocking interactions between HIF-1α and p300. Further detailed studies on these compounds are in progress.
EXPERIMENTAL
Melting points were obtained using a Yanaco micro melting point apparatus MP-500V and are uncorrected. 1H and 13C NMR spectra were recorded on a Bruker ADVANCE Ⅲ (400 MHz and 100 MHz, respectively). IR spectra were recorded using an FTIR apparatus, Shimazu IRPrestige-21. Mass spectra were obtained by the ESI method from Shimazu LCMS-IT-TOF. Flash column chromatography (FCC) was performed using SiliaFlash® F60 (230–400 mesh). Medium-pressure column chromatography (MCC) was performed using UNIVERSALTM column (silica gel 40 µm 60Å, size M 20 I.D. × 80 mm and size 2L 26 I.D. × 170 mm packed length). 5-[2-(Trimethylsilyl)ethynyl]-2-furancarboxaldehyde 4a,18 3-(phenylmethyl)-2-thioxo-4-thiazolidinone 5A,15 3-[(2-dimethylamino)ethyl]-2-thioxo-4-thiazolidinone 5D,19 and 4-(5-{[4-oxo-3-(2-phenylethyl)-2- thioxo-5-thiazolidinylidene]methyl}-2-furanyl)benzonitrile 115 were prepared according to the literature procedures.
General procedure for preparation of 5-alkynyl-2-furaldehydes 4b-f
To a mixture of 5-bromo-2-furaldehyde 3 (1.03 g, 5.9 mmol), PdCl2(PPh3)2 (84.2 mg, 0.12 mmol), PPh3 (62.9 mg, 0.24 mmol), and CuI (66.7 mg, 0.35 mmol) were added Et3N (5.9 mL), THF (3.0 mL), and an appropriate terminal alkyne (7.13 mmol) under an argon atmosphere. After being stirred at room temperature for 2–4 h, the reaction mixture was filtered through a pad of Celite. The filtrate was concentrated under reduced pressure. The residue was purified by FCC to give 5-alkynyl-2-furaldehydes 4b–f as shown in Scheme.
5-[2-(Triethylsilyl)ethynyl]-2-furancarboxaldehyde (4b)
Yield 82%; pale yellow oil; IR (CHCl3) 2155, 1685 cm−1; 1H NMR (400 MHz, CDCl3) δ: 9.61 (br s, 1H), 7.20 (d, J = 3.6 Hz, 1H), 6.71 (d, J = 3.6 Hz, 1H), 1.04 (br t, J = 7.6 Hz, 9H), 0.70 (br q, J = 7.6 Hz, 6H); 13C NMR (100 MHz, CDCl3) δ: 177.3, 152.3, 141.7, 120.9, 117.4, 101.8, 94.0, 7.4, 4.1; HRMS (ESI, m/z) calcd for C13H18O2SiNa 257.0968 [M+Na]+, found 257.0974.
5-(3,3-Dimethyl-1-butyn-1-yl)-2-furancarboxaldehyde (4c)
Yield 84%; white solid; mp 91–92 °C; IR (KBr) 2220, 1689 cm−1; 1H NMR (400 MHz, CDCl3) δ: 9.57 (s, 1H), 7.19 (d, J = 3.6 Hz, 1H), 6.59 (d, J = 3.6 Hz, 1H), 1.33 (s, 9H);13C NMR (100 MHz, CDCl3) δ: 177.0, 151.9, 142.7, 121.5, 115.8, 106.3, 69.1, 30.4, 28.3; HRMS (ESI, m/z) calcd for C11H12O2Na 199.0730 [M+Na]+, found 199.0724.
5-(2-Cyclopropylethynyl)-2-furancarboxaldehyde (4d)
Yield 84%; yellow solid; mp 56–57 °C; IR (KBr) 2220, 1674 cm−1; 1H NMR (400 MHz, CDCl3) δ: 9.56 (s, 1H), 7.18 (d, J = 3.6 Hz, 1H), 6.57 (br d, J = 3.6 Hz, 1H), 1.53–1.46 (m, 1H), 0.98–0.89 (m, 4H); 13C NMR (100 MHz, CDCl3) δ: 177.1, 152.0, 142.7, 121.5, 116.1, 102.0, 65.5, 9.2, 0.3; HRMS (ESI, m/z) calcd for C10H8O2Na 183.0417 [M+Na]+, found 183.0425.
