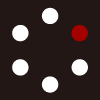
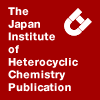
HETEROCYCLES
An International Journal for Reviews and Communications in Heterocyclic ChemistryWeb Edition ISSN: 1881-0942
Published online by The Japan Institute of Heterocyclic Chemistry
e-Journal
Full Text HTML
Received, 1st November, 2014, Accepted, 2nd December, 2014, Published online, 5th December, 2014.
DOI: 10.3987/COM-14-13117
■ A New and Practical Synthesis of Bosutinib
Han Wang, Chunping Zhu, Guoqing Zhu, Xin Tian, Yongjia Shen,* and Yongjun Mao*
College of Chemistry and Chemical Engineering, Shanghai University of Engineering Science, 333 Longteng Rd., Songjiang, Shanghai, 201620, China
Abstract
New and improved synthetic route of bosutinib is described on a decagram scale. An intramolecular cyclization of 3-amino-2-(2-bromobenzoyl)- acrylonitrile (22) in K2CO3/DMF condition to form the key 3-cyano-4-hydroxyquinoline intermediate (13) is adopted as the key step. Bosutinib is obtained in 13.7% yield over ten steps and 98.9% purity (HPLC), which make it as a process of cost effective, environmentally friendly and feasible for scale-up operation.Bosutinib (1, SKI-606, Scheme 1) marketed as Bosulif ®, is an ATP-competitive Bcr-Abl tyrosine-kinase inhibitor with an additional inhibitory effect on Src family kinases (including Src, Lyn and Hck) for use in the treatment of cancer.1 Bosulif ® was originally developed by Wyeth Pharmaceuticals and received the US FDA and EU European Medicines Agency approval on 2012 and 2013 respectively for the treatment of adult patients with Philadelphia chromosome-positive (Ph+) chronic myelogenous leukemia (CML) with resistance, or intolerance to prior therapy.2
A couple of synthetic route of 1 were developed on a multi-grams scale, while a practical synthetic process is needed. The earlier and common work to prepare 1 was based on the Gould-Jacobs methodology through a thermal cyclization at 250 ºC for 4 h in Dowtherm A to synthesize the key intermediate 7-fluoro-4-hydroxy-6-methoxyquinoline-3-carbonitrile (5) (Scheme 1).3 The main problem was that the high reaction temperature leaded to a messy and tedious operation and too many materials were destroyed in the reaction as tar or resin, which resulted in difficulties for purification and thus the overall yield was reduced dramatically. Withbroe et al.4 developed a streamlined process for the synthesis and isolation of bosutinib monohydrate (Scheme 2). A three-component coupling reaction of 6 with cyanoacetamide 7 and triethyl orthoformate led to 8 as a mixture of cis/trans isomers. Cyclization of 8 using POCl3 in sulfolane provided the core structure of 1. Yin et al.5 also reported a new synthesis of 1 (Scheme 3), which started with esterification of the material vanillic acid (9), followed by alkylation, nitration, reduction to give the methyl 2-aminobenzoate 10. Condensation of 10 with 3,3-diethoxypropanenitrile (11) in TFA, followed by cyclization of 12 in NaOH/EtOH gave 3-cyano-4-hydroxyquinoline compound 13 in around 40% overall yield from 9. Bosutinib was eventually obtained in 16% yield from 9 after chlorination and two consecutive aminations.
In order to develop a practical and commercial process of preparing compound 1, a new and practical synthetic route was established, which adopted an intramolecular cyclization of 3-amino-2- (2-bromobenzoyl)acrylonitrile 22 in K2CO3/DMF condition to form the key 3-cyano-4-hydroxyquinoline intermediate 13, as shown in Scheme 4.
