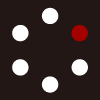
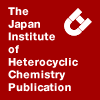
HETEROCYCLES
An International Journal for Reviews and Communications in Heterocyclic ChemistryWeb Edition ISSN: 1881-0942
Published online by The Japan Institute of Heterocyclic Chemistry
e-Journal
Full Text HTML
Received, 5th December, 2014, Accepted, 19th January, 2015, Published online, 20th January, 2015.
DOI: 10.3987/COM-14-13145
■ Synthesis and Photophysical Properties of Some Dithienylbenzo[c]thiophene Derivatives
Maurizio D'Auria,* Ambra Guarnaccio, Rocco Racioppi, Antonio Santagata, and Roberto Teghil
Departament of Science, Università della Basilicata, viale dell'Ateneo Lucano 10, 00137, Italy
Abstract
The synthesis of a new fullerene - dithienylbenzo[c]thiophene (DTBT) - fullerene triad has been reported. The synthetic scheme provides the synthesis of DTBT unit, a Sonogashira reaction to introduce two acetylenic groups and the coupling with fullerene. The product showed an absorption at 485 nm and a fluorescence band at 575 nm. Treatment of 5-(3-(5-ethynyl-2-thienyl)benzo-[c]-1-thienyl)thiophene-2-carbaldehyde with LHDMS gave a dimeric product that showed an absorption at 475 nm and a fluorescence band at 595 nm. Furthermore, 5-(3-(2-thienyl)benzo[c]-1-thienyl)thiophene-2-carbaldehyde was treated with hydrazine to give the corresponding adduct that showed an absorption at 480 nm and a fluorescence band at 605 nm.INTRODUCTION
Past years have shown a rapid development of researches devoted to the synthesis of new heterocyclic compounds to be used for optoelectronic applications. The main applications for these new semiconductor materials are organic thin film transistors (OTFTs), light-emitting diodes (OLEDs), photovoltaic cells, sensors, and radio frequency identification (RF-ID) tags. The use of organic materials can allow to bypass some expensive processes necessary for silicon-based materials, such as lithography and vacuum deposition steps.
Several semiconductors have been synthesized and they belonged to different classes of compounds.1 A significant part of these studies has been performed on thiophene derivatives.2 In this class of compounds we focused our attention on benzo[c]thiophene derivatives. The first example of a fused thiophene-based material was reported by Wudl in 1984.3 Poly(isothianaphthene) (PITN) was synthesized by electrochemical polymerization of benzo[c]thiophene. Both theoretical and experimental investigations4-6 showed that fused benzene in benzo[c]thiophene increases the quinoid character of the electronic ground state, causing a band gap of ~ 1.0 eV, lower than that of polythiophene (2.0 eV).7,8 Alkyl and alkoxy substituted PITNs were also developed.9-11 Since 1992 the synthesis and the electrochemical behavior of 1,3-dithienylbenzo[c]thiophene have been reported.12-14 In addition, Cava and coworkers have outlined detailed synthetic studies of 1,3-dithienylbenzo[c]thiophene analogues.15 Bäuerle and coworkers developed a series of end-capped benzo[c]thiophene-based oligomers that showed a significant red shift in their absorption spectra.16 Alkyl substituted terthiophenes incorporating a central benzo[c]thiophene unit were successfully dimerized to form hexathiophenes.17 Oxidative coupling of noncapped terthiophenes yielded higher oligomers.15 Application of these materials as donor and fullerene PCBM as acceptor in bulk heterojunction solar cells was also investigated.18
Recently, we reported a communication on the synthesis of a new dithienylbenzo[c]thiophene derivative.19 In this paper, we want to report all the results we obtained in this field.
RESULTS AND DISCUSSION
The main building block in our approach was 1,3-dithienylisothianaphthene (DTBT). It was prepared in 37% overall yield (Scheme 1).20 2-Thienylmagnesium bromide (2 mol equivalents) reacted with ortho-phthalaldehyde (1) to give the secondary diol 2 with 76% yield. The treatment of the diol in presence of pyridinium chlorochromate (PCC)21 led to the diketone 3 with 49% of yield.
The 1,4-diketone was transformed in the corresponding thiophene through treatment with Lawesson’s reagent (LR) to afford desired 1,3-bis(2-thienyl)benzo[c]thiophene (4).
1,3-Bis(5-bromo-2-thienyl)benzo[c]thiophene (5) was synthesized according to the standard bromination of 4 with N-bromosuccinimide (NBS) as reported by Mitschke and Bäuerle (Scheme 2).16 Although, as it was reported by Kisselev and Thelakkat,22 a 1:1 CHCl3/AcOH solvent mixture has been used. In that case, the reaction was carried out at -40 °C while we tried to synthesize 5 in less drastic conditions of temperature (cooled in an ice bath). Under these conditions, small amounts of the tetrabrominated by-product were obtained. This product resulted hard to separate by purification from the product of our interest. The use of DMF as solvent gave better results. Furthermore, better results were obtained working always at 0 °C and in the dark as reported by Jung.23 Proceeding in this way, we obtained product of interest 5 in good yield and the tetrabrominated byproduct was not observed at all (Scheme 2).
1,3-Bis(5-bromo-2-thienyl)benzo[c]thiophene (5) was used as substrate in a cross-coupling reaction to realize an acetylene coupling: this way allowed us to obtain 1,3-bis(5-ethynyl-2- thienyl)benzo[c]thiophene (6) (Scheme 2).
