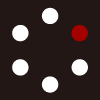
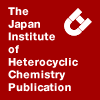
HETEROCYCLES
An International Journal for Reviews and Communications in Heterocyclic ChemistryWeb Edition ISSN: 1881-0942
Published online by The Japan Institute of Heterocyclic Chemistry
e-Journal
Full Text HTML
Received, 26th September, 2014, Accepted, 31st October, 2014, Published online, 4th November, 2014.
DOI: 10.3987/COM-14-13092
■ Rhodium(I)-N-Heterocyclic Carbene-Catalyzed Addition of Sodium Tetraphenylborate to Ketones to Form Tertiary Alcohols
Beyhan Yiğit, Murat Yiğit,* and İsmail Özdemir
Department of Chemistry, Faculty of Arts and Sciences, Adıyaman University, 02040 Adıyaman, Turkey
Abstract
Rhodium complexes ([Rh(COD)(NHC)Cl]) were synthesized by the reaction of bis(1,3-dialkylperhydrobenzimidazolin-2-ylidene) with [RhClCOD]2 in toluene and characterized by elemental analysis, 1H NMR, 13C NMR and IR spectroscopy. These complexes were used as catalysts for the addition of sodium tetraphenylborate to aromatic ketones and corresponding tertiary alcohols were obtained in good yields.INTRODUCTION
The additions of carbon nucleophiles to carbon heteroatom multiple bonds catalyzed by transition metal complexes are recognized to be an extremely important tool for carbon-carbon bond formation in organic synthesis and the addition products are important intermediates for the synthesis of biologically and pharmaceutically active compounds.1-7 The addition of aryl and alkenylboronic acids to aldehydes catalyzed by rhodium complexes were initially reported by Miyaura’s group in 19988 and in the following years, this transformation have attracted many chemists’ interest. There have been reports on the addition of arylboronic acids to aldehydes giving diarylmethanols or chiral diarylmethanols, which is catalyzed by Rh,9-14 Pd,15-21 Cu,22,23 Fe24 and Ni25-31 complexes. Among these complexes, rhodium and palladium catalysts are well works. In 2001, Fürstner and Krause32 reported the use of rhodium-carbene complexes formed in situ as catalysts in the arylation of aldehydes and, in subsequent years, rhodium-carbene complexes as catalysts were used by other groups.10, 33-43 Much progress have been seen in the addition of arylboron compounds to aldehydes. However, use of ketones as substrates are quite limited due to lesser reactivity of ketones toward the nucleophlic addition.12,44-47 Recently, arylboron compounds including arylboronic acids and tetraaryl borates have proved to be effective arylating reagents in rhodium catalysis.48-50 Rhodium-catalyzed arylation of ketones for tertiary alcohols by sodium tetraphenylborate as effective nucleofiles was achieved by Miura’s group in 2006.51 In the same year, Feringa et al.52 reported the first rhodium-catalyzed asymmetric 1,2-addition of arylboronic acids to trifluoroacetophenones for the synthesis of trifluoromethyl substituted tertiary alcohols with high yields and good enantioselectivities up to 83%. Recently, Buesking and Ellman have demonstrated the Rh-catalyzed addition of α-sulfonamido trifluoroborates to trifluoromethyl ketones for the asymmetric synthesis of vicinal amino alcohols with good diasteroselectivity.53
We have previously reported the rhodium-catalyzed addition of phenylboronic acid to aldehydes and sodium tetraphenylborate to C-N triple bond.54 Herein, we wish to report the synthesis of rhodium(I) complexes bearing perhydrobenzimidazolin-2-ylidene ligands and their use as catalyst in the addition of sodium tetraphenylborate to aromatic ketones.
