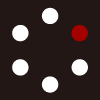
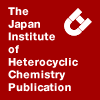
HETEROCYCLES
An International Journal for Reviews and Communications in Heterocyclic ChemistryWeb Edition ISSN: 1881-0942
Published online by The Japan Institute of Heterocyclic Chemistry
e-Journal
Full Text HTML
Received, 3rd December, 2014, Accepted, 17th December, 2014, Published online, 18th December, 2014.
DOI: 10.3987/COM-14-13143
■ Stereoselective Synthesis of the A-Ring of Armatol A from a Bromo-substituted Chiral Building Block Based on Ireland-Claisen Rearrangement and Ring-Closing Olefin Metathesis
Yuta Hirose, Kenshu Fujiwara,* Takafumi Saito, Ryo Katoono, and Takanori Suzuki
Division of Chemistry, Graduate School of Science, Hokkaido University, Kita 10 Nishi 8, Kita-ku, Sapporo, Hokkaido 060-0810, Japan
Abstract
The stereoselective synthesis of the A-ring of armatol A, a natural polycyclic ether triterpene from the red alga Chondria armata, was achieved in a non-biomimetic way. The synthesis employed Ireland-Claisen rearrangement of an ester, prepared from a bromo-substituted chiral building block, for the construction of C6 and C7 stereocenters and a relay ring-closing olefin metathesis for the seven-membered ring formation.INTRODUCTION
Armatol A (Figure 1) and five other congeners, armatols B–F, were isolated from the red alga Chondria armata by Ciavatta and co-workers.1 All the armatols have a solitary bromo-substituted oxepane (A-ring), a fused tricyclic ether moiety (BCD-ring), and a triterpene framework in common. The partial relative configurations of the A-ring and the BCD-ring of each armatol congener were determined by NMR analysis, and the partial absolute configuration of the A-ring of armatol A was confirmed via chemical degradation. However, the relative relationship between the A- and the BCD-rings and the configuration at C10 were unclear until Jamison and co-workers determined the full absolute configuration of armatol A, shown in Figure 1, by total synthesis in 2013.2
It is remarkable that the hydroxy group at the C6 tetrasubstituted carbon in the common A-ring of armatols A, B, D, and F is in a cis-relationship to the carbon chain at C7, because the cis-configuration is unusual among natural oxepanes possessing similar substituents.3 The bromo-substitution at the congested C3 position in the solitary oxepane (A-ring) is also an unprecedented feature of armatols. In addition to these structural characteristics of the A-ring, the structural complexity in the fused tricyclic ether system4 of the BCD ring of armatols prompted us to initiate a program toward their total synthesis.5,6 As part of this program, the synthesis of the A-ring of armatol A was studied.
The A-ring was synthesized by Jamison's group through a biomimetic bromonium-initiated epoxide-opening cascade with modification of McDonald's procedure (Scheme 1).2,7 An alternative biogenetic-like cyclization of a 6-methyl-hept-5-en-1-ol system initiated by N-bromosuccinimide was also reported by Morimoto for the construction of the related bromo-substituted oxepanes.8 In contrast, we have studied a non-biomimetic synthetic route to the A-ring in expectation of the successful establishment of the bromo-substituted C3-stereocenter, which was not necessarily effective in the biomimetic synthesis. We describe herein the stereoselective synthesis of the A-ring (1) of armatol A from a bromo-substituted chiral building block via a route including the Ireland-Claisen rearrangement9 of ester 8 and the relay ring-closing olefin metathesis10,11 of triene 10.
RESULTS AND DISCUSSION
Previously, we reported the synthesis of oxepane 16 having the same framework as the A-ring of armatol A through a process involving the Ireland-Claisen rearrangement of ester 11 via (Z)-ketene silyl acetal 12 to produce 13.12 Conversion of 13 to diene 14, cyclization of 14 by ring-closing olefin metathesis (RCM), and transformation of the resulting 15 produced 16, which has a hydroxy group at C3 as a possible footing for the installation of a bromo group (Scheme 2).5 However, all efforts for the final establishment of the bromo group at C3 were in vain.13 Therefore, we revised the synthetic route to the A-ring in order to circumvent the late stage installation of the C3 bromo group.
Our previous route also included several successful processes that should be retained in the revised route. In the synthesis of 16, the establishment of the C6 and C7 stereocenters and the formation of the oxepane skeleton were successfully achieved by Ireland-Claisen rearrangement and RCM, respectively. Accordingly, we applied these two key reactions in the revised route. The previous route also showed that the bromo group at C3 of 11, which was introduced in a non-stereoselective manner as a protecting group for the olefin group at C3 to be used for RCM, was not affected in the Ireland-Claisen rearrangement reaction. Therefore, we planned to install the bromo group at C3 stereoselectively at an earlier stage of the synthesis, and not to tamper with it later.
The revised retrosynthetic route to the A-ring of armatol A is shown in Scheme 3. The construction of the A-ring was planned for the final stage of the synthesis via C4-C5 double bond formation from triene 10 by relay RCM followed by hydrogenation of the double bond.14 We expected smooth ring closure at the sterically congested reactive sites (C4 and C5) by applying relay RCM, which was reported to be effective for preparing sterically hindered cyclic alkenes.6,11 The establishment of stereocenters C6 and C7 relied on the Ireland-Claisen rearrangement of ester 8, which would be transformed to 9 stereoselectively via (Z)-ketene silyl acetal 18.6,12 The resulting 9 would be converted to triene 10 by a conventional process. To prevent the elimination of the oxygen functional group at C4, the electron-withdrawing pivaloyl (Piv) group was selected to stabilize ester 8 by suppressing electron donation from O6 to the adjacent double bond. The synthesis of 8 was undertaken from allylic alcohol 19 having (4S,5E)-stereochemistry and chiral carboxylic acid 20 having a bromo group at C3 with (R)-configuration. Alcohol 19 would be synthesized according to the previous study6 with small modification. Carboxylic acid 20, a bromo-substituted chiral building block, would be prepared from a chiral alcohol available from L-malic acid.
Allylic alcohol 19 was synthesized from 5,6-O-isopropylidene-L-gulonic acid γ-lactone 21 (Scheme 4). Oxidative cleavage of 21 with NaIO4 produced aldehyde 22,15 which was reacted with 1-propynyllithium, derived from 1-bromoprop-1-ene, in the presence of MgBr2 to give alcohol 23. The alcohol was oxidized with Dess-Martin periodinane (DMPI) to afford acetylene ketone 24 in 51% yield from 21.16 The hetero-Michael reaction of 24 with pivalic acid was successfully catalyzed by 1,4-diazabicyclo[2.2.2]octane (DABCO)17 to furnish acyloxyvinyl ketone 25 with (E)-geometry in 72% yield. The diastereoselective reduction of 25, assisted by the neighboring 2,2-dimethyl-1,3-dioxolan-4-yl group,18 produced the desired alcohol 19 as a major component (19:epi-19 = 8:1, 96% combined yield) under Luche conditions,19 though the epimers were inseparable. For the isolation of 19, the mixture of 19 and epi-19 was reacted with 3,5-dinitrobenzoyl chloride to produce a mixture of 3,5-dinitrobenzoate (DNBz) esters, which were separated by HPLC to give pure DNBz ester 26 (88%). The DNBz group of 26 was selectively removed with K2CO3 in MeOH at –20 °C to afford 19 in pure form (92%).
The stereochemistry of 19 was determined by NMR analysis (Scheme 5). The (E)-geometry of the double bond of 19 was confirmed by the presence of an NOE correlation between H4 and protons of the methyl group at C6. The (S)-configuration of C4 was established by application of the modified Mosher's method to 19.20
The synthesis of bromo-substituted chiral building block 20 from alcohol 28, available from L-malic acid,21 is shown in Scheme 6. After alcohol 28 was oxidized under Swern conditions,22 the resulting aldehyde was reacted with MeMgBr followed by Ac2O to give acetate 29. The triethylsilyl (TES) ether of 29 was selectively removed using pyridinium p-toluenesulfonate (PPTS) to produce alcohol 30 in 39% overall yield from 28. Treatment of 30 with CBr4 and Bu3P in toluene at 70 °C afforded bromide 31 with inversion of stereochemistry in 84% yield without formation of byproducts caused by neighboring participation of the acetate group.23 Next, the acetate group was removed with diisobutylaluminum hydride (DIBALH) (94%), and the resulting alcohol 32 was oxidized with Dess-Martin periodinane (DMPI)16 to give ketone 33 (98%). The unstable ketone 33 was immediately reacted with 1,2-bis(trimethylsiloxy)ethane in the presence of trimethylsilyl trifluoromethanesulfonate (TMSOTf) under Noyori's conditions to furnish cyclic acetal 34 quantitatively.24 Methylation of 34 was successfully achieved by the reaction with Me3Al in toluene under reflux conditions (87%).25 The resulting alcohol 35 was then subjected to Parikh-Doering oxidation26 followed by Lindgren oxidation27 to produce carboxylic acid 36 (83% from 35). The optical purity of the compound was determined at this stage by HPLC analysis using a chiral column. The optical purity varied significantly according to the reaction conditions employed for the oxidation of alcohol 32. While Dess-Martin oxidation resulted in 100–82.4% ee, Swern oxidation showed at most 67% ee. The reaction scale of the Dess-Martin oxidation also affected the optical purity. The sub-gram scale oxidation gave a better result (~100% ee) than the gram scale oxidation (82.4% ee). The tert-butyldiphenylsilyl group (TBDPS) of 36 was converted to a triisopropylsilyl (TIPS) group through a process including methyl ester formation, removal of the TBDPS group, attachment of a TIPS group, and hydrolysis of the methyl ester to produce 20 in 56% yield from 36. Thus, bromo-substituted chiral building block 20 was obtained with good optical purity.
Next, ester 8 was synthesized from allylic alcohol 19 and carboxylic acid 20 by condensation with 1-(3-dimethylaminopropyl)-3-ethylcarbodiimide hydrochloride (EDCI•HCl) in the presence of 4-dimethylaminopyridine (DMAP) (89%) (Scheme 6). Thanks to the pivaloyl group, the stability of 8 was significantly improved over that of ester 11, which was found to be labile in the previous study.6 Ester 8 was stable under refrigeration for several months without decomposition.
The establishment of stereocenters C6 and C7 was accomplished with ester 8 by Ireland-Claisen rearrangement (Scheme 7). After intensive optimization of reaction conditions, ester 8 was reacted with potassium bis(trimethylsilyl)amide (KHMDS) in the presence of chlorotrimethylsilane (TMSCl) in toluene at –78 °C for 30 min. The reaction mixture was treated with diethyl malonate at –78 °C to scavenge unreacted KHMDS and then warmed to 0 °C to complete the rearrangement. The resulting carboxylic acid was esterified with TMSCHN228 to afford an 8:1 mixture of 9 and 7-epi-9 in 91% yield from 8. Interestingly, ester 8 showed reduced reactivity with lithium diisopropylamide (LDA) in toluene, which caused a low yield of products. Although the reactivity of 8 to LDA was increased in tetrahydrofuran (THF), the rearrangement proceeded with reversed stereoselectivity. Thus, the Ireland-Claisen rearrangement of 8 using KHMDS/TMSCl in toluene was found to produce the desired 9 selectively in good yield.
Next, the conversion of 9 to diol 39, including removal of the pivaloyl group and reduction of the methyl ester, was examined under reductive conditions (Scheme 7). The reduction of 9 with an excess amount of DIBALH in CH2Cl2 at –20 °C removed the pivaloyl group completely, but the methyl ester was only partly reduced to give aldehyde 38 (27%) and alcohol 39 (50%). Several attempts to improve the yield of 39 were unsuccessful; prolonged reaction time or warmed conditions only led to the reductive cleavage of the acetonide group. Therefore, the synthesis of 39 employed stepwise reduction via 38, i.e., treatment of 38 with NaBH4 in MeOH gave 39 in 86% yield, thereby furnishing 39 in 73% total yield from 9. Other stereoisomers were also removed at this stage.