Methyl vanillate (14) was used as the starting material, which was reacted with 1-bromo-3-chloropropane in K2CO3/DMF to give compound 15 in 91% isolated yield, based on the reported method 5 as well as our optimization.6 The next bromination was carried out by Br2 in AcOH at room temperature, compound 16 was obtained in 89% isolated yield. The benzoic acid 17 was obtained from 16 in 93% yield through the basic ester hydrolysis. By treating 17 with SOCl2 and following reaction with ethyl cyanoacetate and NaOEt, compound 19 was achieved (as the enol form confirmed by 1H NMR), which was purified by recrystallization from hexane/EtOAc in fair yield (77% over two steps). Heating 19 with 90% DMSO/H2O solution provided the compound 20 in 81% yield after recrystallization from hexane/EtOAc. 20 was then condensed with CH(OMe)3 and Ac2O, substituted by NH3 in MeOH to give 22 in 82% yield.7 The intramolecular cyclization of 22 was carried out in K2CO3/DMF condition to afford 13,8 which was purified by heating and stirring in 50% EtOH/EtOAc to give the compound with 73% overall yield and 98.6% purity (HPLC). During the synthetic process research of N-(4-chloro-3-cyano-7-ethoxyquinolin-6- yl)acetamide as the key intermediate of neratinib,9 we developed the similar method of this intramolecular cyclization. 4-Chloro-3-cyanoquinoline 23 was obtained by heating 13 in POCl3 with 83% isolated yield, which was condensed with commercially available 2,4-dichloro-5-methoxyaniline (24), catalyzed by pyridine hydrochloride to give compound 25 with 77% isolated yield. At the last step, 25 reacted with N-methylpiperazine, catalyzed by KI at 80 ºC to give the final product bosutinib (1), which was purified by recrystallization in MeOH/EtOAc with 76% overall yield and 98.9% purity (HPLC).
In summary, we have developed a new and practical synthetic route of bosutinib on a decagram scale. Adopting the easily commercially available methyl vanillate (14) as the starting material, through the simple and traditional steps including alkylation, bromination, ester hydrolysis, decarboxylation, and intramolecular cyclization to give the key 3-cyano-4-hydroxyquinoline intermediate 13. After another three steps including chlorination and condensation to give the final product 1 in 13.7% yield over ten steps and 98.9% purity (HPLC). Purification methods of the intermediates involved in the route were also given.
ACKNOWLEDGMENT
This work was supported by the Special Scientific Foundation for Outstanding Young Teachers in Shanghai Higher Education Institutions (No. ZZGJD13017), and the Undergraduate Innovative Training Project of Shanghai (cs1404011, cs1404013).
EXPERIMENTAL
All commercially available chemicals and solvents were used as received without any further purification. 1H NMR and 13C NMR spectra were recorded on a Bruker UltraShield 400 Plus spectrometer using TMS as an internal standard. Mass spectra were obtained from a Finnigan MAT-95/711 spectrometer. The HPLC results were generated using a Waters 2487 UV/Visible Detector and Waters 515 Binary HPLC Pump.
Methyl 4-(3-chloropropoxy)-3-methoxybenzoate (15). Methyl vanillate 14 (360 g, 1.98 mol), 1-bromo-3-chloropropane (404 g, 2.57 mol) and potassium carbonate (415 g, 3.0 mol) were mixed and stirred in DMF (1.8 kg) at 60 ºC for 2 h. The reaction suspension was cooled to room temperature and poured slowly into water (8 kg) while stirring constantly. The solid formed was filtered off and washed with water (0.8 kg × 2), dried at 60 ºC for 4 h. The white product was stirred and heated with 2:1 hexane/EtOAc (1 kg) at 60 ºC for 2 h then cooled to room temperature, the resulting solid was filtered off and washed with 2:1 hexane/EtOAc (300 g × 2), dried at 50 ºC for 4 h to afford 15 (466 g, 91%) as a white solid. 1H NMR (400 MHz, CDCl3): δ 2.32 (m, 2H), 3.78 (t, J = 6.4 Hz, 2H), 3.90 (s, 3H), 3.91 (s, 3H), 4.23 (t, J = 6.0 Hz, 2H), 6.91 (d, J = 8.4 Hz, 1H), 7.55 (d, J = 2.0 Hz, 1H), 7.67 (dd, J = 2.0, 8.4 Hz, 1H). ESI-MS (m/z) 281.0 [M+Na]+.
Methyl 2-bromo-4-(3-chloropropoxy)-5-methoxybenzoate (16). A stirred suspension of 15 (259 g, 1.0 mol), Br2 (183 g, 1.15 mol) and AcOH (1.5 kg) was stirred at 40–50 ºC for 6 h to form a white solution. The mixture was poured slowly into water (5 kg) over 20 min and stirred. The resulting white solid was filtered, washed with H2O (300 g × 3) and dried at 60 ºC for 5 h to afford 16 (301 g, 89%) as a white solid. 1H NMR (400 MHz, DMSO-d6): δ 2.19 (m, 2H), 3.77 (t, J = 6.4 Hz, 2H), 3.81 (s, 3H), 3.83 (s, 3H), 4.18 (t, J = 6.4 Hz, 2H), 7.28 (s, 1H), 7.38 (s, 1H). 13C NMR (100 MHz, DMSO-d6): δ 32.0, 42.2, 52.7, 56.3, 66.2, 112.9, 114.4, 118.3, 123.7, 148.3, 151.4, 165.8. ESI-MS (m/z) 338.9 [M+H]+, 360.9 [M+Na]+.