The reaction was attempted by using several different reaction conditions. Pd(OAc)2/CuI in triethylamine/THF, Pd(PPh3)4/CuI in Et3N/THF, PdCl2(PPh3)2/CuI in Et3N/THF, PdCl2(PPh3)2/CuI in piperidine/THF, and PdCl2(PPh3)2/CuI in piperidine/toluene. The best reaction conditions were found using four equivalents of trimethylsilylacetylene with PdCl2(PPh3)2 (4% mol) and CuI (6% mol) as catalysts, piperidine as base, and toluene as solvent. We obtained the compound 6 in a two-step synthetic pathway. After Sonogashira coupling we obtained the corresponding trimethylsilyl derivative. The acetylene protected functionality has been deprotected in standard basic condition in presence of K2CO3 obtaining desired compound 6 with 80% yield.
In organic solar cells blending of PCBM ([6,6]-phenyl-C61-butyric acid methyl ester) with regioregular poly(3-hexylthiophene) (P3HT) gave power conversion efficiencies up to 3.5% after annealing of the devices.24 Higher efficiencies of more than 5% using P3HT and PCBM in bulk heterojunction tandem solar cells have been recently reported.25,26 On the basis of these positive results, various attempts have been made to covalently link C60 to an oligothiophene backbone in order to prepare D-A-based materials and tuning their electronic properties for photovoltaic devices.27-30 The attachment of fullerene units prevented large scale phase separation in thin films. Several examples of oligothiophenes linked to C60 have been reported.31-45
We decided to do a one-pot ethynylation that allows the attachment of fullerene C60 moiety to both terminal alkynes as reported in Scheme 3.
Ethynylated fullerenes can be prepared through the formation of lithium acetylide and the following addition to fullerene as first reported by Komatsu.46 Lafleur-Lambert reported a coupling of a similar substrate with fullerene.47 We modified the ethynylation experimental procedure. We decided to change solvent because in THF fullerene showed a poor solubility. We found that by using chlorobenzene as reaction solvent at room temperature, we can better dissolve the fullerene (in fact solubility of C60 in THF is 0.04 g/L while in chlorobenzene is 6.35 g/L). In this way, we obtained desired product 7 with 18% yield over last two steps.
With the aim to use the above described approach to the synthesis of extended π systems we synthesized some asymmetric dithienybenzo[c]thiophenes. The approach is described in Scheme 4.
Vilsmeier-Haack formylation of 4 gave the aldehyde 8 where only one thienyl ring showed the presence of the carbonyl group. Bromination of the other thienyl ring gave the bromoaldehyde 9. Ethynylation with trimethylsilylacetylene in the presence of PdCl2(PPh3)2 and CuI followed by deprotection of the alkyne gave 11. Compound 11 was treated with LHDMS to give the corresponding dimer 12 (Scheme 5). It is noteworthy the anomalous reactivity of 11. In fact, it did not react with fullerene if the lithium acetylide of 11 was treated with this reagent, and did not give an addition reaction to the carbonyl group of another molecule of the substrate: the only product we observed was the dimer 12.
A second dimer was obtained by coupling the aldehyde 8 with hydrazine as reported for the synthesis of polymeric materials.48 The reaction of 8 with hydrazine gave the corresponding adduct 13 (Scheme 6).
In Figure 1 is reported the UV-Vis absorption spectrum of a solution of DTBT-(C60)2 (7) in CHCl3. Two absorption bands at 310 and 485 nm could be observed. The absorption band at 310 nm can be attributed to a π-π* transition to the S1 state of the π-conjugated system, while the absorption band at 485 nm could be due to a π-π* transition into S2 state. The presence of the fullerene units induces a marked red shift from 435 nm (DTBT) to 485 nm (DTBT-(C60)2).
Two different fluorescence bands were observed pumping the DTBT-(C60)2 system at the two different wavelengths corresponding at each maximum absorption band present in the UV-Vis spectrum. Pumping the system at 320 nm, a fluorescence band centered around 390 nm was observed, while pumping at 480 nm a fluorescence band centered around 575 nm was detected. In this case, a pronounced shoulder at 610 nm was observed (Figure 2).
A Stokes shift of 90 nm was determined. DTBT showed an emission maximum at 532 nm when irradiated at 435 nm. The presence of the fullerene units induces an evident hypsochromic shift.
The HOMO of DTBT-(C60)2 was found at -0.17177 H and it is mainly localized on the dithienyl-benzo[c]thiophene part of the molecule. Furthermore, the LUMO was found at 0.11032 H and was localized on the fullerene (Figure 3). The calculations were performed at DFT/B3LYP/6-31G+(d,p) level on Gaussian 09.49 These calculations shows that the HOMO →LUMO transition is an electron transfer process.
Dimer 12 showed the UV-Vis spectrum reported in Figure 4. The main absorption is a band centered at 475 nm, while another absorption band has a maximum at 320 nm. The fluorescence emission is red shifted in comparison to that showed by 7 (Figure 5). Compound 12 showed an emission centered at 595 nm with a shift of 63 nm, if compared with 4, and 20 nm, if compared with 7. A Stokes shift of 120 nm was observed.
The HOMO of 12 was found at -0.20775 H and, while the LUMO was found at -0.09639 H (Figure 6).