The protection of the 1,3-diol moiety of 39 and the subsequent transformation of the 2,2-dimethyl-1,3-dioxolanyl group to a hydroxymethyl group were examined next (Scheme 8). A 4-chlorobenzylidene acetal was selected as a protecting group for the 1,3-diol moiety because of its expected resistance to the acidic conditions needed for the hydrolysis of the acetonide group. However, the protection of the 1,3-diol moiety with 4-chlorobenzaldehyde dimethyl acetal in the presence of 10-camphorsulfonic acid (CSA) was very slow, and therefore the removal of the TIPS group and the partial exchange of the acetonide group for a 4-chlorobenzylidene acetal group competed under these conditions. As a result, a mixture of acetal alcohols 40, 41a and 41b was obtained. Fortunately, selective hydrolysis of the five-membered cyclic acetal group of each compound was achieved with 0.5 mol/L aq. HCl in MeCN at 0 °C to give the same triol. The 1,2-diol moiety of the triol was then oxidatively cleaved to give aldehyde alcohol 42 in 27% total yield from 39. The protection of the alcohol of 42 as a TIPS ether and the subsequent Luche reduction19 of the aldehyde group gave allylic alcohol 43 in 94% yield over two steps.
The relative stereochemical relationship between C6 and C7, which were constructed by the Ireland-Claisen rearrangement of 8, was determined by NMR analysis of 41a, which was separated from the above mixture of alcohols 40, 41a and 41b by HPLC (Figure 2). The axial proton at C8 (H8ax) of the 1,3-dioxane ring, which has a large JH7-H8ax value (10.7 Hz) indicating the trans-diaxial relationship between H7ax and H8ax, shows an NOE correlation with the acetal proton of the 1,3-dioxane indicating the proximate 1,3-diaxial relationship between H8ax and the acetal proton. The axial acetal proton also shows NOE correlations with H4 and H5, which indicates that the alkenyl group at C6 is in an axial position and is proximate to the acetal proton. Accordingly, the trans-diaxial relationship between the alkenyl group and H7ax is suggested. This is also supported by the absence of an NOE correlation between H7ax and H5 or H4, which suggests that H7ax and the alkenyl group are remote from each other. Thus, the (6S*,7S*) relative configuration, which originated from 9, was determined by NMR analysis of 41a.
The absolute configuration of C7 was determined to be (S) by NMR analysis applying the modified Mosher's method20 to alcohol 44, which was prepared by the reductive degradation of 43 with Zn (Scheme 9). Accordingly, from the above relative stereochemical relationship between C6 and C7, the (6S,7S) absolute stereochemistry was confirmed.
Triene 10, a substrate for seven-membered ring formation by relay RCM at the final stage, was then synthesized from 43 (Scheme 10). After allylation of alcohol 43 under conventional conditions, the resulting 46 was desilylated with Bu4NF to give alcohol 47 (76% from 43). The application of Grieco-Nishizawa elimination conditions29 to alcohol 47 successfully produced allylic bromide 48 (87%), of which the 4-chlorobenzylidene acetal was hydrolyzed under acidic conditions to afford 10 (92%).
The completion of the synthesis of A-ring 1 is shown in Scheme 11. The successful cyclization of 10 by relay RCM with the second generation Hoveyda-Grubbs catalyst30 required heating at reflux in xylenes and produced 7-membered ring ether 49 in 35% yield along with some oligomeric byproducts. It is notable that the treatment of 48 with the second generation Grubbs31 or the second generation Hoveyda-Grubbs catalyst only promoted oligomerization, which rationalized the removal of the 1,3-dioxane moiety from 48 before performing relay RCM. The hydrogenation of the double bond of 49, which employed a diimide generated in situ from 2-nitrobenzenesulfonylhydrazide (NBSH), produced A-ring 1 (≤ 43% yield).32 The reduction was slow and required three days for completion even with an excess amount of NBSH, which was attributable to the steric congestion around the double bond.
The stereochemistry of the bromo-substituted C3 stereocenter, which originated from building block 20, was confirmed by NMR analysis. The presence of NOE correlations H3/H7, H3/H5b, H5b/H7, H3/2-CHb3, and H7/2-CHb3, suggests that H3, H5b, H7, and 2-CHb3 are in proximity to each other and are located on the same side of the oxepane ring with cis-relationships. On the other hand, H4a, which is suggested to be anti to both H3 and H5b by the large JH3-H4a (11.0 Hz) and JH4a-H5b (13.0 Hz), only shows an NOE enhancement with 2-CHa3 at 1.48 ppm. This suggests that H4a and 2-CHa3 are on the other side of the oxepane ring. Thus, the (3R) configuration was confirmed based on its relative relationship with the (7S) stereocenter, thereby completing the synthesis of the A-ring (1) of armatol A.
In conclusion, the stereoselective synthesis of the A-ring of armatol A, a natural polycyclic ether triterpene from the red alga Chondria armata, was achieved in a non-biomimetic way. The synthesis employed the Ireland-Claisen rearrangement of ester 8, prepared from bromo-substituted chiral building block 20, for the construction of C6 and C7 stereocenters and the relay ring-closing olefin metathesis of triene 10 for the seven-membered ring formation. Further studies toward the total synthesis of armatols are in progress in our laboratory.
EXPERIMENTAL
All air sensitive reactions were carried out under Ar atmosphere in oven-dried glassware using standard syringe, cannula and septa techniques. THF was prepared by Glass Contour Solvent Dispensing System (Nikko Hansen & Co., Ltd.). Other anhydrous solvents were purchased from commercial sources. All reactions were monitored by thin-layer chromatography (TLC) with precoated silica gel plates (Merck, silica gel 60 F254). Plates were visualized by ultraviolet light and by treatment with acidic anisaldehyde or phosphomolybdic acid stain followed by heating. Chromatographic purifications were performed on silica gel (YMC, Silica Gel 60, 63–210 µm) columns with indicated eluents. Optical rotations were recorded on a JASCO P-1020 digital polarimeter at 589 nm. IR spectra were recorded on a JEOL JIR-WINSPEC100 Fourier-transform infrared spectrometer in noted states. NMR spectra were recorded on a JEOL JNM-AL300 (1H at 300 MHz, 13C at 75 MHz) or a JEOL JNM-α-400 (1H at 400 MHz, 13C at 100 MHz) magnetic resonance spectrometer at ambient temperature. Chemical shifts (δ) are reported in ppm based on the resonance of tetramethylsilane (0 ppm for 1H NMR in CDCl3) or the respective solvent (1H NMR: 7.15 ppm in C6D6; 13C NMR: 77.0 ppm in CDCl3, 128.0 ppm in C6D6) as the internal standard. High resolution mass spectra (HRMS) were measured on a JEOL JMS-T100GCV (under field desorption [FD] or field ionization (FI) conditions) or a JEOL JMS-SX102A (under chemical ionization [CI] conditions) double focusing magnetic sector mass spectrometer or a Thermo Scientific Exactive Fourier transform ion cyclotron resonance mass spectrometer (under electrospray ionization (ESI) conditions).
(S)-1-(2,2-Dimethyl-1,3-dioxolan-4-yl)but-2-yn-1-one (24). To a solution of 21 (8.57 g, 39.3 mmol) in CH2Cl2 (100 mL) and H2O (10 mL) were added KHCO3 (9.83 g, 98.2 mmol) and NaIO4 (21.12 g, 98.74 mmol) at 23 ºC, and the mixture was stirred overnight. Then, anhydrous MgSO4 was added to the mixture to remove H2O. The mixture was filtered, and concentrated under reduced pressure to give crude aldehyde 22, which was immediately used in the next reaction without further purification. To a stirred suspension of magnesium (2.86 g, 118 mmol) in THF (65 mL) was added dropwise 1,2-dibromoethane (10.1 mL, 118 mmol) via a dropping funnel. The ethene evolving reaction was exothermic, and the reaction temperature was maintained below the boiling point of THF. The mixture was further stirred at ambient temperature for 1 h. After the resulting suspension of MgBr2 was cooled to –20 ºC, a solution of 1-propynyllithium, prepared by the reaction of 1-bromoprop-1-ene (6.4 mL, 75 mmol) with BuLi (1.62 mol/L in hexane, 92.0 mL, 149 mmol) in THF (65 mL) at –78 ºC for 20 min, was added to the suspension, and the mixture was stirred for 30 min at –20 ºC. To the mixture were added a solution of the above crude 22 in THF (65 mL) at –20 ºC via cannula, and the mixture was stirred at 0 ºC for 30 min. Then, the reaction was quenched with saturated aq. NH4Cl, and the mixture was extracted with EtOAc several times. The combined organic layers were washed with brine, dried over anhydrous MgSO4, filtered, and concentrated under reduced pressure. The residue was roughly purified by column chromatography (silica gel, hexane/EtOAc = 5) to give crude 23 (3.79 g), which was used immediately in the next reaction. To a solution of the above crude 23 in CH2Cl2 (110 mL) were added DMPI (18.9 g, 44.6 mmol) at 23 ºC, and the mixture was stirred for 3 h. Then, saturated aq. NaHCO3 (50 mL) and saturated aq. Na2SO3 (50 mL) were added, and the mixture was extracted with CH2Cl2 several times. The combined organic layers were washed with brine, dried over anhydrous MgSO4, filtered, and concentrated under reduced pressure. The residue was purified by column chromatography (silica gel, hexane/EtOAc = 8) to give 24 (3.36 g, 20.0 mmol, 51% over 3 steps); a colorless oil; [α]D26 –30 (c 0.72, CHCl3); IR (neat) ν 2989, 2938, 2890, 2217, 1673, 1385, 1372, 1258, 1216, 1150, 1103, 1067, 912, 845 cm–1; 1H NMR (300 MHz, CDCl3) δ 1.42 (3H, s), 1.52 (3H, s), 2.08 (3H, s), 4.16 (1H, dd, J = 5.0, 8.8 Hz), 4.24 (1H, dd, J = 7.4, 8.8 Hz), 4.53 (1H, dd, J = 5.0, 7.4 Hz); 13C NMR (75 MHz, CDCl3) δ 4.3 (CH3), 25.3 (CH3), 26.0 (CH3), 66.6 (CH2), 78.2 (C), 80.9 (CH), 95.2 (C), 111.6 (C), 186.5 (C); FI-HRMS (m/z) calcd for C9H12O3 [M+]: 168.0786, found: 168.0788.
(S,E)-4-(2,2-Dimethyl-1,3-dioxolan-4-yl)-4-oxobut-2-en-2-yl pivalate (25). To a solution of 24 (1.96 g, 11.7 mmol) in CH2Cl2 (120 mL) were added pivalic acid (2.0 mL, 18 mmol), DABCO (1.31 g, 11.7 mmol) at 25 ºC, and the mixture was stirred at 25 ºC for 14 h. Then, H2O was added, and the mixture was extracted with hexane several times. The combined organic layers were washed with brine, dried over anhydrous MgSO4, filtered, and concentrated under reduced pressure. The residue was purified by column chromatography (silica gel, hexane/EtOAc = 15) to give 25 (2.28 g, 8.43 mmol, 72%); a pale yellow oil; [α]D24 –52.0 (c 1.12, CHCl3); IR (neat) ν 2983, 2938, 2916, 2876, 1753, 1700, 1624, 1481, 1465, 1458, 1384, 1373, 1274, 1213, 1152, 1107, 1084, 1062, 1032, 902, 885, 843 cm–1; 1H NMR (300MHz, CDCl3) δ 1.27 (9H, s), 1.41 (3H, s), 1.47 (3H, s), 2.35 (3H, d, J = 0.8 Hz), 4.06 (1H, dd, J = 5.5, 8.5 Hz), 4.21 (1H, dd, J = 7.5, 8.5 Hz), 4.46 (1H, dd, J = 5.5, 7.5 Hz), 6.44 (1H, q, J = 0.8 Hz); 13C NMR (75 MHz, CDCl3) δ 18.7 (CH3), 25.0 (CH3), 25.8 (CH3), 26.6 (CH3 × 3), 39.0 (C), 66.4 (CH2), 80.3 (CH), 110.7 (C), 111.1 (CH), 165.7 (C), 175.3 (C), 198.5 (C); FD-HRMS calcd for C14H22O5 [M+]: 270.1467, found: 270.1430.
(S,E)-4-[(S)-2,2-Dimethyl-1,3-dioxolan-4-yl]-4-hydroxybut-2-en-2-yl pivalate (19) from 25. To a solution of 25 (2.28 g, 8.43 mmol) in MeOH (100 mL) were added CeCl3·7H2O (9.42 g, 25.3 mmol) and NaBH4 (957 mg, 25.3 mmol) at –78 ºC, and the mixture was stirred for 20 min. Then, the reaction was quenched with saturated aq. NH4Cl, and the mixture was extracted with EtOAc several times. The combined organic layers were washed with brine, dried over anhydrous MgSO4, filtered, and concentrated under reduced pressure. The residue was purified by column chromatography (silica gel, hexane/EtOAc = 5) to give an 8:1 mixture of 19 and its epimer at the allylic stereocenter (2.20 g, 8.08 mmol, 96% combined yield).
