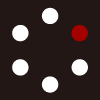
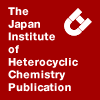
HETEROCYCLES
An International Journal for Reviews and Communications in Heterocyclic ChemistryWeb Edition ISSN: 1881-0942
Published online by The Japan Institute of Heterocyclic Chemistry
e-Journal
Full Text HTML
Received, 16th October, 2014, Accepted, 3rd December, 2014, Published online, 11th December, 2014.
DOI: 10.3987/COM-14-13107
■ γ-Lactonic and Linear Carboxylic Acids from Cultured Lichen Mycobionts of Graphis scripta
Yukiko Takenaka, Nobuo Hamada, and Takao Tanahashi*
Organic chemistry, Kobe Pharmaceutical University, 4-19-1 Motoyamakita-machi, Higashinada-ku, Kobe 658-8558, Japan
Abstract
Spore-derived mycobionts of the lichen Graphis scripta collected in Nikko, Japan, were cultivated on a malt-yeast extract medium supplemented with 10% sucrose to produce three new carboxylic acids 1–3. Their structures were characterized by chemical and spectroscopic methods. These compounds are prototypes of characteristic lichen substances, γ-lactonic and linear carboxylic acids with a long side chain, and were obtained as enantiomeric mixtures.Lichens, several of which have important medicinal usages as crude drugs, produce characteristic secondary metabolites such as depsides, depsidones, dibenzofuran and γ-lactone carboxylic acids, so-called lichen substances. Some lichen substances have been found to exhibit a wide range of potentially useful biological activities.1-5 It is well known that some of these secondary metabolites are produced by not only lichen thalli, symbiotic organisms of fungi (mycobiont) and algae (photobiont), but also isolated cultured mycobionts of lichens independent of their photobionts.6
Furthermore, cultures of isolated lichen mycobionts have the ability under osmotically stressed conditions to produce substances that have never been detected in the lichenized state but are structurally related to fungal metabolites.6-8 From our interest in the metabolic capability of isolated lichen mycobionts, we previously cultivated the mycobionts of many lichen species collected in Japan and other countries and isolated diverse metabolites from the cultures.9-11 In the course of our studies on cultured lichen mycobionts of the genus Graphis,12-15 we cultivated the spore-derived mycobiont of Graphis scripta to investigate its metabolites. We report here the characterization of new carboxylic acids.
A polyspore-derived mycobiont of Graphis scripta collected in Nikko, Japan, was cultured on conventional malt-yeast extract medium supplemented with 10% sucrose at 18 °C in the dark. After cultivation for 6 months, the colonies and agar medium were extracted with Et2O and with acetone. The extracts were separated by a combination of preparative TLC and preparative HPLC to afford three aliphatic compounds 1–3.
Compound 1 was isolated as a colorless crystalline solid and the molecular formula was established as C7H8O4 by HR-CIMS. Its UV spectrum showed a maximum at 227 nm, and its IR spectrum exhibited absorption bands at 3004 and 1732 cm-1, indicating that 1 possessed carboxylic acid and α,β-unsaturated lactone moieties.1 The presence of a carboxyl group was confirmed by methylation of 1 to a monomethyl ester 4. The 1H NMR spectrum of 1 exhibited signals for a carboxyl group at δ 9.26 (br), a vinyl methyl group at δ 2.25 (d, J=2.0 Hz) and a secondary methyl group at δ 1.58 (d, J=6.5 Hz) coupled with an oxygenated methine proton at δ 5.21 (qq, J=6.5, 2.0 Hz). Its 13C NMR spectrum showed seven carbon signals, and DEPT measurement revealed the presence of two methyl groups, an oxygenated methine and four quaternary carbons, which were assigned to carboxylic acid, lactone carbonyl and two olefin carbons. In the HMBC spectrum, the methine proton was correlated with a secondary methyl group and three quaternary carbons, but not with a vinyl methyl carbon, and the vinyl methyl protons were correlated with two olefinic and one lactone carbonyl carbons, confirming the same substituted pattern on the γ-lactone ring as in lichesterinic acid (5).16 Chiral HPLC analysis of 4 demonstrated two peaks, suggesting that the isolated compound 1 was a mixture of enantiomers. This was supported by the fact that the 1H NMR spectrum of the phenylglycine methyl ester (PGME) amides of 1 showed duplicate signals due to two diastereomers 6a and 6b at a ratio of 7:3. The absolute configuration of the major enantiomer was estimated to be 4S by the negative sign of optical rotation of 1 ([α]D -17), since the optical rotations of (4R)- and (4S)-2,4-dimethyl-5-oxo-2,5-dihydrofuran-3-carboxylic acid were reported to be +29.7 and -32.4, respectively.17 Thus, compound 1 was elucidated to be a mixture of (4R)- and (4S)-2,4-dimethyl-5-oxo-2,5-dihydrofuran-3-carboxylic acids at a ratio of 3:7. Some metabolites have been isolated from the cultured mycobionts in the form of enantiomeric mixtures.13 2,4-Dimethyl-5-oxo-2,5-dihydrofuran-3-carboxylic acids have been synthesized as derivatives of latifolin17 and rubrenolide, 18 but were isolated as natural products for the first time.
