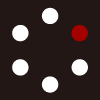
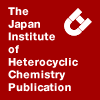
HETEROCYCLES
An International Journal for Reviews and Communications in Heterocyclic ChemistryWeb Edition ISSN: 1881-0942
Published online by The Japan Institute of Heterocyclic Chemistry
e-Journal
Full Text HTML
Received, 5th October, 2014, Accepted, 6th November, 2014, Published online, 17th November, 2014.
DOI: 10.3987/COM-14-13098
■ A Practical and Scalable Process for the Preparation of 4-Aminophenylboronic Acid Pinacol Ester
Feng Xue,* Chang-Gong Li, Yong Zhu, Tian-Jun Lou, and Guang-Jie He*
Key Laboratory of Functional Organic Molecules of Xinxiang City Henan Province, College of Chemistry and Chemical Engineering, Henan Institute of Science and Technology, Xinxiang Henan, 453002, China
Abstract
A practical process has been developed for scalable preparation of 4-aminophenylboronic acid pinacol ester through facile two-step procedure. After boron trifluoride etherate efficiently activated the reaction of 4-bromoaniline with diphenyl ketone, metalation reaction of protected 4-bromoaniline with lithium trialkylmagnesiate readily proceeded under mild conditions (−20 °C), which was subsequently subjected to esterification and deprotection reaction to give the 4-aminophenylboronic acid pinacol ester. The improved synthetic route has been successfully scaled up to kilogram quantities in good yield and high quality.Arylboronic acids and arylboronates have shown great importance due to their widespread applications in organic synthesis and other fields.1,2 In particular, functionalized arylboronic acids and arylboronates are highly valuable because consecutive cross-coupling is possible from these compounds, which can rapidly construct the complex structure of target molecules.3 Of these compounds, 4-aminophenylboronic acid pinacol ester is one of important arylboronates for its versatile applications in a wide variety of biologically active compounds,4 for example piperazine-substituted s-triazine derivatives exhibiting various types of biological properties, such as antiHIV, antituberculosis, anticancer, antimycobacterial, etc.5 In addition, the compound has also been successfully applied in synthesis of fluorescent sensors for heavy and soft metal ions such as mercury.6 Up to now, some successes have been made in the preparation of 4-aminophenylboronic acid pinacol ester,7 typical examples included Raj’s Pd-catalyzed cross-coupling reaction,8 Marder’s Cu-catalyzed borylation reaction,9 Maligre’s Ir-catalyzed C−H bond activation reaction,10 Hsu’s aryl Grignard reagents or aryllithium species with trialkyl boronates,11 Wang’s transition-metal-free transformation from aromatic amines.12 Although these procedures have shown some practical applications in synthesis, their application on a preparative scale has still been limited and deficient in view of laborious synthetic process, harsh reaction conditions, heavy metal contamination, with the result that it is difficult to meet the demands for large-scale preparation. To overcome these problems, we herein wish to describe an improved and efficient process for the scalable preparation of 4-aminophenylboronic acid pinacol ester. With smooth and simple reaction procedures from readily available starting materials, this method of synthesis provides easy access to the target compound (Scheme 1).
Over these years, the reaction of aryl Grignard reagents or aryllithium species with trialkyl boronates remained the most general synthetic method to prepare aromatic boronic compounds in contrast to those catalyzed by heavy metal complex. It was desirable to use readily available aryl bromides as substrates, so we chose 4-bromoaniline as starting material in our synthetic route, 4-bromoaniline was firstly reacted with benzophenone activated by boron trifluoride diethyl etherate (BF3.Et2O), which was more practical in contrast to other activating reagents such as TsOH and 4Å molecular sieve through careful exploration of reaction conditions, to afford 4-bromo-N-(diphenylmethylidene)aniline 2 on large scale and in high yield (Table 1). Meanwhile, compared to the previous route4 in which n-BuLi and trimethylsilyl chloride (TMSCl) was used, the present procedure both smoothed previously-reported harsh reaction conditions and improved the yield of product. Moreover, the diphenyl methylidene group imparted excellent crystallinity to the products, and the free primary amine could be readily liberated by hydrogenolysis under weakly acidic conditions. When the reaction was completed, the recrystallization in methanol conveniently afforded the pure intermediate 2 in 86% yield and high purity (Table 1, entry 3), thus bypassing time-consuming and laborious procedures through column chromatography. It was worthwhile to note that since it was difficult to completely remove residual BF3.Et2O through vacuum evaporation, which could have a negative influence in crystallization course, tertiary amine such as Et3N or i-Pr2NEt with the same equivalent as BF3.Et2O was required to add in methanol to ensure the crystallization repeatable.