(S,E)-1-[(S)-2,2-Dimethyl-1,3-dioxolan-4-yl]-3-(pivaloyloxy)but-2-en-1-yl 3,5-dinitrobenzoate (26). After two batches of the reaction of a mixture of 19 and its epimer with 3,5-dinitrobenzoyl chloride were performed, the crude products of the two batches were combined and purified to give pure 26 as follows. To a solution of a mixture of 19 and its epimer (batch-1: 2.76 g, 10.1 mmol; batch-2: 1.29 g, 4.74 mmol) in CH2Cl2 (batch-1: 100 mL; batch-2: 40 mL) were added 3,5-dinitrobenzoyl chloride (batch-1: 3.50 g, 15.2 mmol; batch-2: 1.65 g, 7.16 mmol), Et3N (batch-1: 4.3 mL, 31 mmol; batch-2: 2.0 mL, 14 mmol) and DMAP (each batch: a catalytic amount) at 25 ºC, and the mixture was stirred at 25 ºC for 10 h. Then, the reaction was quenched with H2O, and the mixture was extracted with Et2O several times. The combined organic layers were washed with brine, dried over anhydrous MgSO4, filtered, and concentrated under reduced pressure. The residue was roughly purified by column chromatography (silica gel, hexane/EtOAc = 15) to give a mixture of 26 and its epimer at the allylic stereocenter. The diastereomeric mixtures from batches-1 and -2 were combined and purified by preparative HPLC (YMC-Pack SIL-06, 5 μm, 500 mm × 20 mm ID, 2% EtOH in hexane; flow rate: 20 mL/min, UV 254 nm detection) to give pure 26 (5.80 g, 13.0 mmol, 88% combined yield of batches-1 and -2); a colorless amorphous; [α]D24 +0.68 (c 0.925, CHCl3); IR (neat) ν 3101, 2982, 2917, 2881, 2849, 1738, 1629, 1547, 1481, 1461, 1383, 1371, 1345, 1275, 1227, 1167, 1143, 1109, 1072, 919, 842, 731, 721 cm–1; 1H NMR (300MHz, CDCl3) δ 1.24 (9H, s), 1.38 (3H, s), 1.50 (3H, s), 2.11 (3H, d, J = 1.1 Hz), 3.88 (1H, dd, J = 4.9, 9.2 Hz), 4.14 (1H, dd, J = 6.6, 9.2 Hz), 4.41 (1H, ddd, J = 4.9, 6.6, 7.5 Hz), 5.23 (1H, qd, J = 1.1, 10.4 Hz), 5.77 (1H, dd, J = 7.5, 10.3 Hz), 9.19 (2H, d, J = 2.1 Hz), 9.23 (1H, t, J = 2.1 Hz); 13C NMR (75 MHz, CDCl3) δ 16.3 (CH3), 25.1 (CH3), 26.6 (CH3), 26.9 (CH3 × 3), 38.8 (C), 65.7 (CH2), 74.4 (CH), 76.6 (CH), 110.6 (C), 111.4 (CH), 122.3 (CH), 129.6 (CH × 2) 133.8 (C), 148.6 (C × 2), 153.7 (C), 161.8 (C), 176.4 (C); FD-HRMS (m/z) calcd for C20H23N2O10 [M+–CH3]: 451.1353, found: 451.1380.
(S,E)-4-[(S)-2,2-Dimethyl-1,3-dioxolan-4-yl]-4-hydroxybut-2-en-2-yl pivalate (19) from 26. To a solution of 26 (5.26 g, 11.8 mmol) in MeOH (100 mL) and THF (100 mL) was added K2CO3 (1.63 g, 11.8 mmol) at –20 ºC, and the mixture was stirred for 30 min. Then, the reaction was quenched with saturated aq. NH4Cl, and the mixture was extracted with Et2O several times. The combined organic layers were washed with saturated aq. NaHCO3 and then with brine, dried over anhydrous MgSO4, filtered, and concentrated under reduced pressure. The residue was purified by column chromatography (silica gel, hexane/EtOAc = 5) to give pure 19 (2.96 g, 10.9 mmol, 92%); a colorless oil; [α]D21 +6.3 (c 0.84, CHCl3); IR (neat) ν 3466, 2983, 2936, 2909, 2876, 1743, 1700, 1481, 1457, 1437, 1383, 1371, 1280, 1258, 1215, 1135, 1066, 1035, 904, 887, 852, 834, 764 cm–1; 1H NMR (300MHz, CDCl3) δ 1.24 (9H, s), 1.37 (3H, s), 1.48 (3H, s), 1.95 (3H, d, J = 1.0 Hz), 2.36 (1H, d, J = 3.5 Hz, -OH), 3.73-3.80 (1H, m), 3.99-4.09 (2H, m), 4.22 (1H, ddd, J = 3.5, 6.6, 9.5 Hz), 5.11 (1H, qd, J = 1.0, 9.5 Hz); 13C NMR (75 MHz, CDCl3) δ 16.0 (CH3), 25.2 (CH3), 26.8 (CH3), 26.9 (CH3 × 3), 38.7 (C), 65.8 (CH2), 69.5 (CH), 79.0 (CH), 109.9 (C), 115.7 (CH), 150.4 (C), 176.7 (C); FD-HRMS (m/z) calcd for C14H24O5 [M+]: 272.1624, found: 272.1599.
(S)-MTPA ester 27a. To a solution of 19 (14.5 mg, 0.0532 mmol), DMAP (a catalytic amount) and Et3N (0.026 mL, 0.19 mmol) in CH2Cl2 (1 mL) was added (R)-(–)-MTPACl (0.030 mL, 0.16 mmol) at 24 ºC, and the mixture was stirred for 10 h. Then, the reaction was quenched with 0.2 M aq. HCl, and the mixture was extracted with Et2O several times. The combined organic layers were washed with brine, dried over anhydrous MgSO4, filtered, and concentrated under reduced pressure. The residue was purified by column chromatography (silica gel, hexane/EtOAc = 30) to give (S)-MTPA ester 27a (23.3 mg, 0.0477 mmol, 90%); a colorless oil; 1H NMR (300MHz, CDCl3) δ 1.24 (9H, s), 1.34 (3H, s), 1.44 (3H, s), 2.06 (3H, brs), 3.62 (3H, brs), 3.79 (1H, dd, J = 4.9, 9.0 Hz), 4.04 (1H, dd, J = 6.6, 9.0 Hz), 4.23 (1H, brddd, J = 4.9, 6.6, 8.0 Hz), 4.94 (1H, brd, J = 10.3 Hz), 5.63 (1H, dd, J = 8.0, 10.3 Hz), 7.34-7.43 (3H, m), 7.54-7.60 (2H, m); FD-HRMS (m/z) calcd for C24H31O7F3 [M+]: 488.2022, found: 488.2004.
(R)-MTPA ester 27b. According to the preparation procedure for 27a, (R)-MTPA ester 27b was prepared from 19 (17.5 mg, 0.0642 mmol), DMAP (a catalytic amount), Et3N (0.032 mL, 0.23 mmol), and (S)-(+)-MTPACl (0.036 mL, 0.19 mmol); a colorless oil; 1H NMR (300MHz, CDCl3) δ 1.25 (9H, s), 1.30 (3H, s), 1.39 (3H, s), 2.04 (3H, d, J = 1.0 Hz), 3.56 (3H, brs), 3.74 (1H, dd, J = 5.0, 9.0 Hz), 3.98 (1H, dd, J = 6.6, 9.0 Hz), 4.19 (1H, brddd, J = 5.0, 6.6, 7.0 Hz), 5.16 (1H, qd, J = 1.0, 10.3 Hz), 5.72 (1H, dd, J = 7.0, 10.3 Hz), 7.36-7.43 (3H, m), 7.49-7.60 (2H, m); FD-HRMS (m/z) calcd for C24H31O7F3 [M+]: 488.2022, found: 488.2004.
(3S)-5-[(tert-Butyldiphenylsilyl)oxy]-3-hydroxypentan-2-yl acetate (30). To a solution of (COCl)2 (2.87 mL, 32.9 mmol) in CH2Cl2 (110 mL) was added DMSO (3.11 mL, 43.8 mmol) at –78 ºC, and the mixture was stirred for 10 min. Then, to the stirred mixture was added slowly a solution of 28 (5.03 g, 11.0 mmol) in CH2Cl2 (22 mL) via cannula, and the mixture was stirred for 15 min. Then, to the mixture was added dropwise Et3N (12.2 mL, 87.5 mmol) at –78 ºC, and the mixture was stirred for 30 min at 0 ºC. Then, H2O was added, and the mixture was extracted with hexane several times. The combined organic layers were washed with brine, dried over anhydrous MgSO4, filtered, and concentrated under reduced pressure to give a crude aldehyde, which was immediately used in the next reaction. To a solution of the crude aldehyde in THF (110 mL) was added MeMgBr (3.0 mol/L in THF, 11.0 mL, 33.0 mmol) at –40 ºC, and the mixture was stirred for 1 h. Then, to the mixture was added Ac2O (6.22 mL, 65.8 mmol) at –40 ºC, and the mixture was stirred for 3 h at 0 ºC. Then, the reaction was quenched with saturated aq. NaHCO3, and the mixture was extracted with EtOAc several times. The combined organic layers were washed with brine, dried over anhydrous MgSO4, filtered, and concentrated under reduced pressure. The residue was roughly purified by column chromatography (silica gel, hexane/EtOAc = 50) to give an inseparable mixture of 29 and small amounts of byproducts, which was used in the next reaction without further purification. To a solution of the above mixture of 29 and byproducts in EtOH (70 mL) was added PPTS (10 mg) at 0 ºC, and the mixture was stirred for 10 h. Then, saturated aq. NaHCO3 was added, and the mixture was extracted with EtOAc several times. The combined organic layers were washed with brine, dried over anhydrous MgSO4, filtered, and concentrated under reduced pressure. The residue was purified by column chromatography (silica gel, hexane/EtOAc = 10) to give 30 (1.74 g, 4.34 mmol, 39% from 28, a 9:5 mixture of diastereomers); a colorless oil; IR (neat) ν 3494, 3071, 3050, 3015, 2957, 2931, 2885, 2858, 1737, 1472, 1463, 1428, 1372, 1244, 1112, 1028, 938, 823, 738, 702, 688, 614 cm–1; 1H NMR (300MHz, CDCl3) δ 1.05 (9H, s), 1.25 (3H×9/14, d, J = 6.4 Hz), 1.26 (3H×5/14, d, J = 6.4 Hz), 1.64-1.75 (2H, m), 2.06 (3H×9/14, s), 2.08 (3H×5/14, s), 2.99 (1H×5/14, brd, J = 3.8 Hz), 3.25 (1H×9/14, brd, J = 2.8 Hz), 3.80-3.99 (3H, m), 4.82-4.95 (1H, m), 7.34-7.48 (6H, m), 7.62-7.70 (4H, m); 13C NMR (75 MHz, CDCl3) δ 14.7 (CH3 × 9/14), 16.0 (CH3×5/14), 19.0 (C), 21.22 (CH3 × 5/14), 21.27 (CH3 × 9/14), 26.8 (CH3 × 3), 34.1 (CH2 × 9/14), 34.7 (CH2 × 5/14), 62.4 (CH2 × 5/14), 62.9 (CH2 × 9/14), 72.6 (CH × 5/14), 72.9 (CH × 9/14), 73.2 (CH × 5/14), 73.5 (CH × 9/14), 127.8(CH × 4), 129.8 (CH × 2), 132.8 (C), 132.9 (C), 135.5 (CH × 4), 170.5 (C × 9/14), 170.6 (C × 5/14); FD-HRMS (m/z) calcd for C23H33O4Si [M+H+]: 401.2148, found: 401.2177.