The calculations were performed at DFT/B3LYP/6-31G+(d,p) level on Gaussian 09.49 These calculations shows that the HOMO →LUMO transition is a π,π∗ transition. The UV-Vis spectrum of the dimer 13 was recorded in DMSO and 1,1,2-trichloroethylene (Figure 7). In both cases, absorption bands were observed at 340 and 480 nm. Both the absorption bands induced a fluorescence with very near maxima (Figure 8). When the compound 13 was excited at 480 nm a low fluorescence band was observed at 605 nm. Excitation at 340 nm gave an intense fluorescence band at 600 nm. A Stokes shift of 120 nm was present also in this case.
The HOMO of 13 was found at -0.20049 H and, while the LUMO was found at -0.08817 H (Figure 9). The calculations were performed at DFT/B3LYP/6-31G+(d,p) level on Gaussian 09.49 These calculations shows that the HOMO →LUMO transition is a π,π∗ transition.
In this article we have shown that dithienylbenzo[c]thiophene units can be used in the synthesis of new oligothiophene derivatives. Compound 7 has the correct structure to be a donor-acceptor triad useful in photovoltaic apparatus. Nevertheless, compounds 12 and 13 showed interesting photophysical properties with emissions in the range 595-605 nm (yellow emission) and Stokes shifts of 120-125 nm.
EXPERIMENTAL
Dry solvents have been prepared by standard procedures. Chloroform (CHCl3) was purified by distillation over calcium hydride. Dry THF, diethyl ether and toluene were refluxed over sodium benzophenone and under argon until a dark blue solution was obtained and then distilled immediately before use under nitrogen or argon. All other chemicals have been purchased from Sigma Aldrich and used as received. All reaction involving organolithium reagents were carried out by using standard techniques for the manipulation of air- and water-sensitive compounds. All of the experiments were carried out under a nitrogen atmosphere unless otherwise stated. Liquids and solvents were transferred via syringe. The progression of all of the reactions was monitored by TLC method. Analytical TLC was done on Macherey-Nagel Alugram Sil G/UV254 alumina coated plates. Column chromatography was carried out on silica gel Merk 60 (70-230 mesh). Spectra were obtained as follows: 1H NMR and 13C NMR spectra were recorded with a Varian INOVA 400 (400 MHz) spectrometer and a Varian INOVA 500 in CDCl3 using tetramethylsilane as an internal standard. Multiplicities are reported as follows: singlet (s), doublet (d), triplet (t), quartet (q), multiplet (m). Gas-chromatography coupled with mass spectrometry analysis were performed using a Hewlett Packard GC/MS 6890-5973 equipments.
1,2-Phenylenebis(2-thienylmethanol) (2).
In a flask fitted with a reflux condenser magnesium turnings (280 mg, 11.67 mmol), I2 (10 mg; catalytic amount) and 40 mL of dry THF were added. Stirring the solution under argon, the commercially available 2-bromothiophene (900 µL; 1.5 g; 9.30 mmol) in 10 mL of dry THF was added dropwise until the exothermic reaction started. The reaction mixture was stirred at room temperature to complete the formation of the Grignard reagent and after 1.5 h the reagent was ready to be used for the next synthetic step. The Grignard reagent was transferred via cannula dropwise at 0 °C under argon to a solution of the commercial ortho-phthalaldehyde (1.99 g; 14.84 mmol) in 40 mL of dry THF. After addition the mixture was allowed to warm up to room temperature and stirred for 3 h. The reaction was quenched using 20 mL of a saturated ammonium chloride aqueous solution followed by extractions with 3 x 10 mL of EtOAc. The organic phase was then treated with Na2SO4 and the solvent removed under reduced pressure. The crude was then purified by column chromatography over silica gel using petroleum ether/Et2O (6:4) eluent to give desired product (2) as mixture of “anti” and “sin” diols (3.39 g; 11.22 mmol, 76% yield). 1H NMR (400 MHz, CDCl3) δ: 7.44 (m, 2 H), 7.29 (m, 4 H), 7.17 (m, 2H), 6.85 (m, 2 H), 6.695 (m, 2H), and 6.14 ppm (s, 2 H). 13C NMR (100 MHz, CDCl3) δ: 147.27, 140.58, 128.78, 127.92, 127.07, 125.68, 125.31, and 70.04 ppm. Anal. Calcd for C16H14O2S2 (302.4): C, 63.55; H, 4.67; S, 21.21. Found: C, 63,44; H, 4.70; S, 21.18%.
1,2-Di-(2-thienoyl)benzene (3).
A round bottom flask was charged with diol 2 (3.39 g; 11.22 mmol) and pyridinium chlorochromate (PCC) (4.50 g; 22.44 mmol) with 60 mL of dry CH2Cl2. Under argon atmosphere and at room temperature the mixture was stirred for 5 h. Then the reaction mixture was quenched adding 30 mL of Et2O and the crude was filtered over silica using CHCl3. After filtration the crude mixture was purified by column chromatography over silica gel and petroleum ether/EtOAc (8:2) as eluent giving the desired compound 3 (1.62 g: 5.44 mmol; 49% yield) as pale yellow crystals. 1H NMR (400 MHz, CDCl3) δ: 7.73 (m, 2 H), 7.64 (m, 4H), 7.46 (m, 2 H), and 7.06 ppm (m, 2 H). 13C NMR (100 MHz, CDCl3) δ: 188.50, 144.28, 139.55, 135.36, 135.18, 130.83, 129.44, and 128.23 ppm. GC-MS (EI) m/z: 298.0 (M+). FT-IR (KBr pellet) νmax cm-1: 1620, 1585, 1570, 1510, 1410. Anal. Calcd for C16H10O2S2 (298.4): C, 64.40; H, 3.38; S, 21.49. Found: C, 64.45; H, 3.30; S, 21.58%.