Compound 2 was obtained as an apparent mixture containing ca. 30% 1, which could not easily be purified by TLC with various solvent systems. Repeated purification by reverse-phase HPLC gave a tiny amount of pure 2. Detailed characterization of 2 was therefore performed as a mixture. The HR-CIMS data established the molecular formula of 2 to be C7H10O4. The 1H NMR spectrum exhibited signals for two secondary methyl groups at δ 1.39 and 1.56 (each d), three methine protons at δ 2.70, 3.03 and 4.60, and a carboxylic proton at δ 9.26, thus indicating 2 to be a 2,3-dihydro derivative of 1. This was supported by its 13C NMR spectral data as well as 1H-1H COSY, HMQC and HMBC experiments. Methylation to methyl ester 7 implied the presence of a carboxyl group in 2. The relative configurations of 2 were determined by NOESY experiments. The important NOE cross-peaks observed between H-3 and H3-5, H3-6 and between H-2 and H-4 demonstrated that H-2 and H-4 exist on the same face as the carboxyl group at C-3. The 2,3-trans and 3,4-trans configuration was further supported by the comparison of NMR data of 2 and roccellaric acid (8).19 For determination of the absolute configuration, the mixture of 2 and 1 was treated with (R)-PGME in the presence of PyBOP, HBT and TEA to give a diastereomeric mixture (10:9) of (R)-PGME amides,20 (R)-9a and (R)-9b together with a mixture of (R)-6a and (R)-6b. (S)-PGME amides, (S)-9a, (S)-9b, (S)-6a, and (S)-6b were prepared in the same way. The 1H NMR spectral features of (R)-9a and (R)-9b coincided with those of (S)-9b and (S)-9a, respectively, indicating 2 to be an enantiomeric mixture of 2a and 2b. The absolute configurations of C-3 of 2a and 2b were determined to be R and S, respectively, by differences in the chemical shifts of the corresponding proton signals between (S)-9a and (R)-9a and between (S)-9b and (R)-9b.20
Thus, the new compound 2 was elucidated to be a mixture of (2S,3S,4R)- and (2R,3R,4S)-2,4-dimethyl- 5-oxotetrahydrofuran-3-carboxylic acids at a ratio of 10:9. Its stereoisomers have already been synthesized as model compounds of phaseolinic acid (10).21
In the HR-CIMS, compound 3 showed a pseudomolecular ion at m/z 159.0678, corresponding to the molecular formula C7H10O4. Its 1H NMR spectrum exhibited signals for exomethylene protons at δ 5.69, and 6.25 (each br s), a methine proton at δ 3.33 and its connected ethyl group at δ 0.94 (3H), 1.71 (1H) and 1.89 (1H). The 13C NMR spectrum of 3 showed three sp3 carbons assigned to a methyl, a methylene and a methine carbon, and four sp2 carbons, two of which were carbonyl carbons. These NMR spectral features together with its structural formula and HMBC correlations suggested a dicarboxylic acid structure similar to (-)-2-methylene-(3R)-carboxy-(18R)-hydroxynonadecanoic acid (11). Methylation of 3 with TMS-CHN2 could not give an expected dimethyl ester 12, but a cyclized compound 13 as a diastereomeric mixture at a ratio of 2:1, while treatment of 3 with HCl in MeOH afforded 12. The enantiomeric purity of 3 was estimated by chiral HPLC analysis of 12, which demonstrated 3 to be an enantiomeric mixture with a ratio of 73:27. To determine the absolute configuration of C-3, compound 3 was treated with with (R)-PGME to yield a mixture of isomers 14 and 15, but not the desired amide 16. Therefore, it remained to be determined which enantiomer is predominant, although the absolute configuration of the major enantiomer was presumed to be R by comparison with the sign of the optical rotation for (2S)-butylitaconic acid ([α]D +10.3, MeOH).22 Thus, compound 3 was characterized as an enantiomeric mixture of ethylitaconic acid. This is the first instance of the isolation of 3 from natural sources, although (±)-ethylitaconic acid has already been synthesized as a plant growth regulator.23