(3R)-3-Bromo-5-[(tert-butyldiphenylsilyl)oxy]pentan-2-yl acetate (31). To a solution of 30 (247.6 mg, 0.618 mmol) in toluene (9 mL) were added CBr4 (1.04 g, 3.14 mmol) and Bu3P (1.55 mL, 6.28 mmol) at 27 ºC, and the mixture was heated to 70 ºC and stirred for 3.5 h. Then, H2O was added, and the mixture was extracted with EtOAc several times. The combined organic layers were washed with brine, dried over anhydrous MgSO4, filtered, and concentrated under reduced pressure. The residue was purified by column chromatography (silica gel, hexane/EtOAc = 70) to give 31 (241.6 mg, 0.521 mmol, 84%, a 9:5 mixture of diastereomers); a pale yellow oil; IR (neat) ν 3071, 3050, 3014, 2958, 2931, 2881, 2858, 1743, 1472, 1463, 1428, 1372, 1234, 1186, 1112, 1030, 1009, 957, 936, 823, 738, 702, 688, 614 cm–1; 1H NMR (300MHz, CDCl3) δ 1.049 (9H × 9/14, s), 1.054 (9H × 5/14, s), 1.32 (3H × 5/14, d, J = 6.3 Hz), 1.35 (3H × 9/14, d, J = 6.3 Hz), 1.79-1.96 (1H, m), 2.02-2.17 (1H, m), 2.06 (3H × 9/14, s), 2.09 (3H × 5/14, s), 3.76-3.91 (2H, m), 4.31-4.45 (1H, m), 4.99-5.14 (1H, m), 7.34-7.47 (6H, m), 7.61-7.71 (4H, m); 13C NMR (75 MHz, CDCl3) δ 16.7 (CH3 × 5/14), 17.5 (CH3 × 9/14), 19.2 (C), 21.0 (CH3 × 9/14), 21.1 (CH3 × 5/14), 26.8 (CH3 × 3), 37.1 (CH2 × 5/14), 37.2 (CH2 × 9/14), 54.0 (CH × 9/14), 54.9 (CH × 5/14), 61.0 (CH2 × 9/14), 61.1 (CH2 × 5/14), 72.0 (CH × 9/14), 72.5 (CH × 5/14), 127.7 (CH × 4), 129.7 (CH × 2), 133.4 (C), 133.5 (C), 135.47 (CH × 2), 135.54 (CH × 2), 170.06 (C × 9/14), 170.10 (C × 5/14); FD-HRMS (m/z) calcd for C19H22O3Si79Br [M+–t-Bu]: 405.0522, found: 405.0531.
(3R)-3-Bromo-5-[(tert-butyldiphenylsilyl)oxy]pentan-2-ol (32). To a solution of 31 (2.83 g, 6.11 mmol) in CH2Cl2 (60 mL) was added DIBALH (1.02 mol/L in hexane, 18.0 mL, 18.4 mmol) at –78 ºC, and the mixture was stirred at –78 ºC for 1 h. Then, the reaction was quenched with saturated aq. potassium sodium tartrate, and the mixture was stirred at ambient temperature for 2 h. Then, the mixture was extracted with EtOAc several times. The combined organic layers were washed with brine, dried over anhydrous MgSO4, filtered, and concentrated under reduced pressure. The residue was purified by column chromatography (silica gel, hexane/EtOAc = 20) to give 32 (2.41 g, 5.72 mmol, 94%, a 9:5 mixture of diastereomers); a colorless oil; IR (neat) ν 3423, 3071, 3050, 2958, 2930, 2858, 1472, 1463, 1428, 1389, 1362, 1259, 1185, 1112, 1009, 933, 823, 738, 702, 688, 614 cm–1; 1H NMR (300MHz, CDCl3) δ 1.05 (9H, s), 1.29 (3H × 5/14, d, J = 6.2 Hz), 1.32 (3H × 9/14, d, J = 6.2 Hz), 1.97-2.22 (2H, m), 2.28 (1H × 9/14, brd, J = 6.9 Hz, OH), 2.50 (1H × 5/14, brd, J = 6.5 Hz, OH), 3.72-4.02 (3H, m), 4.32 (1H × 9/14, brtd, J = 4.2, 9.0 Hz), 4.42 (1H × 5/14, brtd, J = 4.3, 8.1 Hz), 7.34-7.48 (6H, m), 7.62-7.70 (4H, m); 13C NMR (75 MHz, CDCl3) δ 19.1 (C), 19.4 (CH3 × 5/14), 21.3 (CH3 × 9/14), 26.8 (CH3 × 3), 36.4 (CH2 × 5/14), 38.3 (CH2 × 9/14), 61.2 (CH2 × 5/14), 61.40 (CH × 5/14), 61.45 (CH2 × 9/14), 62.4 (CH × 9/14), 70.1 (CH × 9/14), 70.6 (CH × 5/14), 127.7(CH × 4), 129.7 (CH ×2 ), 133.3 (C), 133.4 (C), 135.47 (CH × 2), 135.53 (CH × 2); FD-HRMS (m/z) calcd for C21H30O2Si79Br [M+H+]: 421.1198, found: 421.1185.
(R)-3-Bromo-5-[(tert-butyldiphenylsilyl)oxy]pentan-2-one (33). To a solution of 32 (530 mg, 1.26 mmol) in CH2Cl2 (15 mL) was added DMPI (1.18 g, 2.78 mmol) at 23 ºC, and the mixture was stirred for 1 h. Then, NaHCO3 (1.0 g) and saturated aq. Na2S2O3 (10 mL) were added, and the mixture was extracted with hexane several times. The combined organic layers were washed with brine, dried over anhydrous MgSO4, filtered, and concentrated under reduced pressure. The residue was purified by column chromatography (silica gel, hexane/EtOAc = 50) to give 33 (515 mg, 1.23 mmol, 98%). Ketone 33 was immediately used in the next reaction due to instability; a colorless oil; [α]D24 +59.6 (c 0.810, CHCl3); IR (neat) ν 3071, 3050, 3014, 2999, 2958, 2931, 2884, 2858, 1720, 1472, 1463, 1428, 1390, 1361, 1257, 1230, 1146, 1112, 1008, 998, 926, 823, 738, 702, 688, 615 cm–1; 1H NMR (300MHz, CDCl3) δ 1.05 (9H, s), 2.06 (1H, tdd, J = 4.5 8.6, 14.5 Hz), 2.30 (1H, tdd, J = 5.6, 8.1, 14.5 Hz), 2.37 (3H, s), 3.72-3.85 (2H, m), 4.61 (1H, dd, J = 5.6, 8.6 Hz), 7.34-7.48 (6H, m), 7.60-7.69 (4H, m); 13C NMR (75 MHz, CDCl3) δ 19.2 (C), 26.6 (CH3), 26.8 (CH3 × 3), 36.1 (CH2), 51.0 (CH), 60.8 (CH2), 127.7 (CH × 4), 129.8 (CH × 2), 133.18 (C), 133.23 (C), 135.44 (CH × 2), 135.51 (CH × 2), 201.6 (C); FD-HRMS (m/z) calcd for C21H28O2Si79Br [M+H+]: 419.1042, found: 419.1069.
(R)-[3-Bromo-3-(2-methyl-1,3-dioxolan-2-yl)propoxy](tert-butyl)diphenylsilane (34). To a solution of 33 (492 mg, 1.17 mmol) and (TMSOCH2)2 (2.88 mL, 11.7 mmol) in CH2Cl2 (10 mL) was added TMSOTf (0.113 mL, 0.587 mmol) at –20 ºC, and the mixture was stirred for 18 h. Then, saturated aq. NaHCO3 (10 mL) was added, and the mixture was extracted with hexane several times. The combined organic layers were washed with brine, dried over anhydrous MgSO4, filtered, and concentrated under reduced pressure. The residue was purified by column chromatography (silica gel, hexane/EtOAc = 50) to give 34 (552 mg, 1.18 mmol, 100%); a colorless oil; [α]D25 +22.9 (c 0.910, CHCl3); IR (neat) ν 3071, 3049, 2957, 2930, 2883, 2858, 1472, 1463, 1446, 1428, 1380, 1362, 1307, 1274, 1252, 1218, 1188, 1145, 1112, 1057, 1038, 1008, 998, 949, 937, 874, 823, 738, 702, 688, 614 cm–1; 1H NMR (300MHz, CDCl3) δ 1.05 (9H, s), 1.50 (3H, s), 1.81 (1H, tdd, J = 3.6, 11.3, 14.4 Hz), 2.32 (1H, dddd, J = 2.0, 6.1, 9.6, 14.4 Hz), 3.79-3.93 (2H, m), 3.96-4.07 (4H, m), 4.29 (1H, dd, J = 2.0, 11.3 Hz), 7.34-7.47 (6H, m), 7.64-7.72 (4H, m); 13C NMR (75 MHz, CDCl3) δ 19.2 (C), 20.8 (CH3), 26.8 (CH3 × 3), 36.7 (CH2), 55.8 (CH), 61.2 (CH2), 65.3 (CH2), 65.4 (CH2), 109.7 (C), 127.7 (CH × 4), 129.6 (CH × 2), 133.5 (C), 133.6 (C) 135.5 (CH × 2), 135.6 (CH × 2); FD-HRMS (m/z) calcd for C23H32O3Si79Br [M+–H]: 463.1304, found: 463.1278.
(R)-2-({3-Bromo-5-[(tert-butyldiphenylsilyl)oxy]-2-methylpentan-2-yl}oxy)ethanol (35). To compound 34 (9.10 g, 19.6 mmol) was added Me3Al (1.8 mol/L in toluene, 70 mL, 126 mmol) at ambient temperature, and the mixture was refluxed for 18 h. Then, the mixture was cooled to ambient temperature and diluted with hexane (100 mL). To the mixture was added 1 M aq. HCl (150 mL) carefully. The mixture was extracted with EtOAc several times. The combined organic layers were washed with brine, dried over anhydrous MgSO4, filtered, and concentrated under reduced pressure. The residue was purified by column chromatography (silica gel, hexane/EtOAc = 5) to give 35 (8.15 g, 17.0 mmol, 87%); a colorless oil; [α]D24 +21.0 (c 0.990, CHCl3); IR (neat) ν 3446, 3071, 3049, 2957, 2930, 2858, 1472, 1428, 1385, 1366, 1233, 1188, 1133, 1112, 1051, 1008, 998, 940, 893, 823, 738, 702, 688, 665, 614 cm–1; 1H NMR (300MHz, CDCl3) δ 1.06 (9H, s), 1.32 (3H, s), 1.35 (3H, s), 1.80 (1H, tdd, J = 3.4, 11.1, 14.6 Hz), 2.09 (1H, brs, OH), 2.24 (1H, dddd, J = 1.4, 6.2, 9.4, 14.6 Hz), 3.51 (2H, brt, J = 4.4 Hz), 3.69 (2H, brs), 3.80-3.95 (2H, m), 4.39 (1H, dd, J = 1.4, 11.1 Hz), 7.33-7.48 (6H, m), 7.61-7.73 (4H, m); 13C NMR (75 MHz, CDCl3) δ 19.2 (C), 22.6 (CH3), 23.9 (CH3), 26.9 (CH3 × 3), 36.5 (CH2), 60.0 (CH), 61.6 (CH2), 62.1 (CH2), 62.6 (CH2), 76.6 (C), 127.7 (CH × 4), 129.7 (CH × 2), 133.5 (C), 133.6 (C), 135.5 (CH × 2), 135.6 (CH × 2); FD-HRMS (m/z) calcd for C24H36O3Si79Br [M+H+]: 479.1617, found: 479.1601.
(R)-2-({3-Bromo-5-[(tert-butyldiphenylsilyl)oxy]-2-methylpentan-2-yl}oxy)acetic acid (36). To a solution of 35 (8.15 g, 17.0 mmol), Et3N (11.8 mL, 85.0 mmol), and DMSO (65 mL) in CH2Cl2 (100 mL) was added SO3·pyridine complex (8.12 g, 51.0 mmol) at 0 ºC, and the mixture was stirred for 1.5 h. Then, H2O (100 mL) was added, and the mixture was extracted with hexane several times. The combined organic layers were washed with brine, dried over anhydrous MgSO4, filtered, and concentrated under reduced pressure. The residue was roughly purified by column chromatography (silica gel, hexane/EtOAc = 20) to give an aldehyde (6.77 g). To a solution of the aldehyde and 2-methylbut-2-ene (7.2 mL, 86 mmol) in t-BuOH (80 mL) and H2O (40 mL) were added NaH2PO4 (10.2 g, 85.0 mmol) and NaClO2 (3.84 g, 42.5 mmol) at 0 ºC, and the mixture was stirred at 21 ºC for 10 h. Then, the mixture was extracted with CHCl3 several times. The combined organic layers were dried over anhydrous MgSO4, filtered, and concentrated under reduced pressure. The residue was purified by column chromatography (silica gel, hexane/EtOAc = 5) to give 36 (6.95 g, 14.1 mmol, 83%). Optical purity of 36 was able to be determined by chiral HPLC analysis using a pre-packed column supplied by Daicel Corporation (Chiralpak IA, 250 mm × 4.6 mm ID, hexane:CH2Cl2:TBME:TFA = 200:86:4:1; flow rate: 1.0 mL/min, UV 254 nm detection). (R)-36: tR =10.1 min; (S)-36: tR = 12.5 min. The enantiomeric excess of 36 obtained here was 82.4%ee; a colorless oil; [α]D22 +23 (c 0.15, CHCl3); IR (neat) ν 3500-2400 (broad), 3071, 3050, 2957, 2931, 2884, 2857, 1732, 1471, 1428, 1386, 1370, 1236, 1188, 1133, 1112, 1053, 1008, 998, 939, 911, 890, 823, 738, 703, 688, 614 cm–1; 1H NMR (300MHz, CDCl3) δ 1.06 (9H, s), 1.36 (3H, s), 1.37 (3H, s), 1.81 (1H, tdd, J = 3.4, 11.3, 14.6 Hz), 2.15 (1H, dddd, J = 1.7, 6.2, 9.4, 14.6 Hz), 3.81-3.95 (2H, m), 4.06 (2H, s), 4.35 (1H, dd, J = 1.7, 11.3 Hz) 7.34-7.48 (6H, m), 7.61-7.70 (4H, m); 13C NMR (75 MHz, CDCl3) δ 19.1 (C), 22.7 (CH3), 23.1 (CH3), 26.8 (CH3 × 3), 36.4 (CH2), 59.5 (CH), 60.0 (CH2), 61.3 (CH2), 78.6 (C), 127.7 (CH×4), 129.7 (CH × 2), 133.3 (C), 133.4 (C), 135.4 (CH × 2), 135.5 (CH × 2), 173.0 (C); FD-HRMS (m/z) calcd for C24H34O4Si79Br [M+H+]: 493.1410, found: 493.1380.