1,3-Dithienylisothianaphthene (4).
A mixture of compound 3 (31 mg, 0.10 mmol), Lawesson’s reagent (LR) (46 mg, 0.11 mmol), and 3 mL of dry CH2Cl2 was stirred for 4 h at room temperature. After evaporation of the solvent, EtOH (4 mL) was added into the residual red oil and the mixture was heated for 1 h at 40-50 °C. Then, the solvent was evaporated under reduced pressure and the crude product was purified by chromatography on silica gel using petroleum ether as eluent. The final product 4 (29 mg, 99% yield) was obtained as an orange powder. 1H NMR (400 MHz, CDCl3) δ: 7.20 (m, 4 H), 7.15 (m, 3 H), and 7.00 ppm (m, 3 H). 13C NMR (100 MHz, CDCl3) δ: 135.57, 135.24, 127.86, 126.46, 125.57, 125.52, 124.76, and 121.49 ppm. GC-MS (EI) m/z: 298.0 (M+). UV-Vis (CHCl3) λmax nm: 435. FT-IR (KBr pellet) νmax cm-1: 1530, 1216, 1182, 841, 738. Anal. Calcd for C16H10S3 (298.5): C, 64.39; H, 3.38; S, 32.23. Found: C, 64.45; H, 3.30; S, 32.25%.
1,3-Bis(5-bromo-2-thienyl)benzo[c]thiophene (5).
Under argon atmosphere, a solution of N-bromosuccinimide (NBS) (238 mg, 1.34 mmol) in 5 mL of dry DMF was added dropwise to a solution of compound 4 (200 mg, 0.67 mmol) in 10 mL of dry DMF at 0 °C. The resulting mixture was stirred for 2 h at 0 °C, then the solution was warmed to room temperature and stirred overnight. The orange mixture obtained was poured into water. The precipitate was filtered off and re-crystallized from CHCl3/n-hexane (10 mL/15 mL). The product 5 was obtained as reddish needle-like crystals (271 mg, 89% yield). 1H NMR (400 MHz, CDCl3) δ: 7.87 (dd, J = 4 Hz, 2 H), 7.15 (d, J = 4 Hz, 2 H), and 7.07 ppm (m, 4 H). 13C NMR (100 MHz, CDCl3) δ: 143.45, 136.99, 135.64, 130.95, 126.25, 125.50, 121.46, and 112.59 ppm. GC-MS (EI) m/z: 455.8 (M+). UV-Vis (CHCl3) λmax nm: 440. FT-IR (KBr pellet) νmax cm-1: 3062, 1529, 1489, 1433, 1190, 969, 788, 778, 734, 699, 582. Anal. Calcd for C16H8Br2S3 (456.2): C, 42.12; H, 1.77; S, 22.00. Found: C, 42.23; H, 1.65; S, 21.12%.
1,3-Bis(5-ethynyl-2-thienyl)benzo[c]thiophene (6).
A round bottom flask equipped with a magnetic stir bar was charged with compound 5 (60 mg, 0.13 mmol), dry toluene and piperidine (4 mL: 1 mL) under argon atmosphere. Then PdCl2(PPh3)2 (4.4 mg, 0.0065 mmol), CuI (3 mg, 0.013 mmol) and TMSA (74 µL, 0.52 mmol) were added. The reaction mixture was refluxed for 30 min. The crude was filtered and the orange crystals (64 mg, 99% yield) washed with fresh toluene were characterized by 1H NMR (400 MHz, CDCl3) δ: 7.92 (dd, J = 4 Hz, 2 H), 7.22 (d, J = 4 Hz, 2 H), 7.15 (m, 4H), and 0.27 ppm (s, 18 H). 13C NMR (100 MHz, CDCl3) δ: 137.18, 135.71, 133.58, 126.70, 125.64, 125.24, 123.17, 121.78, 101.20, 97.50, and 0.10 ppm. To the orange compound (64 mg, 0.13 mmol) in a mixture 1:1 of MeOH/THF (4 mL/4 mL) an excess of K2CO3 (100 mg, 0.72 mmol) was added. After 2 h stirring at room temperature and without inert atmosphere the solvent was removed under reduced pressure. The crude was purified by chromatography on silica gel with petroleum ether as eluent to afford compound 7 (28 mg, 62% yield and 80% over 2 steps) as a dark red amorphous powder. 1H NMR (500 MHz, CDCl3) δ: 7.94 (dd, J = 5 Hz, 2 H), 7.35 (d, J = 5 Hz, 2 H), 7.25 (m, 4 H), and 3.49 ppm (s, 2 H). 13C NMR (125 MHz, CDCl3) δ: 137.23, 135.67, 133.90, 126.44, 125.77, 125.02, 121.85, 121.47, 104.88, and 82.94 ppm. Anal. Calcd for C20H10S3 (346.5): C, 69.33; H, 2.91; S, 27.76. Found: C, 69.30; H, 2.99; S, 27.71%.
1,3-Bis(5-ethynyl-2-thienyl)benzo[c]thiophene-di(hydrofullerene) (7).