γ-Butyrolactones constitute an important group of natural products with wide-ranging biological activities including antitumor and antifungal. Several γ-lactones with a long side chain, such as lichesterinic acid (5) and roccellaric acid (8), have been isolated as typical lichen substances from natural lichens.1 The γ-lactone with a C5 side chain, phaseolinic acid (10), has been isolated from the fungus Penicillium sp.24 Linear carboxylic acids such as 11 and tensyuic acid (17)25 are also known as lichen and fungal substances. These carboxylic acids with a various length side chain might most likely be biosynthesized from the appropriate C8-C18 fatty acid unit and a C3-unit originated from oxaloactate.26 It is noteworthy that the metabolites isolated here from the cultured mycobionts of Graphis scripta possess the shortest alkyl substituent derived from two acetate units and could be recognized as prototypes of these types of carboxylic acids. The occurrence of these γ-lactones and a dicarboxylic acid in the cultures of lichen mycobionts is of great interest in terms of the evolution of lichenized fungi and free-living fungi.
EXPERIMENTAL
General Procedures. The UV spectra were recorded on a Shimadzu UV-240 spectrophotometer and the IR spectra on a Shimadzu FTIR-8200 infrared spectrophotometer. The optical rotation was measured on a Jasco DIP-370 digital polarimeter. EIMS, CIMS, HR-EIMS and HR-CIMS were obtained with a Hitachi M-4100 mass spectrometer. CIMS were run with iso-butane as the reagent gas. The NMR experiments were performed with Varian Gemini-200, Varian Gemini-300, Varian VXR-500 and Varian UNITY INOVA (500 MHz) spectrometers with tetramethylsilane as an internal standard. HPLC was performed using a Waters system (510 HPLC Pump, 486 Tunable Absorbance Detector). Thin-layer chromatography was performed on precoated Kieselgel 60F254 plates (Merck) and spots were visualized under UV light or by treatment with I2 vapor.
Plant Material. Specimens of Graphis scripta (L.) Ach. were collected from the bark of trees in Nikko, Tochigi Prefecture, Japan. The voucher specimen was identified by Prof. M. Nakanishi of Hiroshima University, Japan and was deposited at Saga University, Japan, with the registration No. NH9893016. Mycobionts were obtained from the spores discharged from apothecia of a thallus, and were cultivated in 80 test tubes containing modified MY10 medium (malt extract 10 g, yeast extract 4 g, sucrose 100 g, agar 15 g, H2O 1 l, pH 7.0) at 18 °C in the dark. After cultivation for 6 months, the colonies and slants were harvested.
Isolation of compounds. The harvested colonies (dry weight 13.38 g) were extracted continuously with Et2O and then with acetone. The Et2O and acetone extracts were concentrated to give residues R-1 (137.4 mg) and R-2 (538.9 mg), respectively. The slants were collected and extracted with acetone. The acetone extracts were concentrated and re-extracted with Et2O to give a residue R-3 (1.189 g). The residues R-1, R-2 and R-3 were repeatedly subjected to preparative TLC (toluene-acetone 8:2, toluene-AcOH 9:1) and preparative HPLC (µBondasphere 5µ C18-100Å, H2O-MeCN, 6:4 or 7:3), giving rise to 1 (R-1: 26.6 mg, R-2: 8.4 mg, R-3: 137.9 mg), a mixture of 1 and 2 (R-1: 33.0 mg, R-2: 49.8 mg, R-3: 315.1 mg) and 3 (R-1: 5.3 mg, R-2: 33.7 mg, R-3: 118.9 mg). The mixture (50.0 mg) of 1 and 2 was repeatedly subjected to preparative HPLC (Cosmosil 5C18-MS-II, 20 i.d. x 250 mm; H2O-MeOH-AcOH, 30:70:0.7; detection 220 nm) to give 2 (1.4 mg) and 1 (7.5 mg).