RESULTS AND DISCUSSION
The tetraaminoethenes 1 used as the source of carbene were synthesized by deprotonation of the 1,3-dialkylperhydrobenzimidazolium salts with sodium hydride in dry and degassed tetrahydrofuran according to the procedures reported by Lappert.55 The reaction of bis(1,3-dialkylperhydrobenzimidazolin-2-ylidene) with the binuclear [RhCl(COD)]2 complex proceeded smoothly in refluxing toluene to give the RhCl(COD)(1,3-dialkylperhydrobenzimidazolin-2-ylidene) complexes as yellow-orange crystalline solids in 76-89% yields (Scheme 1). The rhodium complexes (2a-d) are very stable against to air and moisture in the solid state. The complexes (2a-d) have been fully identified by 1H and 13C- NMR spectroscopy, FTIR and elemental analysis. They show a characteristic υ(NCN) band at 1485, 1496, 1515, 1507 and 1511 cm-1 for 2a, 2b, 2c and 2d, respectively. The 13C- NMR chemical shifts, which provide a useful diagnostic tool for metal carbene complexes, show that Ccarbene is substantially deshielded. The resonance of the carbene carbon of rhodium complexes were observed as a doublet at 213.64 (2a), 219.52 (2b), 219.45 (2c), 219.55 (2d) ppm and coupling constants J(103Rh-13C) were 47.3 (2a, 2b, 2d), 48.0 (2c) Hz and these values were similar to those found for other rhodium(I) carbene complexes.39,56
The phenylation of ketones to tertiary alcohols were carried out using sodium tetraphenylborate as phenyl source with rhodium(I) carbene complexes as catalysts and ammonium chloride as promoter. Arylboronic acids can be used as aryl sources, but less effective than sodium tetraphenylborate. The use of sodium tetraphenylborate instead of arylboronic acids allowed elimination of the addition of base such as CsF in Suzuki-Miyaura type cross-coupling catalyzed by rhodium complex.57 Ammonium salts such as tetrapropylammonium bromide and tetrabutylammonium bromide as a promoter have long been known to improve the efficiency of Heck coupling.58 Although the role of NH4Cl is not clear for the phenylation of ketones, use of NH4Cl as an additive has been significantly raised the product yield from 47 up to 96%.51 The catalytic experiments were carried out using 0.5 mmol ketone, 0.25 mmol NaBPh4, 0.005 mmol 2a-d, 0.5 mmol NH4Cl and 5 mL o-xylene at 120 oC for 25 h. The conversion of the product was monitored by GC. The results of the catalytic phenylation of acetophenone, o-methoxyacetophenone, p-fluoroacetophenone, p-chloroacetophenone and p-methylacetophenone are summarized in Table 1.
The treatment of sodium tetraphenylborate with aromatic ketones bearing electron-withdrawing group such as Cl, F and unsubstituted (Table 1, entries 9-16, 1-4) gave corresponding tertiary alcohols in high yields under these conditions. Use of aromatic ketone bearing electron-donating group such as OMe and Me slightly decreased the yields under the same conditions (entries 5-8 and 17-20). The synthesized complexes were employed as catalyst for the addition of sodium tetraphenylborate to ketones. Among the tested complexes, the complex 2a bearing N,N’-(4-methylbenzyl)perhydrobenzimidazolylidene ligand is highly efficient in the addition of sodium tetraphenylborate to ketones (Table 1, entries 1, 5, 9, 13). This results demonstrated that rhodium(I) N-heterocyclic carbene complexes as catalyst were significantly increased the yields, but the reaction conditions were not improved compared to literature.51
CONCLUSION
In summary, four novel Rh-NHC complexes have been synthesized and characterized. All complexes are stable toward air and moisture in the both solid state and solution. We have demostrated the first use of these complexes as a catalyst in the addition of sodium tetraphenylborate to aromatic ketones to produce tertiary alcohols. The complexes showed high activity in these addition reactions. Among them, complex 2a is the most active complex. Further studies on chiral carbene complexes and asymmetric version of this process are in progress.