5-(3-Hydroxy-3-methyl-1-butyn-1-yl)-2-furancarboxaldehyde (4e)
Yield 92%; brown solid; mp 76–77 °C; IR (KBr) 3396, 2219, 1674 cm−1; 1H NMR (400 MHz, CDCl3) δ: 9.61 (br s, 1H), 7.21 (d, J = 3.6 Hz, 1H), 6.68 (d, J = 3.6 Hz, 1H), 2.17 (br s, 1H), 1.63 (s, 6H); 13C NMR (100 MHz, CDCl3) δ: 177.4, 152.3, 141.5, 121.4, 117.0, 101.2, 71.6, 65.5, 31.0; HRMS (ESI, m/z) calcd for C10H10O3Na 201.0522 [M+Na]+, found 201.0520.
5-(3-Hydroxy-1-propyn-1-yl)-2-furancarboxaldehyde (4f)
Yield 99%; brown solid; mp 94–95 °C; IR (KBr) 3444, 2216, 1659 cm−1; 1H NMR (400 MHz, CDCl3) δ: 9.62 (s, 1H), 7.21 (d, J = 3.6 Hz, 1H), 6.72 (d, J = 3.6 Hz, 1H), 4.53 (d, J = 6.0 Hz, 2H), 1.79 (br t, J = 6.0 Hz, 1H); 13C NMR (100 MHz, CDCl3) δ: 177.4, 152.5, 141.2, 121.0, 117.4, 94.9, 74.9, 51.3; HRMS (ESI, m/z) calcd for C8H6O3Na 173.0209 [M+Na]+, found 173.0216.
General procedure for preparation of 5-alkenyl-2-furaldehydes 4h and 4i
To a mixture of 5-bromo-2-furaldehyde 3 (2.0 mmol), boronic acid pinacol ester (2.4 mmol), Pd(PPh3)4 (115.5 mg, 0.10 mmol), and K2CO3 (414.6 mg, 3.0 mmol) were added 1,4-dioxane (6 mL) and H2O (1.2 mL) under an argon atmosphere. After being heated at reflux for 3.5–5 h, the reaction mixture was filtered through a pad of Celite and extracted with AcOEt. The organic phase was dried over MgSO4 and concentrated under reduced pressure. The residue was purified by MCC to give 5-alkenyl-2-furaldehydes 4h and 4i as shown in Scheme.
5-[(1E)-3,3-Dimethyl-1-buten-1-yl]-2-furancarboxaldehyde (4h)
Yield 51%; pale yellow oil; IR (KBr) 1662 cm−1; 1H NMR (400 MHz, CDCl3) δ: 9.54 (s, 1H), 7.20 (d, J = 3.6 Hz, 1H), 6.63 (br d, J = 16.0 Hz, 1H), 6.36 (d, J = 3.6 Hz, 1H), 6.20 (d, J = 16.0 Hz, 1H), 1.19 (br s, 9H); 13C NMR (100 MHz, CDCl3) δ: 175.2, 157.7, 149.8, 146.0, 122.4, 111.5, 107.3, 32.2, 27.5; HRMS (ESI, m/z) calcd for C11H14O2Na 201.0886 [M+Na]+, found 201.0884.
5-[(1E)-2-Cyclopropylethenyl]-2-furancarboxaldehyde (4i)
Yield 82%; pale yellow oil; IR (KBr) 1669 cm−1; 1H NMR (400 MHz, CDCl3) δ: 9.50 (s, 1H), 7.19 (d, J = 3.6 Hz, 1H), 6.34 (d, J = 16.0 Hz, 1H), 6.28 (d, J = 3.6 Hz, 1H), 6.08 (dd, J = 16.0, 9.6 Hz, 1H), 1.62–1.54 (m, 1H), 0.94–0.89 (m, 2H), 0.63–0.59 (m, 2H); 13C NMR (100 MHz, CDCl3) δ: 176.5, 158.9, 151.1, 141.7, 124.4, 114.9, 108.0, 15.0, 8.2; HRMS (ESI, m/z) calcd for C10H10O2Na 185.0573 [M+Na]+, found 185.0577.