(R)-2-({3-Bromo-2-methyl-5-[(triisopropylsilyl)oxy]pentan-2-yl}oxy)acetic acid (20). To a solution of 36 (2.13 g, 4.32 mmol) in MeOH (10 mL) and benzene (30 mL) was added TMSCHN2 (2 mol/L in Et2O, 4.32 mL, 8.64 mmol) at 26 ºC, and the mixture was stirred for 15 min. Then, the mixture was concentrated under reduced pressure to give a crude methyl ester, which was used in the next reaction without purification. To a solution of the crude methyl ester in THF (40 mL) was added Bu4NF (1 mol/L in THF, 8.6 mL, 8.6 mmol) at 26 ºC, and the mixture was stirred for 1 h. Then, saturated aq. NH4Cl was added, and the mixture was extracted with hexane several times. The combined organic layers were washed with brine, dried over anhydrous MgSO4, filtered, and concentrated under reduced pressure. The residue was purified by column chromatography (silica gel, hexane/EtOAc = 10 → 5 → 2 → 1) to give 37 (993 mg, a colorless oil), which was used immediately in the next reaction. To a solution of the above 37, imidazole (680 mg, 9.99 mmol), and DMAP (a catalytic amount) in DMF (30 mL) was added TIPSCl (1.05 mL, 4.96 mmol) at 25 ºC, and the mixture was stirred for 3 h. Then, saturated aq. NaHCO3 was added, and the mixture was extracted with hexane several times. The combined organic layers were washed with 1 mol/L aq. HCl and then with brine, dried over anhydrous MgSO4, filtered, and concentrated under reduced pressure. The residue was roughly purified by column chromatography (silica gel, hexane/EtOAc = 50) to give a mixture of a TIPS ether and TIPSOH. The mixture was dissolved in MeOH (30 mL), and to the stirred mixture was added 1 mol/L aq. K2CO3 (10 mL, 10 mmol) at 26 ºC. After the mixture was stirred for 3 d, the mixture was acidified with 1 mol/L aq. HCl and extracted with CHCl3 several times. The combined organic layers were dried over anhydrous MgSO4, filtered, and concentrated under reduced pressure. The residue was purified by column chromatography (silica gel, hexane/EtOAc = 5) to give 20 (998.6 mg, 2.427 mmol, 56% over 4 steps); a colorless oil; [α]D23 +20.3 (c 0.105, CHCl3); IR (neat) ν 3600-2400 (broad), 2926, 2867, 1737, 1463, 1426, 1385, 1369, 1246, 1134, 1110, 1071, 1057, 1014, 996, 883, 745, 681, 659, 642 cm–1; 1H NMR (300MHz, CDCl3) δ 0.98-1.18 (21H, m), 1.38 (3H, s), 1.39 (3H, s), 1.86 (1H, tdd, J = 3.3, 11.1, 14.5 Hz), 2.13 (1H, dddd, J = 1.7, 7.1, 8.6, 14.5 Hz), 3.85-3.94 (2H, m), 4.06 (2H, s), 4.35 (1H, dd, J = 1.7, 11.1 Hz); 13C NMR (75 MHz, CDCl3) δ 11.9 (CH × 3), 18.0 (CH3 × 6), 22.8 (CH3), 22.9 (CH3), 36.9 (CH2), 59.8 (CH), 59.9 (CH2), 60.7 (CH2), 78.8 (C), 172.0 (C); FD-HRMS (m/z) calcd for C17H36O4Si79Br [M+H+]: 411.1566, found: 411.1576.
(1S,2E)-1-[(S)-2,2-Dimethyl-1,3-dioxolan-4-yl]-3-(pivaloyloxy)but-2-en-1-yl 2-[{(R)-3-bromo-2-
methyl-5-[(triisopropylsilyl)oxy]pentan-2-yl}oxy]acetate (8). To a stirred solution of 19 (96.0 mg, 0.353 mmol), 20 (131 mg, 0.318 mmol), and DMAP (a catalytic amount) in CH2Cl2 (5 mL) was added EDCI·HCl (122 mg, 0.638 mmol) at 23 ºC, and the mixture was stirred for 10 h. Then, H2O was added, and the mixture was extracted with Et2O several times. The combined organic layers were washed with brine, dried over anhydrous MgSO4, filtered, and concentrated under reduced pressure. The residue was purified by column chromatography (silica gel, hexane/EtOAc = 20) to give 8 (189 mg, 0.284 mmol, 89%); a colorless oil; [α]D21 +22.5 (c 0.815, CHCl3); IR (neat) ν 2960, 2942, 2868, 1747, 1696, 1481, 1464, 1384, 1370, 1281, 1258, 1228, 1193, 1109, 1071, 1014, 998, 968, 940, 920, 883, 847, 746, 681, 659, 638 cm–1; 1H NMR (300MHz, CDCl3) δ 0.98-1.18 (21H, m), 1.23 (9H, s), 1.35 (3H, s), 1.37 (3H, s), 1.40 (3H, s), 1.44 (3H, s), 1.82 (1H, brtdd, J = 3.3, 11.3, 14.5 Hz), 2.01 (3H, s), 2.31 (1H, brtd, J = 7.8, 14.5 Hz), 3.79 (1H, dd, J = 5.1, 9.0 Hz), 3.84-3.91 (2H, m), 4.03 (1H, dd, J = 6.5, 9.0 Hz), 4.05 (1H, d, J = 15.5 Hz), 4.15 (1H, d, J = 15.5 Hz), 4.15-4.23 (1H, m), 4.29 (1H, brd, J = 11.3 Hz), 5.00 (1H, d, J = 10.2 Hz), 5.48 (1H, dd, J = 7.3, 10.2 Hz); 13C NMR (75 MHz, CDCl3) δ 11.8 (CH × 3), 16.2 (CH3), 17.9 (CH3 × 6), 22.4 (CH3), 23.9 (CH3), 25.3 (CH3), 26.5 (CH3), 26.9 (CH3×3), 36.5 (CH2), 38.7 (C), 58.9 (CH), 60.4 (CH2), 60.8 (CH2), 65.7 (CH2), 71.9 (CH), 76.6 (CH), 78.0 (C), 110.2 (C), 111.8 (CH), 152.7 (C), 169.7 (C), 176.4 (C); CI-HRMS (m/z) calcd for C34H67NO8Si79Br [M+i-PrNH3+]: 724.3819, found: 724.3810.
(2R,3S,E)-Methyl 2-({(R)-3-bromo-2-methyl-5-[(triisopropylsilyl)oxy]pentan-2-yl}oxy)-5-[(R)-2,2-
dimethyl-1,3-dioxolan-4-yl]-3-methyl-3-(pivaloyloxy)pent-4-enoate (9). To a solution of 8 (196 mg, 0.294 mmol) in toluene (5 mL) was added TMSCl (0.375 mL, 2.95 mmol) at –78 ºC, and the mixture was stirred for 5 min. To the mixture was added KHMDS (0.5 mol/L in toluene, 5.90 mL, 2.95 mmol), and the mixture was stirred for 30 min. Then, diethyl malonate (0.90 mL, 5.9 mmol) was added, and the mixture was warmed to 0 ºC and stirred for 1 h. Then, saturated aq. NH4Cl was added, and the mixture was extracted with CHCl3 several times. The combined organic layers were dried over anhydrous MgSO4, filtered, and concentrated under reduced pressure. The residue was roughly purified by short column chromatography (silica gel, hexane/EtOAc = 10 → CHCl3) to give a crude carboxylic acid. To a solution of the crude carboxylic acid in benzene (3.75 mL) and MeOH (1.25 mL) was added TMSCHN2 (2 mol/L in Et2O, 0.44 mL, 0.88 mmol) at 26 ºC, and the mixture was stirred for 15 min. Then, the mixture was concentrated under reduced pressure. The residue was purified by column chromatography (silica gel, hexane/EtOAc = 30) to give an 8:1 mixture of 9 and its C7-epimer (181.3 mg, 0.267 mmol, 91% over 2 steps); a colorless oil; [α]D21 –4.88 (c 1.00, CHCl3); IR (neat) ν 2943, 2868, 1755, 1737, 1480, 1463, 1435, 1390, 1380, 1370, 1282, 1254, 1210, 1157, 1130, 1107, 1063, 1030, 1014, 996, 967, 939, 918, 902, 882, 864, 877, 768, 749, 681, 660, 645 cm–1; 1H NMR (300MHz, CDCl3) δ 0.98-1.18 (21H, m), 1.18 (3H, s), 1.19 (9H, s), 1.37 (3H, s), 1.38 (3H, s), 1.43 (3H, s), 1.64 (3H, s), 1.77 (1H, tdd, J = 3.6, 11.3, 14.8 Hz), 2.46 (1H, brtd, J = 8.2, 14.8 Hz), 3.55 (1H, t, J = 7.8 Hz), 3.63 (3H, s), 3.82-3.97 (2H, m), 4.09 (1H, dd, J = 6.3, 8.2 Hz), 4.20 (1H, brd, J = 11.3 Hz), 4.52 (1H, brq, J = 7.1 Hz), 4.88 (1H, s), 5.64 (1H, dd, J = 7.7, 15.7 Hz), 5.98 (1H, d, J = 15.7 Hz); 13C NMR (75 MHz, CDCl3) δ 11.9 (CH × 3), 17.9 (CH3 × 6), 20.8 (CH3), 21.1 (CH3), 23.6 (CH3), 25.7 (CH3), 26.6 (CH3), 27.1 (CH3 × 3), 36.6 (CH2), 39.4 (C), 51.4 (CH3), 61.0 (CH2), 61.1 (CH), 69.4 (CH2), 74.6 (CH), 76.6 (CH), 79.3 (C), 81.4 (C), 109.3 (C), 127.5 (CH), 133.6 (CH), 171.4 (C), 177.0 (C); FD-HRMS (m/z) calcd for C32H59O8Si79Br [M+]: 678.3163, found: 678.3172.