1: Colorless crystalline solid, mp 173 °C (MeOH). [α]D21 - 17 (c 1.34, CHCl3). UV (EtOH) λmax nm (log ε): 227 (4.08). IR (KBr) νmaxcm-1: 3004, 1732. 1H NMR (CDCl3): δ 1.58 (3H, d, J=6.5 Hz, H3-5), 2.25 (3H, d, J=2.0 Hz, H3-6), 5.21 (1H, qq, J=6.5, 2.0 Hz, H-4), 9.26 (1H, br, OH). 13C NMR (CDCl3): δ 11.0 (C-6), 18.9 (C-5), 77.8 (C-4), 139.7 (C-2), 147.9 (C-3), 166.6 (C-7), 172.6 (C-1). HR-CIMS m/z: Calcd for C7H9O4 [M+H]+: 157.0501. Found: 157.0519.
2: Colorless oil, [α]D21 + 3 (c 0.14, MeOH). 1H NMR (CDCl3): δ 1.39 (3H, d, J=7.0 Hz, H3-6), 1.56 (3H, d, J=6.0 Hz, H3-5), 2.70 (1H, dd, J=11.5, 10.0 Hz, H-3), 3.03 (1H, dq, J=11.5, 7.0 Hz, H-2), 4.60 (1H, dq, J=10.0, 6.0 Hz, H-4), 9.26 (1H, br, OH). 1H NMR (300 MHz, CD3OD): δ 1.25 (3H, d, J=6.9 Hz, H3-6), 1.46 (3H, d, J=6.0 Hz, H3-5), 2.55 (1H, dd, J=11.4, 9.6 Hz, H-3), 2.97 (1H, dq, J=11.4, 6.9 Hz, H-2), 4.53 (1H, dq, J=9.6, 6.0 Hz, H-4). 13C NMR (CDCl3): δ 14.4 (C-6), 20.2 (C-5), 39.8 (C-2), 55.5 (C-3), 75.7 (C-4), 175.7 (C-7), 176.7 (C-1). HR-CIMS m/z: Calcd for C7H11O4 [M+H]+: 159.0658. Found: 159.0673.
3: Colorless oil, [α]D26 - 3 (c 1.8, MeOH). UV (EtOH) λmax nm (log ε): 201 (3.84). IR (CHCl3) νmax cm-1: 2983, 1773, 1739, 1717, 1637, 1564.1H NMR (CD3OD): δ 0.94 (3H, t, J=7.5 Hz, H3-5), 1.71, 1.89 (each 1H, dquint, J=15.5, 7.5 Hz, H2-4), 3.33 (1H, br t, J=7.5 Hz, H-3), 5.69, 6.25 (each 1H, br s, H2-6). 13C NMR (CD3OD): δ 12.6 (C-5), 25.4 (C-4), 51.1 (C-3), 126.4 (C-6), 141.5 (C-2), 171.0 (br, C-1), 178.0 (br, C-7). HR-CIMS m/z: Calcd for C7H11O4 [M+H]+: 159.0658. Found: 159.0678.
Methylation of 1 with TMS-CHN2. A solution of 1 (6.4 mg) in MeOH was treated with TMS-CHN2 to give 4 (6.0 mg). 4: oil, IR (CHCl3) νmax cm-1: 1769, 1724. 1H NMR (200 MHz, CDCl3): δ 1.53 (3H, d, J=6.0 Hz, H3-5), 2.21 (3H, d, J=2.0 Hz, H3-6), 3.89 (3H, s, 7-OCH3), 5.17 (1H, m, H-4). HR-CIMS m/z: Calcd for C8H11O4 [M+H]+: 171.0658. Found: 171.0652. Compound 4 was analyzed by chiral HPLC [column, CHIRALCEL OB-H (4.6 i.d. x 250 mm, Daicel Chemical Industries, Ltd.); mobile phase, n-hexane-2-propanol (8:2); flow rate, 0.6 mL/min; detection, 254 nm] and demonstrated a major peak at 24 min and a minor one at 28 min.