3-[2-(2-Pyridinyl)ethyl]-2-thioxo-4-thiazolidinone (5B)
A suspension of bis(carboxymethyl)trithiocarbonate (5.0 g, 22 mmol), K2CO3 (1.4 g, 10 mmol), and 2-(2-aminoethyl)pyridine (2.4 mL, 20 mmol) in water was stirred at reflux for 4 h. After addition of AcOEt, the mixture was cooled to room temperature and extracted with AcOEt. The combined organic layers were dried over Na2SO4, and concentrated under reduced pressure. The residue was purified by FCC (n-hexane /AcOEt = 3/1→1/1) to give 5B (2.4 g, 54%) as a pale yellow solid. mp 100–101 °C; IR (KBr) 1714, 1677 cm−1; 1H NMR (400 MHz, CDCl3) δ: 8.54 (br d, J = 4.6 Hz, 1H), 7.62 (td, J = 7.8, 2.0 Hz, 1H), 7.20 (d, J = 7.8 Hz, 1H), 7.16 (br dd, J = 7.8, 4.6 Hz, 1H), 4.39 (t, J = 7.6 Hz, 2H), 3.95 (s, 2H), 3.14 (t, J = 7.6 Hz, 2H); 13C NMR (100 MHz, CDCl3) δ: 200.9, 173.6, 157.6, 149.2, 136.9, 123.6, 122.0, 44.2, 35.3, 34.6; HRMS (ESI, m/z) calcd for C10H11N2OS2 239.0307 [M+H]+, found 239.0306.
3-[2-(4-Methyl-1-piperazinyl)ethyl]-2-thioxo-4-thiazolidinone (5C)
A solution of bis(carboxymethyl)trithiocarbonate (630 mg, 2.8 mmol), Et3N (0.97 mL, 7 mmol) and 1-(2-aminoethyl)-4-methylpiperazine (463 mg, 2.6 mmol) in DME (25 mL) was stirred at reflux for 9 h. The mixture was diluted with water, and extracted with CH2Cl2/MeOH = 5/1. The combined organic layers were dried over Na2SO4, and concentrated under reduced pressure. The residue was purified by FCC (CH2Cl2/MeOH = 10/1→5/1) to give 5C (175.0 mg, 26%) as a purple solid. mp 85–86 °C; IR (KBr) 1740 cm−1; 1H NMR (400 MHz, CDCl3) δ: 4.13 (t, J = 6.8 Hz, 2H), 3.98 (s, 2H), 2.64 (t, J = 6.8 Hz, 2H), 2.59 (br m, 4H), 2.47 (br m, 4H), 2.31 (s, 3H); 13C NMR (100 MHz, CDCl3) δ: 201.2, 173.8, 55.0, 53.9, 52.9, 45.8, 41.7, 35.3; HRMS (ESI, m/z) calcd for C10H18N3OS2 260.0886 [M+H]+, found 260.0881.
General procedure for preparation of 5-[(5-substituted 2-furannyl)methylene]-2- thioxazolidin-4-ones 6
To a mixture of 5-substituted 2-furaldehyde 4 (1.0 mmol) and N-substituted 2-thioxazolidin-4-one 5 (1.0 mmol) in EtOH (8 mL) was added piperidine (0.030 mL, 0.30 mmol). After being stirred at room temperature for 24 h, the solvent was removed by an evaporator. The residue was purified by MCC to afford 5-[(5-substituted 2-furannyl)methylene]-2-thioxazolidin-4-ones 6 as shown in Scheme.
3-Phenylmethyl-5-{[5-(2-trimethylsilyl)ethynyl-2-furanyl]methylene}-2-thioxo-4-thiazolidinone (6aA)
Yield 41%; orange solid; mp 133–134 °C; IR (KBr) 2147, 1699, 1606 cm−1; 1H NMR (400 MHz, CDCl3) δ: 7.46–7.44 (m, 2H), 7.37 (s, 1H), 7.31–7.25 (m, 3H), 6.77 (d, J = 3.6 Hz, 1H), 6.71 (d, J = 3.6 Hz, 1H), 5.30 (s, 2H), 0.29 (s, 9H); 13C NMR (100 MHz, CDCl3) δ: 194.6, 167.9, 150.6, 141.4, 135.3, 129.3, 129.0, 128.5, 122.7, 119.7, 119.3, 117.6, 105.1, 93.7, 47.9, 0.4; HRMS (ESI, m/z) calcd for C20H19NO2S2SiNa 420.0519 [M+Na]+, found 420.0536.