A round bottom flask was charged with compound 6 (28 mg, 0.08 mmol), C60 (233 mg, 0.32 mmol) and dry chlorobenzene (32 mL). Then LiHMDS 1.0 M/THF (350 µL, 0.35 mmol) was added to the reddish-brown solution. After the addition of LiHMDS, the reaction mixture was stirred for 1 h and quenched with trifluoroacetic acid (805 µL, 10.5 mmol). The dark violet reaction mixture was stirred at room temperature for 24 h before the first filtration performed under vacuum to eliminate the excess of unreacted C60. After removal of the solvent the crude product was diluted in CH2Cl2, filtered under vacuum to remove residual unreacted C60 and the solvent was removed in reduced pressure. The crude product purified by column chromatography on silica gel with 50% toluene/EtOAc as eluent to afford compound 7 (19 mg, 18% yield) as a dark orange amorphous powder. 1H NMR (400 MHz, CDCl3) δ: 8.04 (m, 2 H), 7.93 (m, 2 H), 7.70 (m, 2 H), 7.15 (m, 2 H), and 6.98 ppm (m, 2 H). 13C NMR (100 MHz, CDCl3) δ: 134.8, 134.8, 134.5, 133.4, 133.3, 132.0, 130.4, 130.3, 129.2, 129.2, 128.8, 128.8, 128.8, 128.8, 128.3, 128.1, 128.1, 128.0, 127.79, 127.7, 127.5, 127.2, 127.1, 126.9, 126.5, 126.3, 126.3, 126.27, 125.3, 125.29, 124.6, 124.5, 124.49, 124.4, 124.0, 123.7, 123.3, 122.3, 79.5, 79.44, 64.4, and 54.7 ppm. Anal. Calcd for C140H10S3 (1787.8): C 94.06; H, 0.56; S, 5.38. Found: C, 94.15; H, 0,50; S, 5.35%.
5-(3-(2-Thienyl)benzo[c]-1-thienyl)thiophene-2-carbaldehyde (8).
Under argon atmosphere in a round bottom flask at 0 °C, POCl3 (625 µL, 1.03 g, 6.71 mmol) was slowly added to a mixture of dry DMF (519 µL, 490 mg, 6.71 mmol) and dry DCM (15 mL). After stirring for 1 h at 0 °C and 3 h at room temperature the reaction mixture became light yellow colored. Then the reaction mixture was added at 0 °C and under air exclusion to substrate 4 (1 g, 3.35 mmol) dissolved into dry DCM (30 mL) and stirred overnight at room temperature. In order to quench the reaction a saturated NaHCO3 solution was added and the mixture stirred for 1 h. After few extractions with DCM the organic phases were collected and by solvent evaporation the crude was obtained as a red thick oil. The subsequent purification by column chromatography using petroleum ether/EtOAc (9:1) as eluent mixture gave the desired product (8) as a red powder (787 mg; 72% yield). 1H NMR (400 MHz, CDCl3) δ: 9.91 (s, 1 H,), 8.03 (dd, J = 8.8 Hz, 2 H,), 7.77 (d, J = 4 Hz, 1 H,), 7.43 (m, 2 H), and 7.22 ppm (m, 4 H). 13C NMR (100 MHz, CDCl3) δ: 182.53, 147.90, 142.00, 137.27, 136.58, 135.85, 135.05, 130.09, 128.33, 126.82, 126.73, 126.66, 125.44, 125.40, 124.90, 122.22, and 121.51 ppm. GC-MS (EI) m/z: 326.0 (M+). Anal. Calcd for C17H10OS3 (326.5): C, 62.55; H, 3.09; S, 29.47. Found: C, 62.49; H, 3.12; S, 29.60%.
5-(3-(5-Bromo-2-thienyl)benzo[c]-1-thienyl)thiophene-2-carbaldehyde (9).
Under exclusion of the air, a solution of N-bromosuccinimide (NBS) (273 mg, 1.50 mmol) in 10 mL of dry DMF was added dropwise to a solution of compound 8 (500 mg, 1.50 mmol) in 50 mL of dry DMF at 0 °C. The resulting mixture was stirred for 3 h at 0 °C, afterwards the solution was wormed up to room temperature. The orange mixture obtained was poured into water and the red precipitate formed was filtered off and washed with water. The solid was purified further by column chromatography with the mixture hexanes/EtOAc (8:2) obtaining the desired product 9 as a deep red powder (568 mg, 93% yield). 1H NMR (400 MHz, CDCl3) δ: 9.81 (s, 1 H,), 7.93 (d, J = 8.0 Hz, 1 H), 7.79 (d, J = 8.0 Hz, 1 H,), 7.65 (m, 4 H), 7.45 (d, J = 4.0 Hz, 1 H), and 7.31 ppm (d, J = 4.0 Hz, 1 H,). 13C NMR (100 MHz, CDCl3) δ: 182.4, 137.1, 136.2, 135.4, 135.0, 134.1, 131.3, 131.2, 131.1, 131.0, 130.9, 129.4, 129.3, 126.5, 125.5, 125.4, and 121.3 ppm. GC-MS (EI) m/z: 405.9 (M+). Anal. Calcd for C17H9BrOS3 (405.4): C, 50.37; H, 2.24; S, 23.73. Found: C, 50.42; H, 2.30; S, 23.65%.