2-Bromo-4-(3-chloropropoxy)-5-methoxybenzoic acid (17). A mixture of 16 (260 g, 0.77 mol), NaOH (43 g, 1.08 mol) in MeOH (1.5 kg) and H2O (1.5 kg) was stirred and heated at 50–60 ºC for 12 h to form a clear solution. Until it was cooled to room temperature, H2SO4 was added slowly into the reaction solution to acidify to pH 2–3. The white suspension was stirred at the ambient temperature for 1 h. The resulting solid was filtered off and washed with H2O (0.4 kg × 2), dried at 50 ºC for 5 h to give 17 (232 g, 93%) as a white solid. 1H NMR (400 MHz, DMSO-d6): δ 2.17 (m, 2H), 3.77 (t, J = 6.0 Hz, 2H), 3.81 (s, 3H), 4.17 (t, J = 6.0 Hz, 2H), 7.26 (s, 1H), 7.39 (s, 1H). 13C NMR (100 MHz, DMSO-d6): δ 32.0, 42.1, 56.3, 66.1, 112.7, 114.6, 118.3, 124.8, 148.3, 151.0, 166.9. ESI-MS (m/z) 346.9 [M+Na]+.
Ethyl 3-(2-bromo-4-(3-chloropropoxy)-5-methoxyphenyl)-2-cyano-3-hydroxyacrylate (19). A mixture of 17 (194 g, 0.6 mol) and SOCl2 (2 kg) was stirred and heated to reflux for 4 h to form a homogeneous solution. The solvent was recovered to give 2-bromo-4-(3-chloropropoxy)-5- methoxybenzoyl chloride (18) as a faint yellow solid.
A suspension of NaOEt (95 g, 1.4 mol) in anhydrous EtOH ( 0.8 kg) was stirred at 40–50 ºC for 1 h to get a solution firstly, and CH2(CN)CO2Et (170 g, 1.5 mol) was added. The resulting white suspension was heated to reflux for another 0.5 h and then cooled to –5 ºC in an ice-salt bath and treated dropwise with the above 18 (0.6 mol) in THF (0.8 kg) over 2 h, keeping the reaction temperature below 0 ºC. The reaction mixture was then added to water (5 kg), stirred and acidified to pH 2–3 with H2SO4. The resulting solid was collected by suction filtration, washed with H2O (0.3 kg × 3), and dried at 50 ºC for 5 h to give crude 19, which was recrystallized from 3:1 hexane/EtOAc (0.7 kg) to afford 19 (193 g, 77%) as an off-white solid. 1H NMR (400 MHz, CDCl3): δ 1.44 (t, J = 7.2 Hz, 3H), 2.33 (m, 2H), 3.78 (t, J = 6.0 Hz, 2H), 3.80 (s, 3H), 4.22 (t, J = 6.0 Hz, 2H), 4.44 (q, J = 7.2 Hz, 2H), 6.99 (s, 1H), 7.16 (s, 1H), 13.92 (s, 1H). 13C NMR (100 MHz, DMSO-d6): δ 14.3, 24.9, 32.0, 42.2, 56.6, 60.4, 62.4, 66.2, 83.5, 110.8, 113.0, 116.9, 129.4, 148.8, 163.0, 181.1. ESI-MS (m/z) 419.0 [M+H]+.