3-Phenylmethyl-5-{[5-(2-triethylsilyl)ethynyl-2-furanyl]methylene}-2-thioxo-4-thiazolidinone (6bA)
Yield 97%; orange solid; mp 91–92 °C; IR (KBr) 2149, 1695, 1609 cm−1; 1H NMR (400 MHz, CDCl3) δ: 7.46–7.44 (m, 2H), 7.38 (s, 1H), 7.33–7.27 (m, 3H), 6.77 (d, J = 3.6 Hz, 1H), 6.71 (d, J = 3.6 Hz, 1H), 5.31 (s, 2H), 1.06 (t, J = 7.6 Hz, 9H), 0.72 (q, J = 7.6 Hz, 6H); 13C NMR (100 MHz, CDCl3) δ: 194.2, 167.5, 150.1, 141.1, 134.9, 128.9, 128.6, 128.1, 122.2, 119.3, 118.8, 117.3, 102.7, 94.5, 47.5, 7.4, 4.1; HRMS (ESI, m/z) calcd for C23H25NO2S2SiNa 462.0988 [M+Na]+, found 462.1007.
5-{[5-(3,3-Dimethyl-1-butyn-1-yl)-2-furanyl]methylene}-3-phenylmethyl-2-thioxo-4-thiazolidinone (6cA)
Yield 58%; orange solid; mp 149–150 °C; IR (KBr) 2219, 1694, 1607 cm−1; 1H NMR (400 MHz, CDCl3) δ: 7.46–7.44 (m, 2H), 7.37 (s, 1H), 7.33–7.27 (m, 3H), 6.77 (d, J = 3.6 Hz, 1H), 6.58 (d, J = 3.6 Hz, 1H), 5.31 (s, 2H), 1.35 (s, 9H); 13C NMR (100 MHz, CDCl3) δ: 194.3, 167.5, 149.5, 141.9, 135.0, 128.9, 128.5, 128.0, 121.1, 119.7, 117.5, 117.2, 107.1, 69.4, 47.4, 30.5, 28.4; HRMS (ESI, m/z) calcd for C21H19NO2S2Na 404.0749 [M+Na]+, found 404.0757.
5-{[5-(2-Cyclopropylethynyl)-2-furanyl]methylene}-3-phenylmethyl-2-thioxo-4-thiazolidinone (6dA)
Yield 87%; orange solid; mp 163–164 °C; IR (KBr) 2209, 1697, 1603 cm−1; 1H NMR (400 MHz, CDCl3) δ: 7.46–7.44 (m, 2H), 7.35 (s, 1H), 7.33–7.27 (m, 3H), 6.76 (d, J = 3.6 Hz, 1H), 6.57 (d, J = 3.6 Hz, 1H), 5.30 (s, 2H), 1.56–1.50 (m, 1H), 1.00–0.90 (m, 4H); 13C NMR (100 MHz, CDCl3) δ: 194.5, 167.7, 149.7, 142.1, 135.1, 129.0, 128.7, 128.2, 121.5, 119.9, 117.6, 117.5, 102.9, 66.0, 47.6, 9.3, 0.6; HRMS (ESI, m/z) calcd for C20H15NO2S2Na 388.0436 [M+Na]+, found 388.0448.
5-{[5-(3-Hydroxy-3-methyl-1-butyn-1-yl)-2-furanyl]methylene}-3-phenylmethyl-2-thioxo-4-thiazolidinone (6eA)
Yield 92%; brown solid; mp 145–146 °C; IR (KBr) 3359, 2218, 1699, 1608 cm−1; 1H NMR (400 MHz, CDCl3) δ: 7.46–7.44 (m, 2H), 7.37 (s, 1H), 7.35–7.27 (m, 3H), 6.77 (d, J = 3.6 Hz, 1H), 6.67 (d, J = 3.6 Hz, 1H), 5.31 (s, 2H), 2.04 (br s, 1H), 1.65 (s, 6H); 13C NMR (100 MHz, CDCl3) δ: 194.2, 167.5, 150.2, 140.7, 134.9, 128.9, 128.6, 128.1, 122.1, 119.3, 118.4, 117.3, 101.8, 72.1, 65.7, 47.5, 31.1; HRMS (ESI, m/z) calcd for C20H17NO3S2Na 406.0542 [M+Na]+, found 406.0551.
