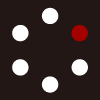
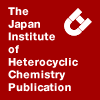
HETEROCYCLES
An International Journal for Reviews and Communications in Heterocyclic ChemistryWeb Edition ISSN: 1881-0942
Published online by The Japan Institute of Heterocyclic Chemistry
e-Journal
Full Text HTML
Received, 1st December, 2014, Accepted, 9th January, 2015, Published online, 13th January, 2015.
DOI: 10.3987/COM-14-13141
■ Selective Synthesis of 2,3-Dihydrofuran or Cyclopropane Derivatives via Tandem Reaction of β,γ-Unsaturated α-Ketoesters with Halides
Dong Cheng,* Xiangzhen Meng, Guoxu Qin, and Yimin Hu*
School of Chemical Engineering and Life Science, Chaohu College, Chaohu Anhui, Peoples 238000, China
Abstract
2,3-Dihydrofuran or cyclopropane derivatives were prepared with high selectivity via reaction of β,γ-unsaturated α-ketoesters with α-phenacyl bromide in different conditions.Dihydrofuran derivatives which are commonly found in the molecular skeleton of natural products and bioactive substances have been studied for many years.1-3 Cyclopropane derivatives are also important in the field of organic synthesis.4,5 A number of methods for the synthesis of them have been reported in the literature.6-9 Among them, stereoselective synthesis of 2,3-dihydrofuran or cyclopropane derivatives via the reaction of ylides with different α, β-unsaturated compounds have been reported.10-14 However, there is no report on selective synthesis of 2,3-dihydrofuran or cyclopropane derivatives via the same starting materials under different conditions.
As a sort of multi-functionalized building blocks, β,γ-unsaturated α-ketoester has recently been found useful utility in organic synthesis.15,16 In this work, we report the selective synthesis of 2,3-dihydrofuran or cyclopropane derivatives via reaction of β,γ-unsaturated α-ketoesters with α-phenacyl bromide in different conditions.
We initiated our study by treatment of 2-bromo-1-phenylethanone (1a), (E)-methyl 2-oxo-4-phenylbut-3-enoate (2a) with K2CO3 in CH2Cl2 at room temperature for 48 h (Scheme 1), the reaction afforded 2,3-dihydrofuran derivative (3a) in 76% yield and a single cyclopropane derivative isomer in 15% yield (4a).
Further, we carried out this reaction in different conditions (Table 1). It was found that when NaOH or Et3N was employed as base, (E)-methyl 2-oxo-4-phenylbut-3-enoate (2a) was full consumed but neither traces of 3a nor 4a was formed (entries 2 and 3). Completely no reaction occurred between 1a and 2a in the presence of DABCO in CH2Cl2 (entry 4). When the reaction was treated in the presence of DBU (3 eq) at room temperature, 3a was obtained in 88% and only a few trace of cyclopropanes was detected (entry 5). When the reaction was quenched at 1.5 h, the reaction become complex and the yield of 2,3-dihydrofuran derivative reduced to 24% (entry 6). The combination of DABCO with K2CO3 as base did not obtain 3a, the major product was 4a (entry 7). CH2Cl2 was found to be the best solvent of choice for the synthesis of 3a, other solvents such as THF, toluene, MeCN and DMF gave 3a in a moderate yield (entries 8-11).
Having established optimal reaction conditions for the synthesis of 3a, we investigated the scope and limitation of substrates by employing various β,γ-unsaturated α-ketoesters and halides (Table 2). It was observed that aromatic substrates bearing an electron-donating groups (entries 2 and 4), and electron-withdrawing groups (entries 3 and 5) all gave the corresponding 2,3-dihydrofuran derivatives. However, (E)-methyl 4-(furan-2-yl)-2-oxobut-3-enoate was not suitable for such transformation and no desired product was observed (entry 6). The ester groups in R2 have less effect on the yield (entry 7). 1-Bromopropan-2-one could also react with (E)-methyl 2-oxo-4-phenylbut-3-enoate to afford corresponding product (entry 8).
Further, we synthesized various cyclopropane derivatives by using various β,γ-unsaturated α-ketoesters and halides with the combination of DABCO and K2CO3 as base in CH2Cl2 at room temperature (Table 3). To our delight, smooth reactions were observed for all substrate and delivered the final products in moderate yields (entries 1-6). However, the reaction of 1-bromopropan-2-one with (E)-methyl 2-oxo-4-phenylbut-3-enoate did not give the desired product (entry 7).