Methylation of 2 with TMS-CHN2. A solution of 1 and 2 (39.9 mg) in MeOH was treated with TMS-CHN2 to give an inseparable mixture of 4 and 7 (34.3 mg). Compound 7 was characterized without purification. 7: 1H NMR (200 MHz, CDCl3): δ 1.34 (3H, d, J=7.5 Hz, H3-6), 1.50 (3H, d, J=6.0 Hz, H3-5), 2.62 (1H, dd, J=11.0, 9.0 Hz, H-3), 2.98 (1H, dq, J=11.0, 7.5 Hz, H-2), 3.79 (3H, s, 7-OCH3), 4.64 (1H, dq, J=9.0, 6.0 Hz, H-4). HR-CIMS m/z: Calcd for C8H13O4 [M+H]+: 173.0814. Found: 173.0822.
Preparation of (R)-PGME amides (R)-6a, (R)-6b, (R)-9a and (R)-9b. To a mixture of 1 and 2 (15.5 mg) in DMF (1.0 mL) were added (R)-PGME·HCl (D-(-)-α-phenylglycine methyl ester hydrochloride, 60 mg), PyBOP (benzotriazol-1-yl-oxy-tris(pyrolidino)phosphonium hexafluorophosphate, 130 mg), HBT (1-hydroxybenzotriazole, 32 mg) and TEA (4 drops), which was then stirred at room temperature for 8.5 h. The reaction mixture was poured into 1N HCl and extracted with CHCl3. The CHCl3 layer was dried and concentrated in vacuo. The residue was purified by preparative TLC (Et2O), giving an inseparable mixture of (R)-6a, (R)-6b (7:3) and (R)-9a, (R)-9b (10:9) (15.1 mg). (R)-6a: 1H NMR (CDCl3): δ 1.506 (3H, d, J=6.5 Hz, H3-5), 2.129 (3H, d, J=2.0 Hz, H3-6), 5.209 (1H, qq, J=6.5, 2.0 Hz, H-4), 3.780 (3H, s, PGME-OCH3), 5.651 (1H, br d, J=6.5 Hz, PGME-CH), 6.927 (1H, br d, J=6.0 Hz, PGME-NH), 7.32-7.42 (5H, m, PGME-Ph). (R)-6b: 1H NMR (CDCl3) δ 1.456 (3H, d, J=6.5 Hz, H3-5), 2.163 (3H, d, J=2.0 Hz, H3-6), 5.225 (1H, qq, J=6.5, 2.0 Hz, H-4), 3.775 (3H, s, PGME-OCH3), 5.677 (1H, br d, J=7.0 Hz, PGME-CH), 6.878 (1H, br d, J=6.0 Hz, PGME-NH), 7.32-7.42 (5H, m, PGME-Ph). HR-EIMS m/z: Calcd for C16H17NO5 [M]+: 303.1107. Found: 303.1117. (R)-9a: 1H NMR (CDCl3): δ 1.333 (3H, d, J=7.5 Hz, H3-5), 1.378 (3H, d, J=7.0 Hz, H3-6), 2.446 (1H, dd, J=11.5, 9.5 Hz, H-3), 3.077 (1H, dq, J=11.5, 7.5 Hz, H-2), 3.752 (3H, s, PGME-OCH3), 4.509 (1H, dq, J=9.5, 7.0 Hz, H-4), 5.582 (1H, br d, J=7.0 Hz, PGME-CH), 6.692 (1H, br d, J=6.0 Hz, PGME-NH), 7.32-7.42 (5H, m, PGME-Ph). (R)-9b: 1H NMR (CDCl3): δ 1.203 (3H, d, J=7.0 Hz, H3-5), 1.513 (3H, d, J=6.0 Hz, H3-6), 2.446 (1H, dd, J=11.5, 9.5 Hz, H-3), 3.034 (1H, dq, J=11.5, 7.0 Hz, H-2), 3.752 (3H, s, PGME-OCH3), 4.565 (1H, dq, J=9.5, 6.0 Hz, H-4), 5.582 (1H, br d, J=7.0 Hz, PGME-CH), 6.719 (1H, br d, J=6.0 Hz, PGME-NH), 7.32-7.42 (5H, m, PGME-Ph). HR-EIMS m/z: Calcd for C16H19NO5 [M]+: 305.1264. Found: 305.1243.