Next, our attention was shifted to metalation of the intermediate 2 needed for the subsequent borylation reaction. Although metalation of the intermediate 2 could be performed with n-BuLi under cryogenic conditions (−78 °C), the instability of the resulting organolithium species precluded its use on large quantity, which was apparently inappropriate for industrial large-scale production. We therefore explored an improved route involving metalation for large-scale production to avoid cryogenic conditions (−78 °C). Subsequently, we explored lithium trialkylmagnesiate (R3MgLi) in place of n-BuLi in view of the fact that lithium trialkylmagnesiate are generally more stable than the corresponding organolithium species, and reactions can be performed under noncryogenic conditions in halogen-magnesium exchange.13 To our delight, we found that i-Bu(n-Bu)2MgLi generated in situ readily allowed for metalation under noncryogenic conditions (−20 °C) (Table 2). In our optimized procedure, i-BuMgBr and n-BuLi in a 1:2 ratio were added sequentially to a solution of 2 in THF at −20 °C. This operationally simple process has proved easy to perform on 20 kg scale and obviated the need for a separate vessel to prepare i-Bu(n-Bu)2MgLi as reported previously.14 Thus, the prepared 4-bromo-N-(diphenylmethylidene)aniline 2 was conveniently converted into the corresponding arylboronate through transmetalation, esterification and submitted to acid deprotection to generate 4-aminophneylboronic acid pinacol ester 3 in 70% yield using i-Bu(n-Bu)2MgLi, trimethyl borate and pinacol in dry tetrahydrofuran solvent (Table 2, entry 5), thus making the reaction facile, safe, and easy to scale-up. The large-scale applicability of this improved method has been tested by conducting the reactions on kilogram scale to produce the desired product in high yield and quality.
In conclusion, we have reported a practical two-step process for the scalable preparation of 4-aminophenylboronic acid pinacol ester from 4-bromoaniline. It has been demonstrated that a lithium ate complex, lithium trialkylmagnesiate (i-Bu(n-Bu)2MgLi) instead of n-BuLi, induced the facile halogen-magnesium exchange of 4-bromo-N-(diphenylmethylidene)aniline under noncryogenic conditions. Thus, the reaction proceeded smoothly at - 20 °C, which was capable of delivering a kilogram of the target compound. Compared with the reported procedures, the overall process was more environmentally friendly as well as cost-effective, which enabled the successful delivery of kilogram-scale batches of 4-aminophenylboronic acid pinacol ester for further development studies.
EXPERIMENTAL
Solvents and reagents were purchased and used without further purification. Melting points were determined on a Mettler FP5 melting point apparatus and are uncorrected. NMR spectra were recorded on Bruker DRX 400 MHz spectrometer using CDCl3 as solvent, and tetramethylsilane (TMS) as internal standard. High resolution mass spectra were recorded on Applied Biosystems Mariner System 5303.
4-Bromo-N-(diphenylmethylidene)aniline (2). A 1-L flask equipped with mechanical stirring, thermometer and Dean-Stark trap was charged with 4-bromoaniline 1 (68.0 g, 0.50 mol), benzophenone (109.4 g, 0.60 mol, 1.2 equiv) and BF3.Et2O (5.6 g, 0.04 mol) in toluene (400 mL). After the reaction mixture was refluxed for 12 h, the solvent was removed via vacuum evaporation. The thick yellow residue was added by 5 mL Et3N and then recrystallized with MeOH to afford 4-bromo-N-(diphenyl- methylidene)aniline 2 (145.3 g, 86 % yield) as a yellow solid. mp 83-84 oC (lit.15: 82-83 °C).