5-(3-(5-((Trimethylsilyl)ethynyl)-2-thienyl)benzo[c]-1-thienyl)thiophene-2-carbaldehyde (10).
A round bottom flask equipped with magnetic stir bar was charged with compound 9 (434 mg, 1.07 mmol), piperidine and dry toluene 1:4 (10 mL, 40 mL respectively) under argon atmosphere. Then the catalyst PdCl2(PPh3)2 4% mol (30 mg, 0.04 mmol), the co-catalyst CuI 6% mol (12 mg, 0.06 mmol) and TMSA 2.5 eq (468 µL, 323 mg, 3.29 mmol) were added. The reaction mixture was refluxed for 9 h. Then it was allowed to cool down to room temperature and filtered on florisil. The crude was purified by column chromatography on silica gel with the mixture hexanes/EtOAc (8:2) and the desired product 10 (144 mg, 32% yield) was collected as red greenish crystals. 1H NMR (400 MHz, CDCl3) δ: 9.84 (s, 1 H), 7.97 (d, J = 8.0 Hz, 1 H), 7.91 (d, J = 8.0 Hz, 1 H), 7.69 (d, J = 4.0 Hz, 1 H), 7.35 (d, J = 4.0 Hz, 1 H), 7.17 (m, 4 H), and 0.27 ppm (s, 9 H). 13C NMR (100 MHz, CDCl3) δ: 182.49, 145.65, 142.23, 137.19, 136.58, 136.52, 135.97, 133.60, 126.72, 125.94, 125.84, 125.83, 125.69, 125.53, 124.12, 122.08, 121.61, 101.69, 97.24, and 0.04 ppm. Anal. Calcd for C22H18OS3Si (422.7): C, 62.52; H, 4.29; S, 22.76. Found: C, 62.59; H, 4.21; S, 22.62%.
5-(3-(5-Ethynyl-2-thienyl)benzo[c]-1-thienyl)thiophene-2-carbaldehyde (11).
At room temperature the substrate 10 (316 mg, 0.75 mmol) in a mixture 1:1 of MeOH/THF (40 mL) was treated with an excess of K2CO3 (700 mg, 5.07 mmol) and was stirred overnight. The reaction mixture was filtered on celite and the solvent was removed by vacuum evaporation. The crude was purified by chromatography on silica gel with hexanes/EtOAc (8:2) to afford the desired compound 11 (103 mg, 39% yield) as a dark red amorphous powder. 1H NMR (400 MHz, CDCl3) δ: 9.79 (s, 1 H), 7.88 (d, J = 8.0 Hz, 1 H), 7.75 (d, J = 9.0 Hz, 1 H), 7.63 (m, 2 H), 7.27 (d, J = 4.0 Hz, 1 H), 7.10 (m, 2 H), 6.99 (s, 1 H), and 3.04 ppm (s, 1 H). 13C NMR (125 MHz, CDCl3) δ: 182.4, 145.4, 142.1, 137.1, 137.0, 136.4, 135.9, 134.1, 133.8, 128.9, 126.7, 126.4, 125.9, 125.6, 125.4, 121.8, 121.4, 83.5, and 83.3 ppm. Anal. Calcd for C19H10OS3 (350.5): C, 65.11; H, 2.88; S, 27.45. Found: C, 65.05; H, 2.95; S, 27.38%.
5,5'-(3,3'-(5,5'-(Buta-1,3-diyne-1,4-diyl)bis(thiophene-5,2-diyl))bis(benzo[c]thiophene-3,1-diyl))bis-(thiophene-2-carbaldehyde) (12).
A round bottom flask was charged with compound 11 (54 mg, 0.15 mmol) dissolved into dry toluene (12 mL). Then 2.1 equivalent of LiHMDS 1.0 M/THF (290 µL, 49 mg, 0.29 mmol) was added dropwise to the red solution. After the addition of LiHMDS, the reaction mixture was stirred at room temperature for 2 h. Successively, 2 more equivalents of LiHMDS (280 µL, 47 mg, 0.28 mmol) were added. After 1 h from the last addition this reaction mixture was refluxed for 1 h. After that the reaction mixture was turned from red to brown and it was cooled down to room temperature. The quenching was conducted adding slowly 1.5 mL of trifluoroacetic acid. At the end of the addition the mixture was red in color and was stirred at room temperature overnight. After removal of the solvent the crude mixture was diluted in DCM and then it was removed by filtration. The crude was purified by column chromatography on silica gel using toluene as eluent to afford the dimer 12 (25 mg, 55% yield) as a red orange solid. 1H NMR (400 MHz, CDCl3) δ: 9.90 (s, 2 H), 8.02 (d, J = 8.0 Hz, 2 H), 7.89 (d, J = 4.0 Hz, 2 H), 7.75 (d, J = 4.0 Hz, 2 H), 7.41 (d, J = 4.0 Hz, 2 H), 7.23 (m, 4 H), and 7.12 ppm (s, 4 H). 13C NMR (100 MHz, CDCl3) δ: 182.6, 149.9, 149.1, 146.1, 145.7, 142.2, 141.9, 137.3, 136.5, 136.5, 136.0, 131.1, 126.8, 126.7, 125.8, 125.7, 121.8, 121.6, and 113.5 ppm. Anal. Calcd for C38H18O2S6 (698.9): C, 65.30; H, 2.60; S, 27.53. Found: C, 65.38; H, 2.50; S, 27.48%.