EXPERIMENTAL
All reactions for the preparation of 1-2 were carried out under an atmosphere of argon in flame-dried glassware by using standart Schlenk techniques. The solvents used were purified by distillation over the drying agents indicated and were transferred under an atmosphere of argon: THF, Et2O (Na/K alloy), CH2Cl2 (P4H10), hexane, toluene (Na). The complex [RhCl(COD)]2 59 was synthesized according to published procedure. 1H and 13C NMR spectra were recorded by using a Varian AS 400 Merkur spectrometer operating at 400 MHz (1H), 100 MHz (13C) in CDCl3 with tetramethylsilane as an internal reference. Chemical shifts (δ) are given in ppm relative to TMS, coupling constants (J) in hertz. FTIR spectra were recorded as KBr pellets in the range 400-4000 cm-1 on a Mattson 1000 spectrophotometer (wavenumbers, cm-1). GC spectra were recorded on an Agilent 6890 N GC system by GC-FID with an HP-5 column of 30 m length, 0.32 mm diameter and 0.25 µm film thickness. Melting points were measured in open capillary tubes with an electrothermal-9200 melting point apparatus and uncorrected. Elemental analyses were performed at Inönü University research center.
General procedure for the preparation of tetraaminoalkenes (1).
A suspension of the imidazolinium salt (10 mmol) and sodium hydride (15 mmol) in THF (20 mL) was stirred at room temperature for 12 h under an argon atmosphere, then heated under reflux for 4 h. The mixture was cooled and volatiles were removed in vacuo. Toluene (20 mL) was added and filtered. Filtrate was concentrated to half of the initial volume and hexane (10 mL) was added. Upon cooling, white crystals were obtained. The tetraaminoalkenes were not characterized due to their sensitivity towards oxygen and moisture.
General procedure for the preparation of the rhodium-carbene complexes (2a-d).
A solution of bis(1,3-dialkylperhydrobenzimidazolin-2-ylidene) (1) (0.40 mmol) and [RhCICOD]2 (0.40 mmol) in toluene (15 mL) was heated under reflux for 2 h. Upon cooling to room temperature, yellow-orange crystals of 2a-d were obtained. The crystals were filtered, washed with Et2O (3x15 mL) and dried under vacuum. The crude product was recrystallized from CH2Cl2/Et2O.
Chloro(η4-1,5-cyclooctadiene){1,3-bis(4-methylbenzyl)perhydrobenzimidazolin-2-ylidene}rhodium(I) (2a).
Yield: 0.20 g, 87%, mp 206 oC. IR(cm-1) ν: 1485 (NCN), 1H NMR (CDCl3) δ: 1.02-1.23 (m, 4H, -N-CHCH2CH2CH2CH2CH-N-); 1.43-1.65 (m, 4H, -N-CHCH2CH2CH2CH2CH-N-); 2.37-2.46 (m, 2H, -N-CHCH2CH2CH2CH2CH-N-); 7.17 (d, 4H, J = 8.0 Hz, CHarom); 7.47 (d, 4H, J = 8.0 Hz, CHarom); 4.46 (d, 2H, J = 14.4 Hz, -N-CH2-Ar); 6.33 (d, 2H, J = 14.4 Hz, -N-CH2-Ar); 2.3 (s, 6H, Ar-CH3-p); 3.37 (m, 2H, COD-CH); 5.02 (m, 2H, COD-CH); 1.90 (m, 4H, COD-CH2); 2.29 (m, 4H, COD-CH2). 13C NMR (CDCl3) δ: 213.64 (d, JRh-Carbene = 47.3 Hz, Ccarbene); 21.35 (-N-CHCH2CH2CH2CH2CH-N-); 21.38 (-N-CHCH2CH2CH2CH2CH-N-); 23.48 (-N-CHCH2CH2CH2CH2CH-N-); 24.00 (-N-CHCH2CH2CH2CH2CH-N-); 52.15 (-N-CHCH2CH2CH2CH2CH-N-); 52.94 (-N-CHCH2CH2CH2CH2CH-N-); 127.58 (Ar-C); 128.97 (Ar-C); 129.49 (Ar-C); 129.63 (Ar-C); 133.16 (Ar-C); 134.45 (Ar-C); 137.33 (Ar-C); 137.49 (Ar-C); 68.08 (-N-CH2-Ar); 68.22 (-N-CH2-Ar); 18.80 (Ar-CH3-p); 19.53 (Ar-CH3-p); 68.81 (d, JRh-C = 14.4 Hz, COD-CH); 99.71 (d, JRh-C = 6.1 Hz, COD-CH); 28.80 (COD-CH2); 28.94 (COD-CH2); 33.00 (COD-CH2); 33.08 (COD-CH2). Anal. Calcd for C31H40N2ClRh: C, 64.30; H, 6.96; N, 4.84. Found: C, 64.46; H, 6.72; N, 4.58.