(2S,3S,E)-2-({(R)-3-Bromo-2-methyl-5-[(triisopropylsilyl)oxy]pentan-2-yl}oxy)-5-[(R)-2,2-dimethyl-
1,3-dioxolan-4-yl]-3-methylpent-4-ene-1,3-diol (39). To a solution of a mixture of 9 and its C7-epimer (181 mg, 0.266 mmol) in CH2Cl2 (4 mL) was added DIBALH (1.02 mol/L in hexane, 2.61 mL, 2.66 mmol) at –20 ºC, and the mixture was stirred at –20 ºC for 1 h. Then, MeOH was added dropwise, and then saturated aq. potassium sodium tartrate was added. The mixture was stirred for 1 h. Then, the mixture was extracted with EtOAc several times. The combined organic layers were washed with brine, dried over anhydrous MgSO4, filtered, and concentrated under reduced pressure. The residue was purified by column chromatography (silica gel, hexane/EtOAc = 5 → 2) to give 39 (a colorless oil, 75.7 mg, 0.133 mmol, 50%) and 38 (a colorless oil, 39.9 mg, 0.0705 mmol, 27%). The products from C7-epimeric 9 were separable at this stage. Aldehyde 38 was immediately reduced to 39 as follows. To a solution of the above 38 (39.9 mg, 0.0705 mmol) in MeOH (1 mL) was added NaBH4 (8.0 mg, 0.21 mmol) at 26 ºC, and the mixture was stirred for 30 min. Then, saturated aq. NH4Cl was added, and the mixture was extracted with EtOAc several times. The combined organic layers were washed with brine, dried over anhydrous MgSO4, filtered, and concentrated under reduced pressure. The residue was purified by column chromatography (silica gel, hexane/EtOAc = 5 → 2) to give 39 (a colorless oil, 34.3 mg, 0.0604 mmol, 86% from 38). The combined yield of 39 from 9 was 73% (total amount of 39: 110.0 mg, 0.194 mmol); a colorless oil; [α]D21 +14 (c 0.22, CHCl3); IR (neat) ν 3416, 2926, 2867, 1463, 1454, 1381, 1370, 1246, 1222, 1156, 1128, 1107, 1061, 1013, 996, 978, 939, 919, 882, 865, 745, 681 cm–1; 1H NMR (300MHz, CDCl3) δ 1.00-1.20 (21H, m), 1.35 (6H, s), 1.38 (3H, s), 1.39 (3H, s), 1.43 (3H, s), 1.87 (1H, tdd, J = 3.6, 11.2, 14.5 Hz), 2.23 (1H, dddd, J = 1.6, 6.7, 8.9, 14.5 Hz), 2.42 (1H, t, J = 5.8 Hz, OH), 2.77 (1H, brs, OH), 3.51 (1H, t, J = 4.3 Hz), 3.61 (1H, t, J = 8.0 Hz), 3.66-3.76 (2H, m), 3.85-3.94 (2H, m), 4.10 (1H, dd, J = 6.1, 8.1 Hz), 4.24 (1H, dd, J = 1.6, 11.2 Hz), 4.54 (1H, ddd, J = 6.1, 7.3, 8.1 Hz), 5.77 (1H, dd, J = 7.3, 15.5 Hz), 5.90 (1H, d, J = 15.5 Hz); 13C NMR (75 MHz, CDCl3) δ 11.9 (CH × 3), 18.0 (CH3 × 6), 23.5 (CH3), 24.6 (CH3), 25.0 (CH3), 25.9 (CH3), 26.7 (CH3), 37.1 (CH2), 61.1 (CH2), 63.4 (C), 63.8 (CH), 69.5 (CH2), 75.3 (C), 76.5 (CH), 76.7 (CH), 78.2 (C), 109.4 (C), 127.1 (CH), 138.5 (CH); FD-HRMS (m/z) calcd for C26H52O6Si79Br [M+H+]: 567.2717, found: 567.2723.
(E)-3-[(2S,4S,5S)-5-{[(R)-3-Bromo-5-hydroxy-2-methylpentan-2-yl]oxy}-2-(4-chlorophenyl)-4-
methyl-1,3-dioxan-4-yl]acrylaldehyde (42). To a solution of 39 (110 mg, 0.194 mmol) in CH2Cl2 (4 mL) were added 4-chlorobenzaldehyde dimethyl acetal (0.0640 mL, 0.387 mmol) and CSA (a catalytic amount) at 26 ºC, and the mixture was stirred for 13 h. Then, saturated aq. NaHCO3 was added, and the mixture was extracted with EtOAc several times. The combined organic layers were washed with brine, dried over anhydrous MgSO4, filtered, and concentrated under reduced pressure. The residue was roughly purified by column chromatography (silica gel, hexane/EtOAc = 30) to give a crude mixture of 40, 41a, and 41b (49.5 mg). [Acetals 41a and 41b were partly separable from the crude mixture by HPLC (YMC-Pack SIL-06, 5 μm, 250 mm × 10 mm ID, hexane/CH2Cl2 = 1; flow rate: 5.0 mL/min, UV254 detection), and their 1H NMR spectra were measured. 41a: 1H NMR (400MHz, CDCl3) δ 1.36 (3H, s), 1.40 (3H, s), 1.47 (3H, s), 1.87 (1H, tdd, J = 4.1, 11.5, 14.7 Hz), 2.26 (1H, dddd, J = 2.0, 6.2, 9.0, 14.7 Hz), 3.67 (1H, t, J = 10.7 Hz), 3.73 (1H, dd, J = 7.3, 8.2 Hz), 3.77 (1H, dd, J = 4.4, 10.7 Hz), 3.79-3.94 (2H, m), 4.03 (1H, dd, J = 4.4, 10.7 Hz), 4.13 (1H, dd, J = 2.0, 11.5 Hz), 4.34 (1H, dd, J = 6.3, 8.2 Hz), 4.74 (1H, brq, J = 6.9 Hz), 5.69 (1H, s), 5.96 (1H, s), 5.99 (1H, dd, J = 7.3, 16.2 Hz), 6.19 (1H, dd, J = 0.9, 16.2 Hz), 7.34 (2H, d, J = 8.4 Hz), 7.36 (2H, d, J = 8.4 Hz), 7.42 (2H, d, J = 8.4 Hz), 7.43 (2H, d, J = 8.4 Hz); 41b: 1H NMR (400MHz, CDCl3) δ 1.35 (3H, s), 1.39 (3H, s), 1.45 (3H, s), 1.84 (1H, tdd, J = 4.1, 11.5, 14.6 Hz), 2.21 (1H, dddd, J = 2.0, 7.0, 8.2, 14.6 Hz), 3.66 (1H, t, J = 10.7 Hz), 3.76 (1H, dd, J = 4.5, 10.7 Hz), 3.76 -3.84 (2H, m), 3.80 (1H, dd, J = 6.4, 7.9 Hz), 4.02 (1H, dd, J = 4.5, 10.7 Hz), 4.10 (1H, dd, J = 2.0, 11.5 Hz), 4.21 (1H, dd, J = 6.9, 7.9 Hz), 4.76 (1H, brq, J = 6.8 Hz), 5.66 (1H, s), 5.87 (1H, s), 5.99 (1H, dd, J = 7.2, 16.2 Hz), 6.17 (1H, dd, J = 0.8, 16.2 Hz), 7.34 (2H, d, J = 8.4 Hz), 7.36 (2H, d, J = 8.4 Hz), 7.39 (2H, d, J = 8.4 Hz), 7.45 (2H, d, J = 8.4 Hz).] The above crude mixture was divided into two batches (31.0 mg and 18.5 mg), and each batch was subjected to the following procedure. To a solution of the crude mixture of 40, 41a, and 41b in MeCN (1.5 mL) was added 0.5 mol/L aq. HCl (0.5 mL) at 0 ºC, and the mixture was stirred for 36 h. Then, pH 7 buffer (1 mL) and NaIO4 (more than 2 equiv.) were added, and the mixture was stirred for 2 h at 26 ºC. Then, saturated aq. NaHCO3 was added, and the mixture was extracted with EtOAc several times. The combined organic layers were washed with saturated aq. Na2S2O3 and then with brine, dried over anhydrous MgSO4, filtered, and concentrated under reduced pressure. The residue was purified by column chromatography (silica gel, hexane/EtOAc = 15) to give 42 (total production of two batches: 24.5 mg, 0.0531 mmol, 27% from 39); a pale yellow oil; [α]D22 +2.46 (c 0.820, CHCl3); IR (neat) ν 3446, 3060, 2981, 2933, 2875, 2733,1689, 1633, 1603, 1494, 1464, 1418, 1404, 1389, 1371, 1301, 1240, 1220, 1192, 1160, 1139, 1129, 1089, 1031, 1016, 986, 949, 914, 871, 820, 805, 787, 733, 671, 647, 622 cm–1; 1H NMR (300MHz, CDCl3) δ 1.39 (3H, s), 1.42 (3H, s), 1.52 (3H, s), 1.88 (1H, tdd, J = 4.0, 11.6, 14.6 Hz), 2.24 (1H, dddd, J = 1.9, 6.4, 9.0, 14.6 Hz), 3.56 (1H, t, J = 11.0 Hz), 3.84-3.98 (2H, m), 3.85 (1H, dd, J = 4.8, 10.9 Hz), 4.11 (1H, dd, J = 4.8, 11.2 Hz), 4.19 (1H, dd, J = 1.9, 11.6 Hz), 5.59 (1H, s), 6.48 (1H, dd, J = 7.9, 16.4 Hz), 7.24 (1H, d, J = 16.4 Hz), 7.35 (2H, d, J = 8.5 Hz), 7.42 (2H, d, J = 8.5 Hz), 9.67 (1H, d, J = 7.9 Hz); 13C NMR (75 MHz, CDCl3) δ 23.0 (CH3), 24.4 (CH3), 28.0 (CH3), 36.1 (CH2), 60.7 (CH2), 62.2 (CH), 69.0 (CH2), 70.0 (CH), 78.7 (C), 79.2 (C), 95.6 (CH), 127.5 (CH × 2), 128.5 (CH × 2), 133.5 (CH), 134.9 (C), 135.9 (C), 156.4 (CH), 193.5 (CH); FD-HRMS (m/z) calcd for C20H27O535Cl79Br [M+H+]: 461.0730, found: 461.0701.
(E)-3-[(2S,4S,5S)-5-({(R)-3-Bromo-2-methyl-5-[(triisopropylsilyl)oxy]pentan-2-yl}oxy)-2-(4-chloro-
phenyl)-4-methyl-1,3-dioxan-4-yl]prop-2-en-1-ol (43). To a solution of 42 (23.8 mg, 0.0515 mmol) and 2,6-lutidine (0.037 mL, 0.32 mmol) in CH2Cl2 (1 mL) was added TIPSOTf (0.042 mL, 0.16 mmol) at 0 ºC, and the mixture was stirred for 1 h. Then, saturated aq. NaHCO3 was added, and the mixture was extracted with hexane several times. The combined organic layers were washed with brine, dried over anhydrous MgSO4, filtered, and concentrated under reduced pressure to give a crude TIPS ether, which was used in the next reaction without purification. To a solution of the crude TIPS ether in MeOH (1 mL) were added CeCl3·7H2O (59 mg, 0.16 mmol) and NaBH4 (6.0 mg, 0.16 mmol) at –78 ºC, and the mixture was stirred for 30 min. Then, saturated aq. NH4Cl was added, and the mixture was extracted with EtOAc several times. The combined organic layers were washed with brine, dried over anhydrous MgSO4, filtered, and concentrated under reduced pressure. The residue was purified by column chromatography (silica gel, hexane/EtOAc = 10) to give 43 (29.9 mg, 0.0482 mmol, 94% over 2 steps); a colorless oil; [α]D22 +6.46 (c 0.465, CHCl3); IR (neat) ν 3422, 2941, 2926, 2866, 1494, 1464, 1419, 1387, 1369, 1234, 1130, 1104, 1088, 1016, 986, 882, 813, 744 cm–1; 1H NMR (300MHz, CDCl3) δ 0.98-1.18 (21H, m), 1.35 (3H, s), 1.38 (3H, s), 1.43 (3H, s), 1.82 (1H, brtdd, J = 3.7, 11.3, 14.5 Hz), 2.25 (1H, brdddd, J = 1.0, 6.8, 8.9, 14.5 Hz), 3.66 (1H, brt, J = 10.4 Hz), 3.74 (1H, dd, J = 3.6, 10.7 Hz), 3.83-3.96 (2H, m), 4.02 (1H, dd, J = 3.6, 9.9 Hz), 4.15 (1H, brdd, J = 1.0, 11.3 Hz), 4.24 (2H, d, J = 3.3 Hz), 5.74 (1H, s), 5.98-6.12 (2H, m), 7.33 (2H, d, J = 8.5 Hz), 7.43 (2H, d, J = 8.5 Hz); 13C NMR (75 MHz, C6D6) δ 12.3 (CH × 3), 18.2 (CH3 × 6), 23.5 (CH3), 23.9 (CH3), 29.5 (CH3), 37.2 (CH2), 61.6 (CH2), 62.7 (CH), 63.3 (CH2), 68.5 (CH2), 70.7 (CH), 78.1 (C), 78.7 (C), 94.8 (CH), 128.1 (CH × 2), 128.5 (CH × 2), 130.0 (CH), 132.3 (CH), 134.6 (C), 137.9 (C); FD-HRMS (m/z) calcd for C29H49O5Si35Cl79Br [M+H+]: 619.2221, found: 619.2184.