1,2-Bis((5-(3-(2-thienyl)benzo[c]-1-thienyl)-2-thienyl)methylene)hydrazine (13).
The dimerization reaction was conducted by simply refluxing the monoaldehyde 8 (20 mg, 0.06 mmol) dissolved into 2 mL of EtOH and 2 mL of CHCl3 with an equimolar amount of hydrazine monohydrate (3 µL) in EtOH (500 µL). The reaction was carried out under argon atmosphere and at room temperature for 4 h and successively for 17 h at reflux temperature. The solvent was removed under low pressure evaporation and 2.5 mL of 1,1,2-trichloroethane were added and the mixture was allowed to reflux for 6 h, still under argon atmosphere. The mixture was cooled down and the solvent removed. The crude was purified by column chromatography in silica gel with the mixture hexanes/EtOAc (9:1) obtaining the desired dimer 13 (22 mg, 60% yield) as a deep violet solid. 1H NMR (400 MHz, CDCl3) δ: 8.79 (s, 2 H), 8.09 (d, J = 8.0 Hz, 1 H), 8.04 (d, J = 8.0 Hz, 1 H), 7.98 (m, 2 H), 7.75 (d, J = 4.0 Hz, 1 H), 7.39 (m, 6 H), and 7.18 ppm (m, 7 H). GC-HRMS (ESI) m/z: 649.00 (M+). C34H20N2S6 requires 648.93.
References
1. J. Roncali, Chem. Rev., 1997, 97, 173. CrossRef
2. M. D'Auria, Adv. Heterocycl. Chem., 2011, 104, 127. CrossRef
3. F. Wudl, M. Kobayashi, and A. J. Heeger, J. Org. Chem., 1984, 49, 3382. CrossRef
4. M. Kertesz, C. H. Choi, and S. Yang, Chem. Rev., 2005, 105, 3448. CrossRef
5. H. Neugebauer, C. Kvamstrom, C. Brabec, N. S. Sariciftci, R. Kiebooms, F. Wudl, and S. Luzzati, J. Chem. Phys., 1999, 110, 12108. CrossRef
6. B. L. Lee and T. Yamamoto, Macromolecules, 1999, 32, 1375. CrossRef
7. M. Kobayashi, J. Chen, T. C. Chung, F. Moraes, A. J. Heeger, and F. Wudl, Synth. Met., 1984, 9, 77. CrossRef
8. M. Kobayashi, N. Colaneri, M. Boysel, F. Wudl, and A. J. Heeger, J. Chem. Phys., 1985, 82, 5717. CrossRef
9. M. Pomerantz, B. Chaloner-Gill, L. O. Harding, J. J. Tseng, and W. J. Pomerantz, Synth. Met., 1993, 55, 960. CrossRef
10. D. L. Vangeneugden, R. H. L. Kiebooms, D. J. M. Vanderzande, and J. M. J. V. Gelan, Synth. Met., 1999, 101, 120. CrossRef
11. T.-T. Hung and S.-A. Chen, Polymer, 1999, 40, 3881. CrossRef
12. R. H. L. Kiebooms, P. J. A. Adriaensens, D. J. M. Vanderzande, and J. M. J. V. Gelan, J. Org. Chem., 1997, 62, 1473. CrossRef
13. P. Bäurie, G. Gotz, P. Emerle, and H. Port, Adv. Mater., 1992, 4, 564. CrossRef
14. D. Lorcy and M. P. Cava, Adv. Mater., 1992, 4, 562. CrossRef
15. A. K. Mohanakrishnan, M. V. Lakshmikantham, C. McDougal, M. P. Cava, J. W. Baldwin, and R. M. Metzger, J. Org. Chem., 1998, 63, 3105. CrossRef
16. U. Mitschke and P. Bäuerle, J. Chem. Soc., Perkin Trans. 1, 2001, 740. CrossRef
17. A. K. Mohanakrishnan, P. Amaladass, and J. Arul Clement, Tetrahedron Lett., 2007, 48, 779. CrossRef
18. D. L. Vangeneugden, D. J. M. Vanderzande, J. Salbeck, P. A. van Hal, R. A. J. Janssen, J. C. Hummelen, C. J. Brabec, S. E. Shaheen, and N. S. Sariciftci, J. Phys. Chem. B, 2001, 105, 11106. CrossRef
19. M. D'Auria, A. Guarnaccio, R. Racioppi, A. Santagata, and R. Teghil, Synlett, 2013, 24, 943. CrossRef
20. S. Musmanni and J. P. Ferraris, J. Chem. Soc., Chem. Commun., 1993, 172. CrossRef
21. G. Piancatelli, A. Scettri, and M. D'Auria, Synthesis, 1982, 245. CrossRef
22. R. Kisselev and M. Thelakkat, Chem. Commun., 2002, 1530. CrossRef
23. Y. K. Jung, H. Kim, J.-H. Park, J. Lee, S. K. Lee, Y. S. Lee, and H.-K. Shim, J. Polym. Sci., A: Polym. Chem., 2008, 46, 3573. CrossRef
24. F. Padinger, R. S. Rittberger, and N. S. Sariciftci, Adv. Funct. Mater., 2003, 13, 85. CrossRef