5-{[5-(3-Hydroxy-1-propyn-1-yl)-2-furanyl]methylene}-3-phenylmethyl-2-thioxo-4-thiazolidinone (6fA)
Yield 44%; brown solid; mp 174–175 °C (dec.); IR (KBr) 3312, 1698, 1603 cm−1; 1H NMR (400 MHz, CDCl3) δ: 7.46–7.44 (m, 2H), 7.38 (s, 1H), 7.34–7.27 (m, 3H), 6.78 (d, J = 3.6 Hz, 1H), 6.71 (d, J = 3.6 Hz, 1H), 5.30 (s, 2H), 4.56 (d, J = 6.0 Hz, 2H), 1.76 (br t, J = 6.0 Hz, 1H); 13C NMR (100 MHz, CDCl3) δ: 194.1, 167.5, 150.5, 140.3, 134.9, 128.9, 128.6, 128.1, 122.6, 119.1, 118.8, 117.1, 95.6, 75.5, 51.6, 47.5; HRMS (ESI, m/z) calcd for C18H13NO3S2Na 378.0229 [M+Na]+, found 378.0241.
5-({5-[(1E)-3,3-Dimethyl-1-buten-1-yl]-2-furanyl}methylene)-3-phenylmethyl-2-thioxo-4-thiazolidinone (6hA)
Yield 78%; orange solid; mp 160–161 °C; IR (KBr) 1696, 1601 cm−1; 1H NMR (400 MHz, CDCl3) δ: 7.47–7.44 (m, 2H), 7.42 (s, 1H), 7.33–7.27 (m, 3H), 6.84 (d, J = 3.6 Hz, 1H), 6.49 (d, J = 16.0 Hz, 1H), 6.36 (d, J = 3.6 Hz, 1H), 6.20 (d, J = 16.0 Hz, 1H), 5.33 (s, 2H), 1.16 (s, 9H); 13C NMR (100 MHz, CDCl3) δ: 194.4, 167.6, 158.8, 148.8, 146.6, 135.1, 128.8, 128.5, 128.0, 121.5, 119.0, 118.1, 113.1, 110.6, 47.5, 33.9, 29.3; HRMS (ESI, m/z) calcd for C21H21NO2S2Na 406.0906 [M+Na]+, found 406.0926.
5-({5-[(1E)-2-Cyclopropylethenyl]-2-furanyl}methylene)-3-phenylmethyl-2-thioxo-4-thiazolidinone (6iA)
Yield 81%; orange solid; mp 150–151 °C; IR (KBr) 1702, 1594 cm−1; 1H NMR (400 MHz, CDCl3) δ: 7.46–7.45 (m, 2H), 7.39 (s, 1H), 7.33–7.25 (m, 3H), 6.83 (d, J = 3.6 Hz, 1H), 6.32 (d, J = 16 Hz, 1H), 6.28 (d, J = 3.6 Hz, 1H), 6.02 (dd, J = 16, 9.2 Hz, 1H), 5.32 (s, 2H), 1.62–1.56 (m, 1H), 0.97–0.92 (m, 2H), 0.69–0.65 (m, 2H); 13C NMR (100 MHz, CDCl3) δ: 194.4, 167.6, 158.5, 148.7, 140.4, 135.1, 128.8, 128.5, 128.0, 121.8, 118.7, 118.1, 115.0, 110.0, 47.5, 15.2, 8.6; HRMS (ESI, m/z) calcd for C20H17NO2S2Na 390.0593 [M+Na]+, found 390.0609.
5-[(5-Ethyl-2-furanyl)methylene]-3-phenylmethyl-2-thioxo-4-thiazolidinone (6jA)
Yield 72%; brown powder; mp 140–141 °C; IR (KBr) 1700, 1610 cm−1; 1H NMR (400 MHz, CDCl3) δ: 7.46–7.44 (m, 2H), 7.41 (s, 1H), 7.33–7.27 (m, 3H), 6.77 (d, J = 3.6 Hz, 1H), 6.21 (d, J = 3.6 Hz, 1H), 5.31 (s, 2H), 2.75 (q, J = 7.6 Hz, 2H), 1.31 (t, J = 7.6 Hz, 3H); 13C NMR (100 MHz, CDCl3) δ: 194.7, 167.6, 164.1, 148.7, 135.1, 128.9, 128.5, 128.0, 120.6, 118.9, 118.7, 108.9, 47.4, 22.0, 11.5; HRMS (ESI, m/z) calcd for C17H15NO2S2Na 352.0436 [M+Na]+, found 352.0449.