(R)-MTPA ester 45b. To a solution of 43 (16.8 mg, 0.0271 mmol) in EtOH (3 mL) were added excess amount of Zn and NH4Cl and reflux for 8 h. Then, the mixture was filtered through a Celite pad. The filtrate was diluted with H2O and extracted with EtOAc several times. The combined organic layers were washed with brine (10 mL), dried over anhydrous MgSO4, filtered, and concentrated under reduced pressure. The residue was purified by column chromatography (silica gel, hexane/EtOAc = 2) to give 44 (7.4 mg, 0.026 mmol, 96%) as a colorless oil. [1H NMR data of 44: 1H NMR (300 MHz, CDCl3) δ 1.49 (3H, s), 3.65 (1H, t, J = 10.7 Hz), 3.80-3.91 (1H, m), 4.02 (1H, dd, J = 5.0, 10.7 Hz), 4.29 (2H, brd, J = 4.3 Hz), 5.75 (1H, s), 6.08 (1H, brtd, J = 4.3, 16.1 Hz), 6.17 (1H, brd, J = 16.1 Hz), 7.33 (2H, d, J = 8.6 Hz), 7.43 (2H, d, J = 8.6 Hz).] Compound 44 was immediately used in the next reaction. To a solution of 44 (6.0 mg, 0.021 mmol), DMAP (a catalytic amount) and Et3N (0.020 mL, 0.210 mmol) in CH2Cl2 (1 mL) was added TBDPSCl (0.009 mL, 0.035 mmol), and the mixture was stirred for 4 h at 25 ºC. Then, (S)-(+)-MTPACl (0.012 mL, 0.064 mmol) was added at 25 ºC, and the mixture was stirred for 10 h. Then, 0.2 M aq. HCl was added, and the mixture was extracted with Et2O several times. The combined organic layers were washed with brine, dried over anhydrous MgSO4, filtered, and concentrated under reduced pressure. The residue was purified by column chromatography (silica gel, hexane/EtOAc = 30) to give (R)-MTPA ester 45b (9.8 mg, 0.013 mmol, 62%); a colorless oil; 1H NMR (300MHz, CDCl3) δ 1.04 (9H, s), 1.26 (3H, s), 3.51 (3H, s), 3.69 (1H, brt, J = 10.7 Hz), 4.17 (1H, dd, J = 5.1, 10.5 Hz), 4.22 (1H, brddd, J = 1.0, 3.3, 14.5 Hz), 4.27(1H, brddd, J = 1.0, 3.3, 14.5 Hz), 5.16 (1H, dd, J = 5.1, 10.8 Hz), 5.67 (1H, s), 5.86 (1H, brtd, J = 3.3, 16.1 Hz), 5.94 (1H, brd, J = 16.1 Hz), 7.28-7.51 (11H, m), 7.60-7.69 (4H, m); FD-HRMS (m/z) calcd for C40H41O6F3Si35Cl [M+–H]: 737.2313, found: 737.2345.
(S)-MTPA ester 45a. According to the preparation procedure for 45b, (S)-MTPA ester 45a was prepared from 44 (4.8 mg, 0.016 mmol), DMAP (a catalytic amount), Et3N (0.016 mL, 0.17 mmol), TBDPSCl (0.0070 mL, 0.026 mmol), and (R)-(–)-MTPACl (0.011 mL, 0.059 mmol); a colorless oil; 1H NMR (300MHz, CDCl3) δ 1.04 (9H, s), 1.39 (3H, s), 3.49 (3H, s), 3.54 (1H, brt, J = 10.9 Hz), 4.07 (1H, dd, J = 5.2, 10.8 Hz), 4.25 (1H, brdd, J = 3.5, 14.4 Hz), 4.29 (1H, brdd, J = 3.5, 14.4 Hz), 5.16 (1H, dd, J = 5.2, 10.9 Hz), 5.62 (1H, s), 5.91 (1H, brtd, J = 3.5, 16.3 Hz), 5.97 (1H, brd, J = 16.3 Hz), 7.27-7.51 (11H, m), 7.60-7.68 (4H, m); FD-HRMS (m/z) calcd for C40H41O6F3Si35Cl [M+–H]: 737.2313, found: 737.2305.
(R)-4-({(2S,4S,5S)-4-[(E)-3-(Allyloxy)prop-1-en-1-yl]-2-(4-chlorophenyl)-4-methyl-1,3-dioxan-5-yl}-
oxy)-3-bromo-4-methylpentan-1-ol (47). To a solution of 43 (13.0 mg, 0.0210 mmol) in DMF (1 mL) were added NaH (60% in mineral oil, 6.7 mg, 0.17 mmol), allyl bromide (0.018 mL, 0.21 mmol) and Bu4NI (a catalytic amount) at 27 ºC, and the mixture was stirred for 8 h. Then, saturated aq. NH4Cl was added, and the mixture was extracted with hexane several times. The combined organic layers were washed with brine, dried over anhydrous MgSO4, filtered, and concentrated under reduced pressure to give crude allyl ether 46, which was used in the next reaction without purification. To a solution of the above crude 46 in THF (1 mL) was added Bu4NF (1.0 mol/L in THF, 0.063 mL, 0.063 mmol) at 28 ºC, and the mixture was stirred for 1 h. Then, saturated aq. NH4Cl was added, and the mixture was extracted with EtOAc several times. The combined organic layers were washed with brine, dried over anhydrous MgSO4, filtered, and concentrated under reduced pressure. The residue was purified by column chromatography (silica gel, hexane/EtOAc = 3 → 2) to give 47 (8.0 mg, 0.016 mmol, 76% over 2 steps); a colorless oil; [α]D23 +5.46 (c 0.675, CHCl3); IR (neat) ν 3444, 3078, 2978, 2929, 2858, 1602, 1494, 1465, 1419, 1387, 1370, 1300, 1239, 1197, 1130, 1087, 1028, 1016, 988, 921, 816, 796, 757, 723, 666, 639 cm–1; 1H NMR (300MHz, C6D6) δ 0.87 (3H, s), 0.97 (3H, s), 1.42 (3H, s), 1.56 (1H, tdd, J = 3.9, 11.3, 14.6 Hz), 1.92 (1H, dddd, J = 1.8, 5.7, 8.0, 14.6 Hz), 3.45-3.66 (4H, m), 3.83 (1H, td, J = 1.2, 5.4 Hz), 3.86-3.95 (3H, m), 4.00 (1H, dd, J = 1.8, 11.3 Hz), 5.04 (1H, brd, J = 10.4 Hz), 5.25 (1H, brqd, J = 1.5, 17.2 Hz), 5.84 (1H, s), 5.85 (1H, brtdd, J = 5.4, 10.4, 17.2 Hz), 6.07 (1H, td, J = 5.3, 16.3 Hz), 6.22 (1H, brd, J = 16.3 Hz), 7.16 (2H, d, J = 8.6 Hz), 7.45 (2H, d, J = 8.6 Hz); 13C NMR (75 MHz, C6D6) δ 23.4 (CH3), 23.5 (CH3), 29.3 (CH3), 36.5 (CH2), 60.5 (CH2), 62.6 (CH), 68.5 (CH2), 70.6 (CH), 70.7 (CH2), 71.3 (CH2), 78.1 (C), 78.7 (C), 94.9 (CH), 116.5 (CH2), 128.1 (CH × 2), 128.5 (CH × 2), 129.6 (CH), 131.7 (CH), 134.6 (C), 135.3 (CH), 137.8 (C);FD-HRMS (m/z) calcd for C23H33O535Cl79Br [M+H+]: 503.1200, found: 503.1189.
(2S,4S,5S)-4-[(E)-3-(Allyloxy)prop-1-en-1-yl]-5-{[(R)-3-bromo-2-methylpent-4-en-2-yl]oxy}-2-(4-
chlorophenyl)-4-methyl-1,3-dioxane (48). To a solution of 47 (17.5 mg, 0.0347 mmol) and 2-nitrophenylselenocyanate (19.7 mg, 0.0868 mmol) in THF (0.2 mL) was added Bu3P (0.022 mL, 0.089 mmol) at 28 ºC, and the mixture was stirred for 1 h. Then, H2O was added, and the mixture was extracted with hexane several times. The combined organic layers were washed with brine, dried over anhydrous MgSO4, filtered, and concentrated under reduced pressure. The residue was purified by column chromatography (silica gel, hexane/EtOAc = 20) to give a selenide, which was immediately used in the next reaction. To a solution of the selenide in THF (1 mL) was added 30% aq. H2O2 (0.1 mL) at 28 ºC, and the mixture was stirred for 12 h in the dark. Then, H2O was added, and the mixture was extracted with hexane several times. The combined organic layers were washed with saturated aq. Na2SO3 and then with brine, dried over anhydrous MgSO4, filtered, and concentrated under reduced pressure. The residue was purified by column chromatography (silica gel, hexane/benzene = 3 → hexane/EtOAc = 3) to give 48 (14.7 mg, 0.0303 mmol, 87% over 2 steps); a pale yellow oil; [α]D22 +21.0 (c 0.505, CHCl3); IR (neat) ν 3083, 2979, 2927, 2855, 1494, 1462, 1417, 1386, 1368, 1300, 1282, 1238, 1225, 1198, 1171, 1131, 1087, 1016, 988, 926, 883, 815, 722, 666 cm–1; 1H NMR (300MHz, C6D6) δ 0.84 (3H, s), 0.91 (3H, s), 1.46 (3H, s), 3.56 (1H, dd, J = 4.7, 10.6 Hz), 3.71 (1H, t, J = 10.7 Hz), 3.85 (2H, td, J = 1.4, 5.4 Hz), 3.91-4.00 (3H, m), 4.04 (1H, d, J = 10.0 Hz), 4.74 (1H, brdd, J = 1.2, 10.0 Hz), 4.83 (1H, brd, J = 17.0 Hz), 5.05 (1H, brqd, J = 1.5, 10.4 Hz), 5.28 (1H, brqd, J = 1.7, 17.2 Hz), 5.76-5.93 (2H, m), 5.87 (1H, s), 6.13 (1H, td, J = 5.4, 16.3 Hz), 6.31 (1H, td, J = 1.4, 16.3 Hz), 7.16 (2H, d, J = 8.5 Hz), 7.46 (2H, d, J = 8.5 Hz); 13C NMR (75 MHz, C6D6) δ 22.3 (CH3), 24.9 (CH3), 29.5 (CH3), 65.2 (CH), 68.6 (CH2), 70.4 (CH), 70.7 (CH2), 71.3 (CH2), 77.1 (C), 78.8 (C), 95.0 (CH), 116.4 (CH2), 117.9 (CH2), 128.1 (CH × 2), 128.5 (CH × 2), 129.7 (CH), 131.7 (CH), 134.6 (C), 135.4 (CH), 136.1 (C), 137.8 (C); FD-HRMS (m/z) calcd for C23H29O435Cl79Br [M+–H]: 483.0938, found: 483.0910.
(2S,3S,E)-6-(Allyloxy)-2-{[(R)-3-bromo-2-methylpent-4-en-2-yl]oxy}-3-methylhex-4-ene-1,3-diol (10). To a solution of 48 (57.5 mg, 0.118 mmol) in EtOH (4 mL) was added 2 mol/L aq. HCl (2 mL) at 25 ºC, and the mixture was stirred for 6 h in the dark. Then, NaHCO3 was added, and the mixture was extracted with EtOAc several times. The combined organic layers were washed with brine, dried over anhydrous MgSO4, filtered, and concentrated under reduced pressure. The residue was purified by column chromatography (silica gel, hexane/EtOAc = 2) to give 10 (39.1 mg, 0.108 mmol, 92%); a colorless oil; [α]D21 +49 (c 0.22, CHCl3); IR (neat) ν 3383, 3083, 2979, 2934, 2852, 1462, 1453, 1417, 1386, 1367, 1282, 1223, 1172, 1127, 1066, 987, 928, 878, 784, 721 cm–1; 1H NMR (300MHz, C6D6) δ 0.99 (3H, s), 1.01 (3H, s), 1.30 (3H, s), 2.02 (1H, brs, OH), 2.59 (1H, brs, OH), 3.29 (1H, dd, J = 3.5, 5.6 Hz), 3.50-3.67 (2H, m), 3.79-3.84 (2H, m), 3.86 (2H, d, J = 4.4 Hz), 4.16 (1H, d, J = 10.0 Hz), 4.73 (1H, brdd, J = 1.2, 9.8 Hz), 4.83 (1H, brd, J = 16.9 Hz), 5.04 (1H, brd, J = 10.4 Hz), 5.26 (1H, brqd, J = 1.7, 17.2 Hz), 5.77-6.05 (4H, m); 13C NMR (75 MHz, C6D6) δ 22.6 (CH3), 24.8 (CH3), 26.2 (CH3), 64.0 (CH2), 66.4 (CH2), 70.5 (CH2), 71.2 (CH2), 75.6 (C), 77.09 (CH), 77.12 (C), 116.4 (CH2), 118.0 (CH2), 126.1 (CH), 135.4 (CH), 136.3 (CH), 136.9 (CH); FD-HRMS (m/z) calcd for C16H28O479Br [M+H+]: 363.1171, found: 363.1195.