Preparation of (S)-PGME amides (S)-6a, (S)-6b, (S)-9a and (S)-9b. (S)-PGME·HCl was prepared from (L)-(+)-α-phenylglycine and SOCl2 in MeOH according to Nagai and Kusumi.20 To a solution of 1 and 2 (15.0 mg) in DMF (1.0 mL) were added (S)-PGME·HCl (60 mg), PyBOP (100 mg), HBT (30 mg) and TEA (2 drops), which was then stirred at room temperature for 8.5 h and worked up in the same way as described above. The resulting residue was subjected to preparative TLC (Et2O) to give (S)-6a, (S)-6b, (S)-9a and (S)-9b (13.5 mg). The 1H NMR spectral data of (S)-6a, (S)-6b, (S)-9a and (S)-9b were identical to those of (R)-6b, (R)-6a, (R)-9b and (R)-9a, respectively.
Methylation of 3 with TMS-CHN2. A solution of 3 (5.3 mg) in MeOH was treated with TMS-CHN2 to give 13 (3.7 mg). 13: 1H NMR (CDCl3): δ 0.98 (3H, d, J=7.0 Hz, H3-5), 1.67 (1H, m, H2-4), 1.99, 2.05 (1H, m, H-3), 2.14*, 2.20 (1H, m, H-2), 3.62, 3.78* (3H, s, OCH3), 4.48, 4.57* (1H, dd, J=9.0, 7.5 Hz, H-6), 4.75*, 4.84 (1H, dd, J=9.0, 4.5 Hz, H-6). *Major product:minor product = 2:1.
Methylation of 3 with HCl-MeOH. A solution of 3 (7.0 mg) in 5% HCl-MeOH was heated at 80 °C for 5 min. After concentration of the solvent, the resulting residue was purified by preparative TLC (toluene-acetone 4:1) to afford 12 (2.0 mg). 12: 1H NMR (300 MHz, CDCl3): δ 0.93 (3H, t, J=7.5 Hz, H3-5), 1.71, 1.93 (each 1H, dquint, J=14.0, 7.5 Hz, H2-4) 3.44 (1H, br t, J=7.5 Hz, H-3), 3.69, 3.77 (each 3H, s, 2 x OMe), 5.75, 6.37 (each 1H, br s, H2-6). CIMS m/z: 187 [M+H]+. Compound 12 was analyzed by chiral HPLC [column, CHIRALCEL OB-H (4.6 i.d. x 250 mm, Daicel Chemical Industries, Ltd.); mobile phase, n-hexane-2-propanol (19:1); flow rate, 0.6 mL/min; detection, 220 nm] and demonstrated a major peak at 14 min and a minor one at 16 min at a ratio of 73:27.
Preparation of (R)-PGME amides 14 and 15 from 3. To a solution of 3 (8.9 mg) in DMF (1.0 mL) were added (R)-PGME·HCl (39 mg), PyBOP (88 mg), HBT (25 mg) and TEA (1 drop), which was then stirred at room temperature for 4 h. The reaction mixture was worked up in the same way as described above and the resulting residue was subjected to preparative TLC (Et2O) to give a mixture of 16 and 17 (5.4 mg). 16 and 17: 1H NMR (200MHz, CDCl3): δ 1.02, 1.03* (3H, t, J=7.5 Hz, H3-5), 1.93 (3H, s, H3-6), 2.30, 2.34* (2H, br q, J=7.5 Hz, H2-4), 3.54, 3.55, 3.699*, 3.700* (each 3H, s, 2 x PGME-OCH3), 5.35, 5.40, 5.43*, 5.44* (each 1H, br d, J=7.0 Hz, 2 x PGME-CH), 6.78*, 6.81, 6.83*, 6.84 (each 1H, br d, J=7.0 Hz, PGME-NH), 7.20-7.35 (10H, m, PGME-Ph). *Major product:minor product = 5:1. HR-CIMS m/z: Calcd for C25H28N2O6 [M+H]+: 452.1948. Found: 452.1961.