5-{[5-(3,3-Dimethyl-1-butyn-1-yl)-2-furanyl]methylene}-3-[2-(2-pyridinyl)ethyl]-2-thioxo-4-thiazoli-dinone (6cB)
Yield 71%; yellow solid; mp 163–164 °C; IR (KBr) 2213, 1701, 1608 cm−1; 1H NMR (400 MHz, CDCl3) δ: 8.55 (br d, J = 4.8 Hz, 1H), 7.58 (br td, J = 7.6, 1.6 Hz, 1H), 7.33 (s, 1H), 7.17 (br d, J = 7.6 Hz, 1H), 7.12 (br dd, J = 7.6, 4.8 Hz, 1H), 6.76 (d, J = 3.6 Hz, 1H), 6.58 (d, J = 3.6 Hz, 1H), 4.52 (br t, J = 7.6 Hz, 2H), 3.20 (br t, J = 7.6 Hz, 2H), 1.35 (s, 9H); 13C NMR (100 MHz, CDCl3) δ: 194.4, 167.3, 157.9, 149.6, 141.9, 136.4, 123.4, 121.7, 121.2, 119.6, 117.3, 117.2, 107.1, 69.4, 44.1, 35.1, 30.5, 28.4; HRMS (ESI, m/z) calcd for C21H21N2O2S2 397.1039 [M+H]+, found 397.1053.
5-({5-[(1E)-3,3-Dimethyl-1-buten-1-yl]-2-furanyl}methylene)-3-[2-(2-pyridinyl)ethyl]-2-thioxo-4-thiazolidinone (6hB)
Yield 83%; yellow solid; mp 157–158 °C; IR (KBr) 1700, 1597 cm−1; 1H NMR (400 MHz, CDCl3) δ: 8.56 (br d, J = 4.8 Hz, 1H), 7.59 (br td, J = 7.6, 1.6 Hz, 1H), 7.38 (s, 1H), 7.18 (br d, J = 7.6 Hz, 1H), 7.13 (br dd, J = 7.6, 4.8 Hz, 1H), 6.83 (d, J = 3.6 Hz, 1H), 6.50 (d, J = 16 Hz, 1H), 6.36 (d, J = 3.6 Hz, 1H), 6.20 (d, J = 16 Hz, 1H), 4.53 (br t, J = 7.6 Hz, 2H), 3.21 (br t, J = 7.6 Hz, 2H), 1.16 (s, 9H); 13C NMR (100 MHz, CDCl3) δ: 194.3, 167.3, 158.6, 157.9, 149.6, 148.8, 146.4, 136.4, 123.3, 121.7, 121.4, 119.0, 117.9, 113.1, 110.6, 44.0, 35.1, 33.9, 29.3; HRMS (ESI, m/z) calcd for C21H23N2O2S2 399.1195 [M+H]+, found 399.1196.
5-{[5-(3,3-Dimethyl-1-butyn-1-yl)-2-furanyl]methylene}-3-[2-(4-methyl-1-piperazinyl)ethyl]-2-thioxo-4-thiazolidinone (6cC)
Yield 25%; brown solid; mp 147–148 °C; IR (KBr) 2215, 1701, 1609 cm−1; 1H NMR (400 MHz, CDCl3) δ: 7.36 (s, 1H), 6.78 (d, J = 3.6 Hz, 1H), 6.59 (d, J = 3.6 Hz, 1H), 4.25 (br t, J = 7.6 Hz, 2H), 2.69 (br t, J = 7.6 Hz, 2H), 2.60 (br m, 4H), 2.45 (br m, 4H), 2.28 (s, 3H), 1.35 (s, 9H); 13C NMR (100 MHz, CDCl3) δ: 194.6, 167.5, 149.6, 141.9, 121.3, 119.6, 117.3, 117.2, 107.1, 69.4, 55.1, 54.1, 53.1, 45.9, 41.7, 30.5, 28.4; HRMS (ESI, m/z) calcd for C21H28N3O2S2 418.1617 [M+H]+, found 418.1608.