4-Aminophenylboronic acid pinacol ester (3). To a solution of isobutylmagnesium bromide (prepared with 3.9 g Mg, 20.4 g isobutylbromide in 60 mL THF) was added n-butyllithium (80.4 g, 2.5 M in hexanes) at 0 °C, and the mixture was stirred for 10 min. The resulting yellow solution was cooled to −20 °C, and a solution of 4-bromo-N-(diphenylmethylidene)aniline 2 (100.8 g, 0.3 mol) in dry THF (600 mL) was introduced dropwise under nitrogen atmosphere. After 1 h of stirring at same temperature, trimethyl borate (47.1 g, 0.45 mol) was added into the reaction mass. The reaction mass was stirred for 1 h at same temperature. The mixture was then warmed to 25 °C over 1 h. After an additional stirring for 30 min at same temperature, the reaction was quenched with cool saturated aqueous NH4Cl solution, then rinsed with EtOAc (3 x 150 mL). The organic solvent was collected, a solution of pinacol (54 g, 0.45 mmol) in EtOAc (60 mL) was added and stirred for 1 h, 156 mL of 10% hydrochloric acid was then added dropwise, and continued to stir for 1 h to ensure complete deprotection, then saturated aqueous NaHCO3 solution was added until the pH reached 7-8. After 45 min of stirring at room temperature, the mixture was diluted with EtOAc, and transferred into a 1-L separatory funnel, then rinsed with EtOAc (3 x 120 mL). The combined organic phases were dried, filtered and concentrated under reduced pressure. The residue was recrystallized in ethanol to afford 4-aminophenylboronic acid pinacol ester 3 (45.8 g, 70 % yield) as a light yellow solid, mp 164–166 °C (lit.4: 162–165 °C). 1H NMR (400 MHz, CDCl3): δ 7.63 (d, J = 8.0 Hz, 2H), 6.65 (d, J = 8.0 Hz, 2H), 3.83 (b, 2H, NH2), 1.32 (s, 12H, CH3). 13C NMR (100 MHz, CDCl3): δ 156.9 (1C, C-NH2), 150.4 (1C, C–B, broad), 141.9, 123.3 (4C, Ar–C), 83.1 (2C, O–C (CH3)2), 23.9 (4C, CH3).
ACKNOWLEDGEMENTS
This work was supported by the National Natural Science Foundation of China (21072046 and 21371148).
References
1. D. G. Hall, Boronic Acids: Preparation and Applications in Organic Synthesis, Medicine and Materials, 2nd ed.; Wiley-VCH: Weinheim, 2011. CrossRef
2. A. Suzuki, Acc. Chem. Res., 1982, 15, 178; CrossRef N. Miyaura and A. Suzuki, Chem. Rev., 1995, 95, 2457; CrossRef T. Ishiyama and N. Miyaura, Chem. Rev., 2004, 104, 271; N. Miyaura, Bull. Chem. Soc. Jpn., 2008, 81, 1535. CrossRef
3. M. Tobisu and N. Chatani, Angew. Chem. Int. Ed., 2009, 48, 3565; CrossRef C. Wang and F. Glorius, Angew. Chem. Int. Ed., 2009, 48, 5240; CrossRef P. Merino and T. Tejero, Angew. Chem. Int. Ed., 2010, 49, 7164. CrossRef
4. A. B. Patel, R. V. Patel, P. Kumari, D. P. Rajani, and K. H. Chikhalia, Med. Chem. Res., 2013, 22, 367. CrossRef
5. A. Solankee, K. Kapadia, A. Ciric, M. Sokovic, I. Doytchinova, and A.Geronikaki, Eur. J. Med. Chem., 2010, 45, 510; CrossRef N. Sunduru, L. Gupta, V. Chaturvedi, R. Dwivedi, S. Sinha, and P. Chauhan, Eur. J. Med. Chem., 2010, 45, 3335. CrossRef
6. B. Vandana, K. Manoj, M. P. Rakesh, R. Kumar, and T. Ruchi, Tetrahedron Lett., 2009, 50, 2649.. CrossRef
7. F. Fache, E. Schulz, M. L.Tommasino, and M. Lemaire, Chem. Rev., 2000, 100, 2159. CrossRef
8. B. Vandana, K. Manoj, K. Naresh, S. Hardev, K. Tandeep, and S. P. Raj, Org. Lett., 2011, 13, 1422. CrossRef
9. C. Kleeberg, L. Dang, Z. Lin, and T. B. Marder, Angew. Chem. Int. Ed., 2009, 48, 5350. CrossRef
10. S. M. Preshlock, D. L. Plattner, P. E. Maligres, S. W. Krska, R. E. Malezka Jr., M. R. Smith Ⅲ, S. W. Krska, and P. E. Maligres, Angew. Chem. Int. Ed., 2013, 52, 12915.
11. C. N. Lo and C. S. Hsu, J. Polym. Sci. Pol. Chem., 2011, 49, 3355. CrossRef
12. L. Jin, H. Meng, F. Y. Mo, D. Qiu, S. B. Tang, and J. B. Wang, Angew. Chem. Int. Ed., 2013, 52, 11581. CrossRef
13. F. Gallou, R. Haenggi, H. Hirt, W. Marterer, F. Schaefer, and M. S. Weibel, Tetrahedron Lett., 2008, 49, 5024. CrossRef
14. O. Bayh, H. Awad, F. Mongin, C. Hoarau, L. Bischoff, F. Trecourt, G. Queguiner, F. Marsais, F. Blanco, B. Abarca, and R. Ballesteros, J. Org. Chem., 2005, 70, 5190. CrossRef
15. J. P. Sadighi, R. A. Singer, and S. L. Buchwald, J. Am. Chem. Soc., 1998, 120, 4960. CrossRef