A plausible reaction mechanism is proposed in Scheme 2. First, the quaternary ammoonium salt I was produced from the addition of α-phenacyl bromide to the tertiary amine. Deprotonation of I with base forms the ylide II, which undergoes Michael addition with β,γ-unsaturated α-ketoester to afford the intermediate III. The intermediate III under ring-closing reaction by internal SN2 reactions forms cyclopropane derivatives, whereas tautomerization of the intermediate III leads to generation of IV, followed by intramolecular cyclization of IV to form 2,3-dihydrofurans.
In summary, we have developed a convenient and efficient method for the synthesis of 2,3-dihydrofuran and cyclopropane derivatives. The reaction takes place via [4+1] or [2+1]-cycloaddition of β,γ-unsaturated α-ketoesters with α-phenacyl bromide. We believe that this method would give a new viable entry to highly functionalized dihydrofurans and cyclopropanes.
EXPERIMENTAL
Column chromatography was carried out on silica gel. The 1H and 13C NMR spectra were recorded at 300 and 75 MHz, respectively. All reagents were used directly as obtained commercially unless otherwise noted.
General procedure for the synthesis of 2,3-dihydrofuran derivatives
DBU (3.0 mmol) was added to a solution of 2-bromo-1-phenylethanone 1a (1.5 mmol) and (E)-methyl 2-oxo-4-phenylbut-3-enoate 2a (1.0 mmol) in CH2Cl2 (3 mL) at room temperature. The reaction was then stirred and followed by TLC. Upon full consumption of 2-oxo-4-phenylbut-3-enoate 2a. The solvent was evaporated under reduced pressure. The residue was purified by column chromatography on silica (EtOAc/petroleum ether = 1:6-1:4) to give 2,3-dihydrofuran derivatives.
General procedure for the synthesis of cyclopropane derivatives
K2CO3 (3.0 mmol) was added to a solution of 2-bromo-1-phenylethanone 1a (1.5 mmol), (E)-methyl 2-oxo-4-phenylbut-3-enoate 2a (1.0 mmol) and DABCO (1.5 mmol) in CH2Cl2 (3.0 mL) at room temperature. The reaction was then stirred and followed by TLC. Upon full consumption of 2-oxo-4-phenylbut-3-enoate 2a. The reaction was quenched with 5 mL of water and extracted with CH2Cl2 (3×5 mL). The combined organic extracts were washed with water and brine, and dried (MgSO4). After evaporating the solvent under reduced pressure, the residue was purified on silica gel (gelusing EtOAc/petroleum ether = 1:6-1:4) to give cyclopropane derivatives.
Methyl 5-benzoyl-4-phenyl-4,5-dihydrofuran-2-carboxylate (3a)
1H NMR (300 MHz, CDCl3): δ 8.03 (d, J = 6.9, 2H), 7.63–7.26 (m, 8H), 7.26 (d, J = 16.2, 1H), 6.66 (d, J = 16.2, 1H), 4.21 (s, 1H), 3.68 (s, 3H). 13C NMR (75 MHz, CDCl3): δ 190.9, 166.8, 135.2, 135.1, 134.2, 133.8, 128.9, 128.8, 128.5, 126.9, 120.9, 65.7, 63.1, 52.9.
1H NMR (300 MHz, CDCl3): δ 7.91 (d, J = 7.2, 2H), 7.62–7.57 (m, 3H), 7.49–7.44 (m, 5H), 6.80 (d, J = 16.2, 1H), 6.40 (d, J = 16.2, 1H), 4.63 (s, 1H), 3.92 (s, 3H). 13C NMR (75 MHz, CDCl3): δ 190.2, 168.3, 135.7, 135.3, 135.2, 134.2, 129.0, 128.5128.5, 128.3, 127.0, 115.8, 64.8, 61.5, 53.5.
HRMS EI (m/z): calcd for C19H16O4, 308.1049; found, 308.1047.
Methyl 5-(4-methylbenzoyl)-4-phenyl-4,5-dihydrofuran-2-carboxylate (3b)
1H NMR (300 MHz, CDCl3): δ 7.82 (d, J = 6.9, 2H), 7.28–7.20 (m, 7H), 6.80 (d, J = 15.9, 1H), 6.39 (d, J = 15.9, 1H), 4.61 (s, 1H), 3.92 (s, 3H), 2.39 (s, 3H). 13C NMR (75 MHz, CDCl3): δ 189.6, 168.4, 145.4, 135.6, 135.4, 132.7, 129.6, 128.7, 128.5, 128.4, 126.9, 116.0, 64.9, 61.4, 53.4, 21.8.