Chloro(η4-1,5-cyclooctadiene){1,3-bis(2,3,4-trimethoxybenzyl)perhydrobenzimidazolin-2-ylidene}rhodium(I) (2b).
Yield 0.24 g, 83%, mp 192 oC. IR(cm-1) ν: 1496 (NCN), 1H NMR (CDCl3) δ: 0.92-1.18 (m, 4H, NCHCH2CH2CH2CH2CHN); 1.46-1.68 (m, 4H, NCHCH2CH2CH2CH2CHN); 2.82-2.89 (m, 2H, NCHCH2CH2CH2CH2CHN); 6.69 (d, 1H, J = 8.8 Hz, CHarom); 6.72 (d, 1H, J = 8.8 Hz, CHarom); 7.33 (d, 1H, J = 8.8 Hz, CHarom); 7.48 (d, 1H, J = 8.8 Hz, CHarom); 4.92 (d, 1H, J = 15.2 Hz, -N-CH2-Ar); 5.75 (d, 1H, J = 15.2 Hz, -N-CH2-Ar); 5.73 (d, 1H, J = 15.6 Hz, -N-CH2-Ar); 6.08 (d, 1H, J = 15.6 Hz, -N-CH2-Ar); 3.83 (s, 3H, -OCH3); 3.85 (s, 3H, -OCH3); 3.87 (s, 3H, -OCH3); 3.88 (s, 3H, -OCH3); 3.94 (s, 3H, -OCH3); 3.95 (s, 3H, -OCH3); 3.43 (m, 2H, COD-CH); 5.01 (m, 2H, COD-CH); 1.88 (m, 4H, COD-CH2);2.28 (m, 4H, COD-CH2). 13C NMR (CDCl3) δ: 219.52 (d, JRh-Carbene = 47.3 Hz, Ccarbene); 24.14 (-N-CHCH2CH2CH2CH2CH-N-); 24.40 (-N-CHCH2CH2CH2CH2CH-N-); 28.23 (-N-CHCH2CH2CH2CH2CH-N-); 28.61 (-N-CHCH2CH2CH2CH2CH-N-); 46.79 (-N-CHCH2CH2CH2CH2CH-N-); 47.33 (-N-CHCH2CH2CH2CH2CH-N-);107.65 (Ar-C); 107.94 (Ar-C); 122.93 (Ar-C); 123.94 (Ar-C); 124.52 (Ar-C); 128.88 (Ar-C); 141.85 (Ar-C); 142.18 (Ar-C); 151.05 (Ar-C); 151.74 (Ar-C); 153.01 (Ar-C); 153.11 (Ar-C); 67.93 (-N-CH2-Ar); 68.44 (-N-CH2-Ar); 56.14 (-OCH3); 61.02 (-OCH3); 61.08 (-OCH3); 61.35 (-OCH3); 69.01 (d, JRh-C = 14.5 Hz, COD-CH); 99.74 (d, JRh-C = 6.1 Hz, COD-CH); 28.99 (COD-CH2); 29.14 (COD-CH2); 32.69 (COD-CH2); 33.30 (COD-CH2). Anal. Calcd for C35H48N2ClO6Rh: C, 57.50; H, 6.62; N, 3.83. Found C, 57.64; H, 6.78; N, 3.95.