3-(2-Bromo-4-(3-chloropropoxy)-5-methoxyphenyl)-3-oxopropanenitrile (20). A mixture of 19 (180 g, 0.43 mol), DMSO (0.9 kg) and H2O (50 g) was heated at 100–110 ºC for 30 min. Then the brown solution was cooled to around 50 ºC, poured into chilled water (2 kg), and stirred for 1 h. The resulting precipitate was collected by suction filtration, washed with H2O (100 g × 3) and 50% EtOH/H2O (100 g × 1), dried at 60 ºC for 4 h to give a brown solid, which was recrystallized from 1:1 hexane/EtOAc (0.8 kg) to afford 20 (121 g, 81%) as faint yellow solid. 1H NMR (400 MHz, CDCl3): δ 2.34 (m, 2H), 3.78 (t, J = 6.0 Hz, 2H), 3.90 (s, 3H), 4.22 (s, 2H), 4.24 (t, J = 6.0 Hz, 2H), 7.12 (s, 1H), 7.22 (s, 1H), 13C NMR (100 MHz, DMSO-d6): δ 31.9, 32.7, 42.2, 56.6, 66.2, 111.5, 113.9, 117.3, 128.9, 148.6, 151.7, 170.9, 190.0. ESI-MS (m/z) 369.9 [M+Na]+.
3-Amino-2-(2-bromo-4-(3-chloropropoxy)-5-methoxybenzoyl)acrylonitrile (22). A mixture of 20 (104 g, 0.3 mol), CH(OMe)3 (64 g, 0.6 mol) and Ac2O (500 g) was heated at 120 ºC for 2 h. The solvent was removed to give the 2-(2-bromo-4-(3-chloropropoxy)-5-methoxybenzoyl)-3-methoxyacrylonitrile 21 as a brown oil, which was used at the next step without purification.
The above 21 (0.3 mol) was dissolved in MeOH (0.7 kg) and cooled to ~10 ºC. NH3 was then babbled to the solution till to saturated, and the reaction solution was stirred at 20 ºC for another 2 h. The resulting solid was collected by suction filtration, washed with 50% MeOH/H2O, and dried under reduced pressure to give 22 (92 g, 82%) as a pale solid. 1H NMR (400 MHz, DMSO-d6): δ 2.18 (m, 2H), 3.78 (s, 3H), 3.80 (t, J = 6.4 Hz, 2H), 4.14 (t, J = 6.4 Hz, 2H), 6.95 (s, 1H), 7.21 (s, 1H), 8.81 (br s, 2H). 13C NMR (100 MHz, DMSO-d6): δ 32.1, 42.3, 56.4, 66.1, 89.2, 109.2, 112.3, 115.8, 117.1, 130.8, 148.8, 149.4, 160.9, 186.4. ESI-MS (m/z) 375.0 [M+H]+.
7-(3-Chloropropoxy)-4-hydroxy-6-methoxyquinoline-3-carbonitrile (13). 22 (37.4 g, 0.1 mol) and K2CO3 (20.7 g, 0.15 mol) were suspended in DMF (180 g) under nitrogen. The reaction mixture was stirred and heated to 120 ºC for 3 h. Around 80 g of DMF was removed under vacuum and the residue was poured into chilled water (400 g), the resulting mixture was stirred at rt for 1 h. The resulting solid was filtered, washed with water, and dried to give the crude product 13 (26 g), which was suspended in 50% EtOH/EtOAc (80 g), stirred and heated to 70 ºC for 1 h. After cooled to rt, the resulting solid was collected by suction filtration, washed by 50% EtOH/EtOAc (15 g × 2), dried at 50 ºC to give the pure product 13 (21.3 g, 73%) as an off-white solid. 1H NMR (400 MHz, DMSO-d6): δ 2.27 (m, 2H), 3.82 (t, 2H), 3.88 (s, 3H), 4.19 (t, 2H), 7.07 (s, 1H), 7.45 (s, 1H), 8.58 (s, 1H), 12.50 (br s, 1H). 13C NMR (100 MHz, DMSO-d6): δ 31.8, 42.2, 56.3, 65.9, 93.2, 101.5, 104.9, 117.6, 119.7, 135.2, 145.3, 148.5, 153.2, 173.8. ESI-MS (m/z) 315.1 [M+Na]+. HPLC Conditions: Column: Acclaim C18 (150 mm × 2.1 mm × 5 µm); Detection: 280 nm; Flow rate: 0.8 mL/min; Temperature: rt; Injection load: 2 μL; Solvent: MeCN; Run time: 5 min; Mobile phase: MeCN /water = 80/20, tR: 0.490 min, purity: 98.6%.