1H NMR (300 MHz, CDCl3): δ 7.93–7.86 (m, 2H), 7.43–7.29 (m, 7H), 6.90 (d, J = 15.9, 1H), 6.66 (d, J = 15.9, 1H), 4.20 (s, 1H), 3.68 (s, 3H), 2.42 (s, 3H). 13C NMR (75 MHz, CDCl3): δ 190.4, 166.9, 145.4, 135.2, 133.7, 132.6, 129.6, 128.8, 128.7, 126.9, 121.0, 65.7, 63.1, 52.9, 21.9.
HRMS EI (m/z): calcd for C20H18O4, 322.1205; found, 322.1204.
Methyl 5-(4-chlorobenzoyl)-4-phenyl-4,5-dihydrofuran-2-carboxylate (3c)
1H NMR (300 MHz, CDCl3): δ 7.99–7.97 (d, J = 8.1, 2H), 7.50–7.43 (m, 4H), 7.38–7.25 (m, 3H), 6.89 (d, J = 16.2, 1H), 6.64 (d, J = 16.2, 1H), 4.15 (s, 1H), 3.68 (s, 3H). 13C NMR (75 MHz, CDCl3): δ 190.0, 166.7, 140.8, 135.1, 133.8, 133.4, 130.0, 129.3, 128.9, 128.8, 126.9, 120.6, 65.7, 63.1, 53.0.
1H NMR (300 MHz, CDCl3): δ 7.86 (d, J = 7.5, 2H), 7.46–7.43 (m, 2H), 7.34–7.25 (m, 5H), 6.76 (d, J = 15.9, 1H), 6.37 (d, J = 15.9, 1H), 4.58 (s, 1H), 3.92 (s, 3H). 13C NMR (75 MHz, CDCl3): δ 189.2, 166.1, 140.8, 135.7, 135.2, 133.5, 129.7, 129.3, 128.6, 126.9, 115.6, 64.7, 61.4, 53.5.
HRMS EI (m/z): calcd for C19H15ClO4, 342.0659; found, 342.0654.
Methyl 5-benzoyl-4-p-tolyl-4,5-dihydrofuran-2-carboxylate (3d)
1H NMR (300 MHz, CDCl3): δ 8.02 (d, J = 7.2, 2H), 7.63–7.61 (m, 1H), 7.53–7.48 (m, 2H), 7.35–7.33 (m, 2H), 7.17–7.15 (m, 2H), 6.88 (d, J = 16.2, 1H), 6.59 (d, J = 16.2, 1H), 4.21 (s, 1H), 3.68 (s, 3H), 2.35 (s, 3H). 13C NMR (75 MHz, CDCl3): δ 191.0, 166.9, 138.9, 135.1, 134.2, 133.8, 132.4, 129.5, 128.9, 128.5, 126.9, 119.8, 65.7, 63.3, 52.9, 21.3.
1H NMR (300 MHz, CDCl3): δ 7.96–7.79 (m, 2H), 7.60–7.47 (m, 3H), 7.15–7.04 (m, 4H), 6.76 (d, J = 15.6, 1H), 6.76 (d, J = 15.9, 1H), 4.16 (s, 1H), 3.93 (s, 3H), 2.29 (s, 3H). 13C NMR (75 MHz, CDCl3): δ 190.2, 168.4, 138.5, 135.6, 135.2, 134.2, 132.5, 129.2, 128.9, 128.3, 126.9, 114.7, 64.9, 61.5, 53.4, 21.2.
HRMS EI (m/z): calcd for C20H18O4, 322.1205; found, 322.1203.
Methyl 5-benzoyl-4-(4-chlorophenyl)-4,5-dihydrofuran-2-carboxylate (3e)
1H NMR (300 MHz, CDCl3): δ 8.01 (d, J = 7.2, 2H), 7.62–7.60 (m, 1H), 7.52–7.47 (m, 2H), 7.37–7.29 (m, 4H), 6.85 (d, J = 15.9, 1H), 6.64 (d, J = 15.9, 1H), 4.16 (s, 1H), 3.66 (s, 3H). 13C NMR (75 MHz, CDCl3): δ 190.8, 166.7, 135.0, 134.5, 134.3, 133.9, 132.5, 129.0, 128.9, 128.5, 128.2, 121.6, 65.7, 63.0, 53.0.
1H NMR (300 MHz, CDCl3): δ 7.89 (d, J = 7.5, 2H), 7.80–7.56 (m, 3H), 7.48–7.03 (m, 4H), 6.74 (d, J = 16.2, 1H), 6.38 (d, J = 15.9, 1H), 4.64 (s, 1H), 3.92 (s, 3H). 13C NMR (75 MHz, CDCl3): δ 190.1, 168.1, 135.1, 134.4, 134.3, 133.8, 129.0, 128.7, 128.3, 128.2, 128.2, 116.5, 64.8, 61.3, 53.5.