5-{[5-(3,3-Dimethyl-1-butyn-1-yl)-2-furanyl]methylene}-3-[(2-dimethylamino)ethyl]-2-thioxo-4-thiazolidinone (6cD)
Yield 33%; brown solid; mp 146–147 °C; IR (KBr) 2214, 1709, 1606 cm−1; 1H NMR (400 MHz, CDCl3) δ: 7.36 (s, 1H), 6.77 (d, J = 3.6 Hz, 1H), 6.58 (d, J = 3.6 Hz, 1H), 4.24 (br t, J = 7.6 Hz, 2H), 2.62 (br t, J = 7.6 Hz, 2H), 2.32 (s, 6H), 1.34 (s, 9H); 13C NMR (100 MHz, CDCl3) δ: 194.7, 167.5, 149.6, 141.9, 121.3, 119.6, 117.4, 117.2, 107.1, 69.4, 55.6, 45.7, 42.3, 30.5, 28.4; HRMS (ESI, m/z) calcd for C18H23N2O2S2 363.1195 [M+H]+, found 363.1189.
N-[(1,1-Dimethylethoxy)carbonyl]-β-alanine 3-[5-(4-oxo-3-phenylmethyl-2-thioxo-5-thiazolidinylidene)methy-2-furanyl]-2-propyn-1-yl ester (6gA)
To a solution of 6fA (355.4 mg, 1.0 mmol) and Boc-β-alanine-OH (170.3 mg, 0.90 mmol) in THF (3.5 mL) were added Et3N (0.38 mL, 2.75 mmol), HOBt (168.4 mg, 1.10 mmol), and EDCI (210.9 mg, 1.10 mmol) at room temperature. After being stirred at the same temperature for 19 h, the reaction mixture was diluted with AcOEt. The mixture was washed with 1N HCl, saturated NaHCO3, H2O, and saturated NaCl. The organic phase was dried over MgSO4, and concentrated in vacuo. The residue was purified by MCC (n-hexane/AcOEt = 1/1) to afford 6gA (483.2 mg, 92%) as a yellow solid. mp 124–125 °C; IR (KBr) 3344, 2233, 1733, 1701, 1680, 1607 cm−1; 1H NMR (400 MHz, CDCl3) δ: 7.46–7.44 (m, 2H), 7.38 (s, 1H), 7.34–7.27 (m, 3H), 6.78 (d, J = 3.6 Hz, 1H), 6.76 (d, J = 3.6 Hz, 1H), 5.30 (s, 2H), 4.98 (s, 2H), 3.44 (br q, J = 6.0 Hz, 2H), 2.62 (br t, J = 6.0 Hz, 2H), 1.43 (s, 9H); 13C NMR (100 MHz, CDCl3) δ: 194.0, 171.6, 167.5, 155.8, 150.8, 139.8, 134.9, 128.9, 128.6, 128.1, 122.9, 119.5, 118.9, 117.0, 91.2, 79.5, 76.3, 52.5, 47.5, 36.0, 34.5, 28.4; HRMS (ESI, m/z) calcd for C26H26N2O6S2Na 549.1124 [M+Na]+, found 549.1151.
In vitro assay for the inhibition of Biotin-HIF-1α786826/p300302-531
NUNC maxisorp 96-well plates (C96 Nunc Maxisorp plates) were incubated overnight with streptavidin (Sigma) to a final concentration of 1 µg/mL in PBS buffer. Each well was subsequently washed three times with 300 µL of TBST buffer (10 ×Tris-buffered saline, 0.05% Tween-20). A 10 nM solution (100 µL) of biotinylated HIF-1α786-826 (Biologica) in TBSB (TBS with 5%BSA) was then added to each well and incubated for 1 h at 25 °C. In the last row of each plate, only the TBSB buffer was added. Each well was subsequently washed three times with 300 µL of TBST buffer. To a 2 nM solution (98 µL) of p300 in the incubation buffer (TBSB added with 0.1% Tween-20, 0.5 mM 1,4-dithioerythritol (DTT), and 10 mM ZnCl2) were added 2 µL of the test compound dissolved in dimethyl sulfoxide (final concentrations: 0.1 to 50 µM), chetomin (final concentrations: 5 µM and 10 µM), and DMSO. The solutions were then mixed and added to each well. After incubation for 1 h at 25 °C, each well was washed three times with 300 µL of TBST buffer. Anti-6x Histidine (R&D) in 100 µL TBS with 5% BSA and 10 mM ZnCl2 was then added to each well. After incubation for 1 h at 25 °C, each well was washed three times with 300 µL of TBST buffer and once with 300 µL of TBS buffer, and then 100 µL of detection solution (1-step Ultra TMB ELIZA) was added. The optical density (OD630 nm) of the plate was measured using a Microplate reader (MTP-800) after 1 h. The inhibitory activity of the test compound was calculated from OD values. The data was shown as an average for three wells.