ACKNOWLEDGEMENTS
We are grateful to Prof. M. Nakanishi (Hiroshima University, Japan) for identification of the voucher specimen. Thanks are also due to Dr. M. Sugiura (Kobe Pharmaceutical University) for 1H and 13C NMR spectra, and to Dr. K. Saiki (Kobe Pharmaceutical University) for mass spectral measurements. This research was financially supported by Kobe Pharmaceutical University Collaboration Fund.
References
1. S. Huneck, Progress in the Chemistry of Organic Natural Products, Vol. 81, pp.1-313, ed. by W. Herz, H. Falk, G. W. Kirby, and R. E. Moore, Springer Verlag, Wien, New York (2001).
2. S. Huneck, Naturwissenshaften, 1999, 86, 559. CrossRef
3. K. Molnár and E. Frakas, Z. Naturforsch., 2010, 65c, 157.
4. K. Müller, Appl. Microbiol. Biotechnol., 2001, 56, 9. CrossRef
5. V. Shukla, G. P. Joshi, and M. S. M. Rawat, Phytochem. Rev., 2010, 9, 303. CrossRef
6. E. Stocker-Wörgötter, Nat. Prod. Rep., 2008, 25, 188. CrossRef
7. H. Miyagawa, N. Hamada, M. Sato, and T. Ueno, Phytochemistry, 1994, 36, 1319. CrossRef
8. T. Tanahashi, M. Kuroishi, A. Kuwahara, N. Nagakura, and N. Hamada, Chem. Pharm. Bull., 1997, 45, 1183. CrossRef
9. D. H. Le, Y. Takenaka, N. Hamada, and T. Tanahashi, Phytochemistry, 2013, 91, 242. CrossRef
10. Y. Takenaka, Y. Naito, D. H. Le, N. Hamada, and T. Tanahashi, Heterocycles, 2013, 87, 1897. CrossRef
11. D. H. Le, Y. Takenaka, N. Hamada, Y. Mizushina, and T. Tanahashi, J. Nat. Prod., 2014, 77, 1404. CrossRef
12. Y. Takenaka, N. Morimoto, N. Hamada, and T. Tanahashi, Phytochemistry, 2011, 72, 1431. CrossRef
13. Y. Takenaka, N. Hamada, and T. Tanahashi, Heterocycles, 2011, 83, 2157. CrossRef
14. D. H. Le, Y. Takenaka, N. Hamada, H. Miyawaki, and T. Tanahashi, Chem. Pharm. Bull., 2013, 61, 358. CrossRef
15. Y. Takenaka, S. Taguchi, D. H. Le, N. Hamada, and T. Tanahashi, Heterocycles, 2013, 87, 2651. CrossRef
16. S. Huneck and R. Takeda, Z. Naturforsch., 1992, 47b, 842.
17. T. Matsumoto, T. Okabe, and K. Fukui, Chem. Lett., 1973, 773. CrossRef
18. S. A. M. T. Hussain, W. D. Ollis, C. Smith, and F. Stoddart, J. Chem. Soc., Perkin Trans. 1, 1975, 1480. CrossRef
19. J. P. Devlin, C. P. Falschaw, W. D. Ollis, and R. E. Wheeler, J. Chem. Soc. (C), 1971, 1318. CrossRef
20. Y. Nagai and T. Kusumi, Tetrahedron Lett., 1995, 36, 1853. CrossRef
21. P. A. Jacobi and P. Herradura, Tetrahedron Lett., 1996, 37, 8297. CrossRef
22. G. Li, S. Kusari, M. Lamshöft, A. Schüffler, H. Laatsch, and M. Spiteller, J. Nat. Prod., 2014, 77, 2335. CrossRef
23. K. Nanjo, S. Koura, A. Suzuki, A. Isogai, and J. Gen, Jpn. Kokai Tokkyo Koho, 1987, JP 6213804A 19870616.
24. B. K. Park, M. Nakagawa, A. Hirota, and M. Nakayama, J. Antibiot., 1988, 41, 751. CrossRef
25. Y. Hasegawa, T. Fukuda, K. Hagimori, H. Tomoda, and S. Omura, Chem. Pharm. Bull., 2007, 55, 1383. CrossRef
26. N. C. J. E. Chesters and D. O’Hagan, J. Chem. Soc., Perkin Trans. 1, 1997, 827. CrossRef