Chloro(η4-1,5-cyclooctadiene){1,3-bis(4-ethoxybenzyl)perhydrobenzimidazolin-2-ylidene}rhodium(I) (2c).
Yield 0.19 g, 76%, mp 218 oC. IR(cm-1) ν: 1515 (NCN), 1H NMR (CDCl3) δ: 0.81-0.97 (m, 4H, NCHCH2CH2CH2CH2CHN); 1.38-1.54 (m, 4H, NCHCH2CH2CH2CH2CHN); 2.74-2.82 (m, 2H, NCHCH2CH2CH2CH2CHN); 6.80 (d, 4H, J = 8.4 Hz, CHarom); 7.34 (d, 4H, J = 8.4 Hz, CHarom); 4.70 (d, 1H, J = 15.2 Hz, -N-CH2-Ar); 5.69 (d, 1H, J = 15.2 Hz, -N-CH2-Ar); 5.04 (d, 1H, J = 14.8 Hz, -N-CH2-Ar); 6.05 (d, 1H, J = 14.8 Hz, -N-CH2-Ar); 1.33 (t, 6H, J = 7.2 Hz, -OCH2CH3-p); 3.96 (quar, 4H, J = 5.2 Hz, -OCH2CH3-p); 3.34 (m, 2H, COD-CH); 4.94 (m, 2H, COD-CH); 1.81 (m, 4H, COD-CH2); 2.20(m, 4H, COD-CH2). 13C NMR (CDCl3) δ: 219.45 (d, JRh-C = 48.0 Hz, Ccarbene); 24.13 (-N-CHCH2CH2CH2CH2CH-N-); 24.38 (-N-CHCH2CH2CH2CH2CH-N-); 28.78 (-N-CHCH2CH2CH2CH2CH-N-); 28.84 (-N-CHCH2CH2CH2CH2CH-N-); 53.63 (-N-CHCH2CH2CH2CH2CH-N-); 53.95 (-N-CHCH2CH2CH2CH2CH-N-); 114.62 (Ar-C); 114.69 (Ar-C); 129.22 (Ar-C); 129.45 (Ar-C); 129.49 (Ar-C); 130.05 (Ar-C); 158.53 (Ar-C); 68.15 (-N-CH2-Ar); 68.40 (-N-CH2-Ar); 15.09 (OCH2CH3-p); 63.64 (OCH2CH3-p); 69.20 (d, J = 14.5 Hz, COD-CH); 99.83 (d, J = 6.1 Hz, COD-CH); 29.45 (COD-CH2); 29.48 (COD-CH2); 32.86 (COD-CH2); 33.10 (COD-CH2). Anal. Calcd for C33H44N2ClO2Rh: C, 62.02; H, 6.94; N, 4.38. Found: C, 62.21; H, 6.78; N, 4.54.