ACKNOWLEDGMENTS
The authors would like to thank Dr. Shinnosuke Machida for useful discussions.
References
1. G. L. Semenza, Cell, 2012, 148, 399. CrossRef
2. Y. Yang, M. Sun, L. Wang, and B. Jiao, J. Cell. Biochem., 2013, 114, 967. CrossRef
3. C. M. Tang and J. Yu, J. Gastroenterol. Hepatol., 2013, Mar, 28, 401. CrossRef
4. A. L. Jackson, B. Zhou B, and W. Y. Kim, Expert Opin. Ther. Targets, 2010, 14, 1047. CrossRef
5. Y. Wang, J. B. Trepel, L. M. Neckers, and G. Giaccone, Curr. Opin. Investig. Drugs, 2010, 11, 1466.
6. J. Y. Zeng, S. Sharma, Y. Q. Zhou, H. P. Yao, X. Hu, R. Zhang, and M. H. Wang, Mol. Cancer Ther., 2014, 13, 37. CrossRef
7. G. L. Semenza, Nat. Rev. Cancer, 2003, 3, 721. CrossRef
8. L. Kung, S. D. Zabludoff, D. S. France, S. J. Freedman, E. A. Tanner, A. Vieira, S. Cornell-Kennon, J. Lee, B. Wang, J. Wang, K. Memmert, H. U. Naegeli, F. Petersen, M. J. Eck, K. W. Bair, A. W. Wood, and D. M. Livingston, Cancer Cell, 2004, 6, 33. CrossRef
9. K. M. Cook, S. T. Hilton, J. Mecinović, W. B. Motherwell, W. D. Figg, and C. J. Schofield, J. Biol. Chem., 2009, 284, 26381. CrossRef
10. S. Yin, S. Kaluz, N. S. Devi, A. A. Jabbar, R. G. De Noronha, J. Mun, Z. Zhang, P. R. Boreddy, W. Wang, Z. Wang, T. Abbruscato, Z. Chen, J. J. Olson, R. Zhang, M. M. Goodman, K. C. Nicolaou, and E. G. Van Meir, Clin. Cancer Res., 2012, 18, 6623. CrossRef
11. K. M. Cook, S. T. Hilton, J. Mecinović, W. B. Motherwell, W. D. Figg, and C. J. Schofield, J. Biol. Chem., 2009, 284, 26381. CrossRef
12. D. Wu, R. Zhang, R. Zhao, G. Chen, Y. Cai, and J. Jin, PLOS one, 2013, 8, e62014. CrossRef
13. Y.-R. Na, K.-C. Han, H. Park, and E. G. Yang, Biochem. Biophys. Res. Commun., 2010, 296, 370.
14. M. Grugni, M. Cassin, G. Colella, S. De Munari, G. Pardi, and P. Pavesi, Cell Therapeutics Europe S. R. L., WO 2006/066923.
15. M. Cassin, G. Colella, S. De Munari, and M. Grugni, Cell Therapeutics Europe S. R. L., WO 2006/066846.
16. H. S. Ban, Y. Uto, and H. Nakamura, Expert Opin. Ther. Patents, 2011, 131. CrossRef
17. Y. Xia, H.-K. Choi, and K. Lee, Eur. J. Med. Chem., 2012, 49, 24 and references therein. CrossRef
18. A. S. K. Hashmi, E. Enns, T. M. Frost, S. Schäfer, W. Frey, and F. Rominger, Synthesis, 2008, 2707. CrossRef
19. S. Wang, Y. Zhao, G. Zhang, Y. Lv, N. Zhang, and P. Gong, Eur. J. Med. Chem., 2011, 46, 3509. CrossRef