4-Chloro-7-(3-chloropropoxy)-6-methoxyquinoline-3-carbonitrile (23). A suspension of compound 13 (20 g, 0.068 mol) and POCl3 (80 g, 0.52 mol) was stirred and heated to reflux for 2 h to give a homo- sulution. Around 40 g of POCl3 was removed under vacuum and the residue was poured into ice-water (200 g), the resulting mixture was stirred at rt for 1 h. The resulting solid was filtered, washed with water (30 g × 2), and dried at 50 ºC to give product 1 (17.6 g, 83%) as a faint yellow solid. 1H NMR (400 MHz, DMSO-d6): δ 2.29 (m, 2H), 3.83 (t, J = 6.0 Hz, 2H), 3.98 (s, 3H), 4.33 (t, J = 6.0 Hz, 2H), 7.29 (s, 1H), 7.46 (s, 1H), 8.90 (s, 1H). 13C NMR (100 MHz, DMSO-d6): δ 31.8, 42.2, 56.6, 66.3, 102.1, 104.9, 109.4, 116.0, 120.3, 143.1, 146.9, 148.7, 152.1, 154.7. ESI-MS (m/z) 311.0 [M–H] –.
7-(3-Chloropropoxy)-4-((2,4-dichloro-5-methoxyphenyl)amino)-6-methoxyquinoline-3-carbonitrile (25). A mixture of 23 (15 g, 0.048 mol), 2,4-dichloro-5-methoxyaniline 24 (9.6 g, 0.05 mmol) and pyridine hydrochloride (5.8 g, 0.05 mmol) in 2-methoxyethanol (80 g) was heated to 120 ºC for 3 h. The reaction suspension was cooled to room temperature and poured into water (300 g) while stirring. The resulting solid was filtered, washed with water (20 g × 2), and dried at 50 ºC to give product 25 (17.3 g, 77%) as a off-white solid. 1H NMR (400 MHz, DMSO-d6): δ 2.28 (m, 2H), 3.82 (t, J = 6.0 Hz, 2H), 3.87 (s, 3H), 3.97 (s, 3H), 4.30 (t, J = 6.0 Hz, 2H), 7.35 (s, 1H), 7.37 (s, 1H), 7.75 (s, 1H), 7.85 (s, 1H), 8.43 (s, 1H), 9.63 (s, 1H). 13C NMR (100 MHz, DMSO-d6): δ 31.9, 42.1, 56.7, 57.3, 65.8, 86.9, 102.4, 110.0, 113.1, 114.0, 117.4, 120.9, 123.6, 130.3, 136.7, 146.2, 149.8, 149.9, 151.3, 152.9, 154.5. ESI-MS (m/z) 468.0 [M+H]+.
Bosutinib (1). A mixture of 25 (12 g, 0.026 mmol) and KI (5.0 g, 0.03 mmol) in N-methylpiperazine (50 mL) was heated at 80 ºC for 12 h. Around 30 g of N-methylpiperazine was removed was removed under vacuum, the residue was poured into water (100 g) and stirred at rt for 1 h. The resulting solid was filtered, washed with water (20 g × 2), and dried at 50 ºC to give the crude product 1, which was purified by recrystallization in 20% MeOH/EtOAc with 76% overall yield (10.5 g). 1H NMR (400 MHz, DMSO-d6): δ 1.95 (m, 2H), 2.15 (s, 3H), 2.33–2.39 (m, 8H), 2.43 (t, J = 6.4 Hz, 2H), 3.86 (s, 3H), 3.94 (s, 3H), 4.19 (t, J = 6.4 Hz, 2H), 7.29 (m, 2H), 7.72 (s, 1H), 7.82 (s, 1H), 8.39 (s, 1H). 13C NMR (100 MHz, DMSO-d6): δ 26.5, 46.2, 53.2, 54.8, 55.3, 56.6, 57.2, 61.0, 67.3, 86.6, 102.5, 109.5, 113.6, 117.5, 123.1, 130.2, 137.8, 146.1, 148.2, 149.7, 150.0, 151.1, 153.2, 154.4. ESI-MS (m/z) 530.0 [M–H] –. HPLC Conditions: Column: Agilent Eclipse XDB-C18 (250 mm × 4.6 mm × 5 µm); Detection: 254 nm; Flow rate: 0.8 mL/min; Temperature: rt; Injection load: 2 μL; Solvent: MeCN; Run time: 15 min; Mobile phase: MeCN /water = 60/40, tR: 2.090 min, purity: 98.9%.