Chloro(η4-1,5-cyclooctadiene){1,3-bis(4-benzyloxybenzyl)perhydrobenzimidazolin-2-ylidene}rhodium(I) (2d)
Yield 0.27 g, 89%, mp 176 oC. IR(cm-1) ν: 1511 (NCN), 1H NMR (CDCl3) δ: 0.85-1.02 (m, 4H, NCHCH2CH2CH2CH2CHN); 1.46-1.63 (m, 4H, NCHCH2CH2CH2CH2CHN); 2.84-2.92 (m, 2H, NCHCH2CH2CH2CH2CHN); 6.95 (m, 9H, CHarom); 7.38 (m, 9H, CHarom); 4.70 (d, 1H, J = 15.2 Hz, -N-CH2-Ar); 5.74 (d, 1H, J = 15.2 Hz, -N-CH2-Ar); 5.15 (d, 1H, J = 15.2 Hz, -N-CH2-Ar); 6.15 (d, 1H, J = 15.2 Hz, -N-CH2-Ar); 5.05 (s, 2H, -O-CH2-Ar); 5.06 (s, 2H, -O-CH2-Ar); 3.41 (m, 2H, COD-CH); 5.02 (m, 2H, COD-CH); 1.88 (m, 4H, COD-CH2); 2.27 (m, 4H, COD-CH2). 13C NMR (CDCl3) δ: 219.55 (d, JRh-C = 47.3 Hz, Ccarbene); 24.12 (-N-CHCH2CH2CH2CH2CH-N-); 24.37 (-N-CHCH2CH2CH2CH2CH-N-); 28.74 (-N-CHCH2CH2CH2CH2CH-N-); 28.86 (-N-CHCH2CH2CH2CH2CH-N-); 53.62 (-N-CHCH2CH2CH2CH2CH-N-); 53.88 (-N-CHCH2CH2CH2CH2CH-N-); 115.05(Ar-C); 127.73 (Ar-C); 128.18 (Ar-C); 128.79 (Ar-C); 129.28 (Ar-C); 129.53 (Ar-C); 129.86 (Ar-C); 130.57 (Ar-C); 137.27 (Ar-C); 158.48 (Ar-C); 67.89 (-N-CH2-Ar); 68.21 (-N-CH2-Ar); 68.34 (-O-CH2-Ar); 70.30 (-O-CH2-Ar); 69.25 (d, JRh-C = 14.5 Hz, COD-CH); 99.90 (d, JRh-C = 6.9 Hz, COD-CH); 29.41 (COD-CH2); 29.47 (COD-CH2); 32.85 (COD-CH2); 33.10 (COD-CH2). Anal. Calcd for C43H48N2ClO2Rh: C, 67.67; H, 6.34; N, 3.76. Found: C, 67.82; H, 6.52; N, 3.86.
General procedure for rhodium-carbene catalyzed reaction of sodium tetraphenylborate with acetophenone
In a schlenk tube was placed NH4Cl (27 mg, 0.5 mmol), which was then dried 150 oC in vacuo for 2 h. Then sodium tetraphenylborate (85.5 mg, 0.25 mmol), substituted ketones (0.5 mmol), rhodium carbene catalyst (0.005 mmol), o-xylene (5 mL) were added and the resulting mixture was heated for 25 h at 120 oC, cooled to ambient temperature, exracted with EtOAc (30 mL). After drying over MgSO4 the organic phase was evaporated and the residue was purified by flash chromatography (hexane/EtOAc, 5/1). Isolated yield is checked by NMR and GC, all reactions were monitored by TLC.
ACKNOWLEDGEMENTS
This research was supported by the Adıyaman University Research Fund (FEFBAP/2012-0006).
References
1. M. Beller and C. Bolm, Transition Metals for Organic Synthesis: Building Blocks and Fine Chemicals, Wiley-VCH, New York, 1998. CrossRef