HRMS EI (m/z): calcd for C19H15ClO4, 342.0659; found, 342.0658.
Isopropyl 5-benzoyl-4-phenyl-4,5-dihydrofuran-2-carboxylate (3f)
1H NMR (300 MHz, CDCl3): δ 8.06–7.90 (m, 2H), 7.63–7.25 (m, 8H), 6.93–6.34 (m, 2H), 5.28–5.22 (m, 1H), 4.58–4.21 (m, 1H), 1.41–1.01 (m, 6H). 13C NMR (75 MHz, CDCl3): δ 190.6, 190.4, 167.3, 165.7, 135.5, 135.5, 135.3, 135.3,135.1, 134.2, 134.1, 133.7,128.9, 128.9, 128.8, 128.7, 128.6, 128.5, 128.4, 128.3, 126.9 121.3, 116.2, 70.7, 70.3, 66.0, 64.8, 62.8, 61.6, 21.7, 21.4, 21.3.
HRMS EI (m/z): calcd for C21H20O4, 336.1362; found, 336.1362.
Methyl 5-acetyl-4-phenyl-4,5-dihydrofuran-2-carboxylate (3g)
1H NMR (300 MHz, CDCl3): δ 7.34-7.23 (m, 5H), 6.88–6.82 (m, 1H), 6.46–6.41 (m, 1H), 3.94–3.55 (4H), 2.24–2.06 (3H). 13C NMR (75 MHz, CDCl3): δ 201.51, 200.58, 167.96, 166.85, 135.52, 135.13, 133.55, 128.72, 126.90, 126.87, 121.15, 116.44, 66.66, 65.31, 63.09, 60.91, 53.26, 53.01, 28.31, 27.57, 23.46, 22.67.
HRMS EI (m/z): calcd for C16H18O4, 274.1205; found, 274.1201.
Methyl 2-(2-benzoyl-3-phenylcyclopropyl)-2-oxoacetate (4a)
1H NMR (300 MHz, CDCl3): δ 7.96–7.93 (m, 2H), 7.57–7.23 (m, 7H), 3.87 (s, 3H), 3.46–3.41 (m, 2H), 3.33–3.30 (m, 1H). 13C NMR (75 MHz, CDCl3): δ 194.2, 188.7, 161.0, 137.4, 136.0, 133.8, 128.9, 128.8, 128.6, 127.6, 126.5, 53.2, 39.5, 34.8, 32.2.
HRMS EI (m/z): calcd for C19H16O4, 308.1049; found, 308.1046.
Methyl 2-(2-(4-methylbenzoyl)-3-phenylcyclopropyl)-2-oxoacetate (4b)
1H NMR (300 MHz, CDCl3): δ 7.87–7.84 (m, 2H), 7.35–7.24 (m, 7H), 3.87 (s, 3H), 3.45–3.42 (m, 2H), 3.30–3.27 (m, 1H), 2.39 (s, 3H). 13C NMR (75 MHz, CDCl3): δ 193.8, 188.8, 161.0, 144.8, 137.6, 133.6, 129.5, 128.9, 128.8, 127.5, 126.5, 53.2, 39.6, 34.7, 32.0, 21.8.
HRMS EI (m/z): calcd for C20H18O4, 322.1205; found, 322.1199.
Methyl 2-(2-(4-chlorobenzoyl)-3-phenylcyclopropyl)-2-oxoacetate (4c)
1H NMR (300 MHz, CDCl3): δ 7.90–7.87 (m, 2H), 7.43–7.23 (m, 7H), 3.88 (s, 3H), 3.42–3.33 (m, 3H). 13C NMR (75 MHz, CDCl3): δ 193.0, 188.5, 160.9, 140.3, 137.2, 134.4, 130.0, 129.1, 129.0, 127.7, 126.4, 53.3, 39.3, 34.8, 32.3.
HRMS EI (m/z): calcd for C19H15ClO4, 342.0659; found, 342.0657.
Methyl 2-(2-benzoyl-3-p-tolylcyclopropyl)-2-oxoacetate (4d)
1H NMR (300 MHz, CDCl3): δ 7.96–7.93 (m, 2H), 7.57–7.55 (m, 1H), 7.46–7.41 (m, 2H), 7.25–7.15 (m, 4H), 3.88 (s, 3H), 3.43–3.39 (m, 2H), 3.29–3.27 (m, 1H), 2.45 (s, 3H). 13C NMR (75 MHz, CDCl3): δ 194.3, 188.8, 161.0, 137.3, 136.1, 134.4, 133.8, 129.6, 128.8, 128.6, 126.4, 53.2, 39.8, 34.8, 32.1, 21.1.