(2S,3S,6R)-6-Bromo-2-(hydroxymethyl)-3,7,7-trimethyl-2,3,6,7-tetrahydrooxepin-3-ol (49). To a solution of 10 (12.6 mg, 0.0347 mmol) in xylenes (15 mL) was added the second generation Hoveyda-Grubbs catalyst (6.5 mg, 0.010 mmol), and the mixture was refluxed for 1 h in the dark. Then, the mixture was cooled to 25 ºC and concentrated under reduced pressure. The residue was purified by column chromatography (silica gel, hexane/EtOAc = 2) to give 49 (3.2 mg, 0.012 mmol, 35%); a colorless amorphous; [α]D22 –100 (c 0.060, CHCl3); IR (neat) ν 3450, 3026, 2974, 2922, 2850, 1462, 1453, 1377, 1264, 1230, 1170, 1137, 1090, 1060, 1034, 969, 843, 795, 720 cm–1; 1H NMR (400MHz, CDCl3) δ 1.23 (3H, s), 1.46 (6H, s), 2.00-2.11 (1H, m, OH), 2.93 (1H, brs, OH), 3.67-3.88 (3H, m), 4.60 (1H, d, J = 6.0 Hz), 5.53 (1H, d, J = 11.8 Hz), 5.85 (1H, dd, J = 6.0, 11.8 Hz); 13C NMR (100 MHz, CDCl3) δ 24.0 (CH3), 24.2 (CH3), 28.3 (CH3), 56.1 (CH), 61.3 (CH2), 74.4 (C), 76.6 (CH), 78.9 (C), 128.4 (CH), 139.2 (CH); Under MS measurement conditions, a significant elimination of bromine atom of 49 occurred, and therefore HRMS of [M+] or [M+H+] peak was not obtained.
(2S,3S,6R)-6-Bromo-2-(hydroxymethyl)-3,7,7-trimethyloxepan-3-ol (1). To a solution of 49 (2.3 mg, 0.0087 mmol) in MeCN (3 mL) was added excess amount of NBSH and KHCO3 at 23 ºC, and the mixture was stirred for 3 days in the dark. Then, NaHCO3 was added, and the mixture was extracted with EtOAc several times. The combined organic layers were washed with brine, dried over anhydrous MgSO4, filtered, and concentrated under reduced pressure. The residue was purified by column chromatography (silica gel, hexane/EtOAc = 2) and HPLC (JAIGEL 0A2000-10, 250 mm × 10 mm ID, hexane/EtOAc = 4; flow rate: 3.0 mL/min, RI detection) to give 1 (≤ 1.0 mg, ≤43%); a colorless amorphous; [α]D23 +6.3 (c 0.10, CHCl3); IR (KBr) ν 3422, 2996, 2986, 2975, 2959, 2941, 2926, 2900, 2854, 1461, 1383, 1367, 1343, 1333, 1320, 1294, 1216, 1174, 1158, 1139, 1102, 1076, 1034, 1025, 970, 946, 795, 712, 599, 533 cm–1; 1H NMR (300MHz, CDCl3) δ 1.12 (3H, s), 1.44 (3H, s), 1.48 (3H, s), 1.54 (1H, brddd, J = 3.2, 13.0, 14.7 Hz), 1.75 (brddd, J= 2.3, 5.2, 14.7 Hz), 1.91 (1H, brs, OH), 2.06 (1H, brddd, J = 3.2, 5.2, 14.8 Hz), 2.37 (1H, brdddd, J = 2.3, 11.0, 13.0, 14.8 Hz), 3.04 (1H, brs, OH), 3.49 (1H, brt, J = 5.9 Hz), 3.65 (2H, m), 3.93 (1H, d, J = 11.0 Hz); 13C NMR (75 MHz, CDCl3) δ 24.0 (CH3), 25.1 (CH3), 25.6 (CH3), 30.3 (CH2), 44.2 (CH2), 58.2 (CH), 61.4 (CH2), 71.5 (C), 75.7 (CH), 78.7 (C); ESI-HRMS (m/z) calcd for C10H19O379BrNa [M+Na+]: 289.04098, found: 289.04105.
ACKNOWLEDGEMENTS
We thank Dr. Eri Fukushi and Mr. Yusuke Takata (GC-MS and NMR Laboratory, Graduate School of Agriculture, Hokkaido University) as well as Ms. Seiko Oka and Ms. Ai Tokumitsu (Equipment Management Center, Creative Research Institution, Hokkaido University) for the measurements of mass spectra. This work was supported by Grants-in-Aid for Scientific Research from MEXT, Japan.
References
1. M. L. Ciavatta, S. Wahidulla, L. D'Souza, G. Scognamiglio, and G. Cimino, Tetrahedron, 2001, 57, 617. CrossRef
2. B. S. Underwood, J. Tanuwidjaja, S.-S. Ng, and T. F. Jamison, Tetrahedron, 2013, 69, 5205. CrossRef
3. A. V. K. Prasad and Y. Shimizu, J. Am. Chem. Soc., 1989, 111, 6476; CrossRef M. Satake, M. Murata, and T. Yasumoto, J. Am. Chem. Soc., 1993, 115, 361; CrossRef A. Morohashi, M. Satake, and T. Yasumoto, Tetrahedron Lett., 1999, 40, 97; CrossRef A. J. Bourdelais, H. M. Jacocks, J. L. C. Wright, P. M. Bigwarfe, Jr., and D. G. Baden, J. Nat. Prod., 2005, 68, 2; CrossRef H. Fuwa, M. Ebine, A. J. Bourdelais, D. G. Baden, and M. Sasaki, J. Am. Chem. Soc., 2006, 128, 16989. CrossRef
4. T. Yasumoto and M. Murata, Chem. Rev., 1993, 93, 1897; CrossRef Y. Shimizu, Chem. Rev., 1993, 93, 1685; CrossRef M. Murata and T. Yasumoto, Nat. Prod. Rep., 2000, 17, 293; CrossRef T. Yasumoto, Chem. Rec., 2001, 1, 228; CrossRef A. H. Daranas, M. Norte, and J. J. Fernández, Toxicon, 2001, 39, 1101. CrossRef
5. K. Fujiwara, Y. Hirose, D. Sato, H. Kawai, and T. Suzuki, Tetrahedron Lett., 2010, 51, 4263. CrossRef
6. K. Fujiwara, K. Tanaka, Y. Katagiri, H. Kawai, and T. Suzuki, Tetrahedron Lett., 2010, 51, 4543. CrossRef
7. F. Bravo, F. E. McDonald, W. A. Neiwert, and K. I. Hardcastle, Org. Lett., 2004, 6, 4487. CrossRef
8. Y. Morimoto, Y. Nishikawa, and M. Takaishi, J. Am. Chem. Soc., 2005, 127, 5806; CrossRef Y. Morimoto, H. Yata, and Y. Nishikawa, Angew. Chem. Int. Ed., 2007, 46, 6481. CrossRef
9. R. E. Ireland, R. H. Muller, and A. K. Willard, J. Am. Chem. Soc., 1976, 98, 2868; CrossRef C. M. McFarland and M. C. McIntosh, 'The Claisen Rearrangement,' ed. by M. Hiersemann and U. Nubbemeyer, Wiley-VCH, Weinheim, 2007, pp. 117-210. CrossRef
10. S. Han and S. Chang, 'Handbook of Metathesis,' Vol. 2, ed. by R. H. Grubbs, Wiley-VCH, Weinheim, 2003, pp. 5-127. CrossRef
11. T. R. Hoye, C. S. Jeffrey, M. A. Tennakoon, J. Wang, and H. Zhao, J. Am. Chem. Soc., 2004, 126, 10210. CrossRef
12. K. Fujiwara, A. Goto, D. Sato, H. Kawai, and T. Suzuki, Tetrahedron Lett., 2005, 46, 3465; CrossRef D. Sato, K. Fujiwara, H. Kawai, and T. Suzuki, Tetrahedron Lett., 2008, 49, 1514; CrossRef K. Fujiwara, N. Kawamura, H. Kawai, and T. Suzuki, Tetrahedron Lett., 2009, 50, 1236; CrossRef K. Nogoshi, D. Domon, K. Fujiwara, N. Kawamura, R. Katoono, H. Kawai, and T. Suzuki, Tetrahedron Lett., 2013, 54, 676; CrossRef D. Domon, K. Fujiwara, N. Kawamura, R. Katoono, H. Kawai, and T. Suzuki, Nat. Prod. Commun., 2013, 8, 929. See also refs. 5 and 6.
13. A number of electrophilic or nucleophilic reaction conditions were examined with 15, 16, and their derivatives for the installation of bromo group at C3. However, in most cases, significant decomposition of the starting materials was observed, and, in some cases, small amounts of ring-contracted byproducts, which would be attributable to the unnecessary participation of the oxygen atom of the oxepane ring under the reaction conditions, were obtained.
14. M. Bandini, P. G. Cozzi, S. Licciulli, and A. Umani-Ronchi, Synthesis, 2004, 409; J. I. Yun, H. R. Kim, S. K. Kim, D. Kim, and J. Lee, Tetrahedron, 2012, 68, 1177. CrossRef
15. C. Hubschwerlen, J.-L. Specklin, and J. Higelin, Org. Synth., 1995, 72, 1. CrossRef
16. D. B. Dess and J. C. Martin, J. Org. Chem., 1983, 48, 4155; CrossRef D. B. Dess and J. C. Martin, J. Am. Chem. Soc., 1991, 113, 7277. CrossRef
17. M. J. Fan, G.-Q. Li, and Y.-M. Liang Tetrahedron, 2006, 62, 6782.
18. S. Pikul, M. Kozlowska, and J. Jurczak, Tetrahedron Lett., 1987, 28, 2627; CrossRef T. Yamanoi, T. Akiyama, E. Ishida, H. Abe, M. Amemiya, and T. Inazu, Chem. Lett., 1989, 335; CrossRef A. Dondoni, G. Fantin, M. Fogagnolo, A. Medici, and P. Pedrini, J. Org. Chem., 1989, 54, 702; CrossRef H. Chikashita, T. Nikaya, H. Uemura, and H. Itoh, Bull. Chem. Soc. Jpn., 1989, 62, 2121. CrossRef
19. A. L. Gemal and J.-L. Luche, J. Am. Chem. Soc., 1981, 103, 5454. CrossRef
20. I. Ohtani, T. Kusumi, Y. Kashman, and H. Kakisawa, J. Am. Chem. Soc., 1991, 113, 4092. CrossRef
21. Y. Hayashi, J. Yamaguchi, and M. Shoji, Tetrahedron, 2002, 58, 9839. CrossRef
22. A. J. Mancuso, S.-L. Huang, and D. Swern, J. Org. Chem., 1978, 43, 2480. CrossRef
23. J. Hooz and S. S. H. Gilani, Can. J. Chem., 1968, 46, 86. CrossRef
24. T. Tsunoda, M. Suzuki, and R. Noyori, Tetrahedron Lett., 1980, 21, 1367.
25. H. Knust and R. W. Hoffmann, Helv. Chim. Acta, 2003, 86, 1871. CrossRef
26. J. R. Parikh and W. v. E. Doering, J. Am. Chem. Soc., 1967, 89, 5505. CrossRef
27. B. O. Lindgren, T. Nilsson, S. Huseby, Ø. Mikalsen, K. Leander, and C.-G. Swahn, Acta Chem. Scand., 1973, 27, 888; G. A. Kraus and B. Roth, J. Org. Chem., 1980, 45, 4825.
28. N. Hashimoto, T. Aoyama, and T. Shioiri, Chem. Pharm. Bull., 1981, 29, 1457. CrossRef
29. P. A. Grieco, S. Gilman, and M. Nishizawa, J. Org. Chem., 1976, 41, 1485. CrossRef
30. S. B. Garber, J. S. Kingsbury, B. L. Gray, and A. H. Hoveyda, J. Am. Chem. Soc., 2000, 122, 8168. CrossRef
31. P. Schwab, R. H. Grubbs, and J. W. Ziller, J. Am. Chem. Soc., 1996, 118, 100. CrossRef
32. A. G. Myers, B. Zheng, and M. Movassaghi, J. Org. Chem., 1997, 62, 7507; CrossRef B. J. Marsh and D. R. Carbery, J. Org. Chem., 2009, 74, 3186. CrossRef