2. K. Fagnou and M. Lautens, Chem. Rev., 2003, 103, 169. CrossRef
3. T. Hayashi and K. Yamasaki, Chem. Rev., 2003, 103, 2829. CrossRef
4. F. Schmidt, R. T. Stemmler, J. Rudolph, and C. Bolm, Chem. Soc. Rev., 2006, 35, 454.
5. A. F. Casy, A. F. Drake, C. R. Ganellin, A. D. Mercer, and C. Upton, Chirality, 1992, 4, 356. CrossRef
6. S. Stanev, R. Rakovska, N. Berova, and G. Snatzke, Tetrahedron: Asymmetry, 1995, 6, 183. CrossRef
7. A. F. Trindade, P. M. P. Gois, L. F. Veiros, V. Andre, M. T. Duarte, C. A. M. Afonso, S. Caddick, and F. G. N. Cloke, J. Org. Chem., 2008, 73, 4076. CrossRef
8. M. Sakai, M. Ueda, and N. Miyaura, Angew. Chem. Int. Ed., 1998, 37, 3279. CrossRef
9. R. Huang and K. H. Shaughnessy, Chem. Commun., 2005, 4484. CrossRef
10. P. M. P. Gois, A. F. Trindade, L. F. Veiros, V. Andre, M. T. Duarte, C. A. M. Afonso, S. Caddick, and F. G. N. Cloke, Angew. Chem. Int. Ed., 2007, 46, 5750. CrossRef
11. C.-H. Xing, T.-P. Liu, J. R. Zeng, J. Ng, M. Eposite, and Q.-S. Hu, Tetrahedron Lett., 2009, 50, 4953. CrossRef
12. J. R. White, G. J. Price, P. K. Plucinski, and C. G. Frost, Tetrahedron Lett., 2009, 50, 7365. CrossRef
13. T. Nishimura, H. Kumamoto, M. Nagaosa, and T. Hayashi, Chem. Commun., 2009, 5713. CrossRef
14. Q. Ma, Y. Ma, X. Liu, W. Duan, B. Qu, and C. Song, Tetrahedron: Asymmetry, 2010, 21, 292. CrossRef
15. K. Suzuki, T. Arao, S. Ishii, Y. Maeda, K. Kondo, and T. Aoyama, Tetrahedron Lett., 2006, 47, 5789. CrossRef
16. A. Yu, B. Cheng, Y. Wu, J. Li, and K. Wei, Tetrahedron Lett., 2008, 49, 5405. CrossRef
17. T. Yamamoto, M. Lizuka, H. Takenaka, T. Ohta, and Y. Ito, J. Organomet. Chem., 2009, 694, 1325. CrossRef
18. P. He, Y. Lu, C.-G. Dong, and Q.-S. Hu, Org. Lett., 2007, 9, 343. CrossRef
19. T. Yamamoto, T. Ohta, and Y. Ito, Org. Lett., 2005, 7, 4153. CrossRef
20. S. Lin and X. Lu, Org. Lett., 2007, 72, 9757.
21. C. Qin, H. Wu, J. Cheng, X. Chen, M. Liu, W. Zhang, W, Su, and J. Ding, J. Org. Chem., 2007, 72, 4102. CrossRef
22. H.-M. Zheng, Q. Zhang, J.-X. Chen, M.-C. Liu, S.-H. Cheng, J.-C. Ding, H.-Y. Wu, and W.-K. Su, J. Org. Chem., 2009, 74, 943. CrossRef