HRMS EI (m/z): calcd for C20H18O4, 322.1205; found, 322.1203.
Methyl 2-(2-benzoyl-3-(4-chlorophenyl)cyclopropyl)-2-oxoacetate (4e)
1H NMR (300 MHz, CDCl3): δ 7.94–7.91 (m, 2H), 7.60–7.55 (m, 1H), 7.47–7.42 (m, 2H), 7.34–7.31 (m, 2H), 7.19–7.17 (m, 2H), 3.87 (s, 3H), 3.42–3.38 (m, 2H), 3.30–3.25 (m, 1H). 13C NMR (75 MHz, CDCl3): δ 193.9, 188.3, 160.9, 136.0, 135.9, 133.9, 133.3, 129.1, 128.8, 128.6, 127.9, 53.3, 39.3, 34.7, 31.4.
HRMS EI (m/z): calcd for C19H15ClO4, 342.0659; found, 342.0656.
Isopropyl 2-(2-benzoyl-3-phenylcyclopropyl)-2-oxoacetate (4f)
1H NMR (300 MHz, CDCl3): δ 7.98–7.96 (m, 2H), 7.57–7.25 (m, 8H), 5.16–5.12 (m, 1H), 3.45–3.42 (m, 2H), 3.33–3.28 (m, 1H), 1.33–1.31 (m, 6H). 13C NMR (75 MHz, CDCl3): δ 194.0, 189.1, 160.1, 137.6, 136.2, 133.7, 128.9, 128.7, 128.6, 128.3, 127.5, 126.5, 70.8, 39.0, 35.1, 32.1, 29.7, 21.6.
HRMS EI (m/z): calcd for C21H20O4, 336.1362; found, 336.1360.
ACKNOWLEDGEMENTS
Project was supported by the Natural Science Foundation of Anhui Higher Education Institution (no. KJ2013B166, KJ2013Z232) and Chaohu College Foundation for Doctor in China.
References
1. M. M. Faul and B. E. Huff, Chem. Rev., 2000, 100, 2407. CrossRef
2. A. Schoop, H. Greiving, and A. Gohrt, Tetrahedron Lett., 2000, 41, 1913. CrossRef
3. S. Schabbert and E. Schaumann, Eur. J. Org. Chem., 1998, 1873.
4. T. Tsuji and S. Nishida, 'The Chemistry of the Cyclopropyl Group,' ed. by S. Patai, Wiley and Sons Press, Inc., New York, 1987.
5. F. Gnad and O. Reiser, Chem. Rev., 2003, 103, 1603. CrossRef
6. J. W. Lee and Y. Dong, Heterocycles, 1990, 31, 1417. CrossRef
7. K. S. Feldman and M. L. Wrobleski, J. Org. Chem., 2000, 65, 8659. CrossRef
8. H. Lebel, J. F. Marcoux, C. Molinaro, and A. B. Charette, Chem. Rev., 2003, 103, 977. CrossRef
9. J. Krysiak, T. Kato, H. Gornizka, A. Baceiredo, M. Mikolajczyk, and G. Bertrand, J. Org. Chem., 2001, 66, 8240. CrossRef
10. W. Cao, H. Zhang, J. Chen, X. H. Zhou, M. Shao, and C. M. Mark, Tetrahedron, 2008, 64, 163. CrossRef
11. W. Cao, G. Chen, J. Chen, and R. Chen, Synth. Commun., 2005, 35, 527. CrossRef
12. Z. J. Yang, M. J. Fan, R. Z. Mu, W. M. Liu, and Y. M. Liang, Tetrahedron, 2005, 61, 9140. CrossRef
13. C. Feng, C. F. Lu, Z. X. Chen, N. G. Dong, J. W. Shi, and G. C. Yang, J. Heterocycl. Chem., 2010, 47, 671.
14. C. P. Chuang and A. I. Tsai, Synthesis, 2006, 675. CrossRef
15. M. Rueping, B. J. Nachtsheim, S. A. Moreth, and M. Bolte, Angew. Chem. Int. Ed., 2008, 47, 593. CrossRef
16. Q. G. Wang, X. M. Deng, B. H. Zhu, L.W. Ye, X. L. Sun, C. Y. Zhu, and Y. Tang, J. Am. Chem. Soc., 2008, 130, 5408. CrossRef