References
1. M. Puttini, A. M. Coluccia, F. Boschelli, L. Cleris, E. Marchesi, A. Donella-Deana, S. Ahmed, S. Redaelli, R. Piazza, V. Magistroni, F. Andreoni, L. Scapozza, F. Formelli, and C. Gambacorti- Passerini, Cancer Res., 2006, 66, 11314; CrossRef A. Vultur, R. Buettner, C. Kowolik, W. Liang, D. Smith, F. Boschelli, and R. Jove, Mol. Cancer Ther., 2008, 7, 1185. CrossRef
2. J. E. Cortes, H. M. Kantarjian, T. H. Brummendorf, D. W. Kim, A. G. Turkina, Z. X. Shen, R. Pasquini, H. J. Khoury, S. Arkin, A. Volkert, N. Besson, R. Abbas, J. Wang, E. Leip, and C. Gambacorti-Passerini, Blood, 2013, 118, 4567; CrossRef J. E. Cortes, D. W. Kim, H. M. Kantarjian, T. H. Brummendorf, I. Dyagil, L. Griskevicus, H. Malhotra, C. Powell, K. Gogat, A. M. Countouriotis, and C. Gambacorti-Passerini, J. Clin. Oncol., 2012, 30, 3486; CrossRef A. I. Daud, S. S. Krishnamurthi, M. N. Saleh, B. J. Gitlitz, M. J. Borad, P. J. Gold, E. G. Chiorean, G. M. Springett, R. Abbas, S. Agarwal, N. Bardy-Bouxin, P. H. Hsyu, E. Leip, K. Turnbull, C. Zacharchuk, and W. A. Messersmith, Clin. Cancer Res., 2012, 18, 1092. CrossRef
3. D. Berger, D. Boschelli, S. Johnson, and Y. Wang, U.S. Patent 20030212276 A1, Nov. 13, 2003; K. T. Arndt, D. H. Boschelli, F. C. Boschelli, and M. M. Zaleska, PCT Int. Appl. WO 2004075898 A1, Sep. 10, 2004; D. H. Boschelli, Y. D. Wang, S. Johnson, B. Wu, F. Ye, A. C. Barrios Sosa, J. M. Golas, and F. Boschelli, J. Med. Chem., 2004, 47, 1599. CrossRef
4. G. J. Withbroe, C. Seadeek, K. P. Girard, S. M. Guinness, B. C. Vanderplas, and R. Vaidyanathan, Org. Process Res. Dev., 2013, 17, 500; CrossRef J. D. Olszewski, M. K. May, and D. M. Berger, U.S. Patent 20070208164 A1, Sep. 6, 2007; K. W. Sutherland, G. B. Feigelson, D. H. Boschelli, D. M. Blum, and H. L. Strong, U.S. Patent 2005043537 A1, Feb. 24, 2005.
5. X. Yin, G. Xu, X. Sun, Y. Peng, X. Ji, K. Jiang, and F. Li, Molecules, 2010, 15, 4261; CrossRef F. Li, X. Yin, K. Jiang, X. Sun, and G. Xu, China Patent CN 101792416 A, Aug. 4, 2010.
6. Y. Mao, J. Qin, H. He, G. Liu, B. Gao, and H. Wang, Heterocycles, 2014, 89, 1885. CrossRef
7. D. M. Barnes, A. C. Christesen, K. M. Engstrom, A. R. Haight, M. C. Hsu, E. C. Lee, M. J. Peterson, D. J. Plata, P. S. Raje, E. J. Stoner, J. S. Tedrow, and S. Wagaw, Org. Process Res. Dev., 2006, 10, 803; CrossRef Q. Wang, E. Lucien, A. Hashimoto, G. C. G. Pais, D. M. Nelson, Y. Song, J. A. Thanassi, C. W. Marlor, C. L. Thoma, J. Cheng, S. D. Podos, Y. Ou, M. Deshpande, M. J. Pucci, D. D. Buechter, B. J. Bradbury, and J. A. Wiles, J. Med. Chem., 2007, 50, 199; CrossRef Y. Asahina, K. Iwase, F. Iinuma, M. Hosaka, and T. Ishizaki, J. Med. Chem., 2005, 48, 3194. CrossRef
8. K. Grohe and H. Heitzer, Liebigs Ann. Chem., 1987, 29. CrossRef
9. Y. Mao, Y. He, F. Zhu, W. Chen, J. Shen, and J. Li, Heterocycles, 2014, 89, 1203. CrossRef