23. D. Tomita, M. Kanai, and M. Shibasaki, Chem. Asian J., 2006, 1, 161. CrossRef
24. T. Zou, S.-S. Pi, and J.-H. Li, Org. Lett., 2009, 11, 453. CrossRef
25. G. Takahashi, E. Shirakawa, T. Tsuchimoto, and Y. Kawakami, Chem. Commun., 2005, 1459. CrossRef
26. W. Chen, M. Baghbanzadeh, and C. O. Kappe, Tetrahedron Lett., 2011, 52, 1677. CrossRef
27. T. Arao, K. Kondo, and T. Aoyama, Tetrahedron Lett., 2007, 48, 4115. CrossRef
28. K. Hirano, H. Yorimitsu, and K. Oshima, Org. Lett., 2005, 7, 4689. CrossRef
29. K. Yamamoto, K. Tsurumi, F. Sakurai, K. Kondo, and T. Aoyama, Synthesis, 2008, 3585.
30. C.-H. Xing and Q.-S. Hu, Tetrahedron Lett., 2010, 51, 924. CrossRef
31. L. Zhou, X. Du, R. He, Z. Ci, and M. Bao, Tetrahedron Lett., 2009, 50, 406. CrossRef
32. A. Fürstner and H. Krause, Adv. Synth. Catal., 2001, 343, 343. CrossRef
33. N. Imlinger, M. Mayr, D. Wang, K. Wurst, and M. R. Buchmeiser, Adv. Synth. Catal., 2004, 346, 1836. CrossRef
34. M. Kuriyama, R. Shimazawa, and R. Shirai, J. Org. Chem., 2008, 73, 1597. CrossRef
35. M. Kuriyama, R. Shimazawa, T. Enomoto, and R. Shirai, J. Org. Chem., 2008, 73, 6939. CrossRef
36. J. H. Chen, X. Q. Zhang, Q. Feng, and M. M. Luo, J. Organomet. Chem., 2006, 691, 470. CrossRef
37. M. T. Focken, J. Rudolph, and C. Bolm, Synthesis, 2005, 429.
38. İ. Özdemir, S. Demir, and B. Çetinkaya, J. Mol. Catal. A: Chem., 2004, 215, 45. CrossRef
39. M. Yiğit, İ. Özdemir, E. Çetinkaya, and B. Çetinkaya, Transition Met. Chem., 2007, 32, 536. CrossRef
40. H. Türkmen, S. Denizaltı, İ. Özdemir, E. Çetinkaya, and B. Çetinkaya, J. Organomet. Chem., 2008, 693, 425. CrossRef
41. M. Yiğit, İ. Özdemir, E. Çetinkaya, and B. Çetinkaya, Heteroatom Chem., 2005, 16, 461. CrossRef
42. İ. Özdemir, M. Yiğit, E. Çetinkaya, and B. Çetinkaya, Heterocycles, 2006, 68, 1371. CrossRef
43. R. Kılıçarslan, M. Yiğit, İ. Özdemir, E. Çetinkaya, and B. Çetinkaya, J. Heterocycl. Chem., 2007, 44, 69. CrossRef
44. V. R. Jumde, Facchetti, and A. Iuliano, Tetrahedron: Asymmetry, 2010, 21, 2775. CrossRef
45. Y.-X. Liao, C.-H. Chun, and Q.-S. Hu, Org. Lett., 2012, 14, 1544. CrossRef
46. R. Luo, K. Li, Y. Hu, and W. Tang, Adv. Synth. Catal., 2013, 355, 1297. CrossRef
47. N. Khiar, V. Valdivia, A. Salvador, A. Chelouan, A. Alcudia, and I. Fernandez, Adv. Synth. Catal., 2013, 355, 1303. CrossRef
48. M. Ueda and N. Miyaura, J. Organomet. Chem., 2000, 595, 31. CrossRef
49. K. Oguma, M. Miura, T. Satoh, and M. Nomura, J. Organomet. Chem., 2002, 648, 297. CrossRef
50. S. Miyamura, H. Tsurugi, T. Satoh, and M. Miura, J. Organomet. Chem., 2008, 693, 2438. CrossRef
51. K. Ueura, S. Miyamura, T. Satoh, and M. Miura, J. Organomet. Chem., 2006, 691, 2821. CrossRef
52. S. L. X. Martina, R. B. C. Jagt, J. G. De Vries, B. L. Feringa, and A. J. Minnaard, Chemm. Commun., 2006, 4093.
53. A. W. Buesking and J. A. Ellman, Chem. Sci., 2014, 5, 1983. CrossRef
54. S. Demir, M. Yiğit, and İ. Özdemir, J. Organomet. Chem., 2013, 732, 21. CrossRef
55. E. Çetinkaya, P. B. Hitchcock, H. A. Jasim, M. F. Lappert, and K. Spyropoulos, J. Chem. Soc., Perkin Trans. 1, 1992, 561. CrossRef
56. M. Yiğit, İ. Özdemir, B. Çetinkaya, and E. Çetinkaya, J. Mol. Catal. A: Chem., 2005, 241, 88. CrossRef
57. K. Ueura, T. Satoh, and M. Miura, Org. Lett., 2005, 7, 2229. CrossRef
58. T. Jeffery, J. Chem. Soc., Chem. Commun., 1984, 1287. CrossRef
59. J. Chatt and L. M. Venanzi, J. Chem. Soc., 1975, 4735.