25. G. Li, V. Shrotriya, J. Huang, Y. Yao, T. Moriarty, K. Emery, and Y. Yang, Nature Mater., 2005, 4, 864. CrossRef
26. W. Ma, C. Yang, X. Gong, K. Lee, and A. J. Heeger, Adv. Funct. Mater., 2005, 15, 1617. CrossRef
27. J. Roncali, Chem. Soc. Rev., 2005, 34, 483. CrossRef
28. J. L. Segura, N. Martin, and D. M. Guldi, Chem. Soc. Rev., 2005, 34, 31. CrossRef
29. A. Cravino and N. S. Sariciftici, J. Mater. Chem., 2002, 12, 1931. CrossRef
30. T. Otsubo, Y. Aso, and K. Takimiya, Pure Appl. Chem., 2005, 77, 2003. CrossRef
31. T. Benincori, E. Brenna, F. Sannicolò, L. Trimarco, G. Zotti, and P. Sozzani, Angew. Chem., Int. Ed. Engl., 1996, 35, 648. CrossRef
32. A. Yassar, M. Hmyene, D. C. Loveday, and J. P. Ferraris, Synth. Met., 1997, 84, 231. CrossRef
33. A. Cravino, G. Zerza, M. Maggini, S. Bucella, M. Svensson, M. R. Andersson, H. Neugebauer, and N. S. Sariciftci, Chem. Commun., 2000, 2487. CrossRef
34. A. Cravino, G. Zerza, H. Neugebauer, M. Maggini, S. Bucella, E. Menna, M. Svensson, M. R. Andersson, C. J. Brabec, and N. S. Sariciftci, J. Phys. Chem. B, 2002, 106, 70. CrossRef
35. Y. Murata, M. Suzuki, and K. Komatsu, Org. Biomol. Chem., 2003, 1, 2624. CrossRef
36. T. Yamazaki, Y. Murata, K. Komatsu, K. Furukawa, M. Morita, N. Maruyama, T. Yamao, and S. Fujita, Org. Lett., 2004, 6, 4865. CrossRef
37. F. Effenberger and G. Grube, Synthesis, 1998, 1372. CrossRef
38. S.-G. Liu, L. Shu, J. Rivera, H. Liu, J.-M. Raimundo, J. Roncali, A. Gorgues, and L. Echegoyen, J. Org. Chem., 1999, 64, 4884. CrossRef
39. T. Yamashiro, Y. Aso, T. Otsubo, H. Tang, Y. Harima, and K. Yamashita, Chem. Lett., 1999, 28, 443. CrossRef
40. P. A. van Hal, J. Knol, B. M. W. Langeveld-Voss, S. C. J. Meskers, J. C. Hummelen, and R. A. J. Janssen, J. Phys. Chem. A, 2000, 104, 5974. CrossRef
41. N. Negishi, K. Takimiya, T. Otsubo, Y. Harima, and Y. Aso, Chem. Lett., 2004, 33, 654. CrossRef
42. Y. Obara, K. Takimiya, Y. Aso, and T. Otsubo, Tetrahedron Lett., 2001, 42, 6877. CrossRef
43. M. Narutaki, K. Takimiya, T. Otsubo, Y. Harima, H. Zhang, Y. Araki, and O. Ito, J. Org. Chem., 2006, 71, 1761. CrossRef
44. H. Kanato, M. Narutaki, K. Takimiya, T. Otsubo, and Y. Harima, Chem. Lett., 2006, 35, 668. CrossRef
45. T. L. Chen, Y. Zhang, P. Smith, A. Tamayo, Y. Liu, and B. Ma, Appl. Mater. Interfaces, 2011, 3, 2275. CrossRef
46. K. Komatsu, Y. Murata, N. Takimoto, S. Mori, N. Sugita, and T. S. M. Wan, J. Org. Chem., 1994, 59, 6101. CrossRef
47. A. Lafleur-Lambert, S. Rondeau-Gagné, A. Soldera, and J. F. Morin, Tetrahedron Lett., 2011, 52, 5008. CrossRef
48. W. Hong, B. Sun, H. Aziz, W.-T. Park, and Y.-Y. Noh, Chem. Commun., 2012, 8413. CrossRef
49. Gaussian 09, Revision A.1, M. J. Frisch, G. W. Trucks, H. B. Schlegel, G. E. Scuseria, M. A. Robb, J. R. Cheeseman, G. Scalmani, V. Barone, B. Mennucci, G. A. Petersson, H. Nakatsuji, M. Caricato, X. Li, H. P. Hratchian, A. F. Izmaylov, J. Bloino, G. Zheng, J. L. Sonnenberg, M. Hada, M. Ehara, K. Toyota, R. Fukuda, J. Hasegawa, M. Ishida, T. Nakajima, Y. Honda, O. Kitao, H. Nakai, T. Vreven, J. A. Montgomery, Jr., J. E. Peralta, F. Ogliaro, M. Bearpark, J. J. Heyd, E. Brothers, K. N. Kudin, V. N. Staroverov, R. Kobayashi, J. Normand, K. Raghavachari, A. Rendell, J. C. Burant, S. S. Iyengar, J. Tomasi, M. Cossi, N. Rega, J. M. Millam, M. Klene, J. E. Knox, J. B. Cross, V. Bakken, C. Adamo, J. Jaramillo, R. Gomperts, R. E. Stratmann, O. Yazyev, A. J. Austin, R. Cammi, C. Pomelli, J. W. Ochterski, R. L. Martin, K. Morokuma, V. G. Zakrzewski, G. A. Voth, P. Salvador, J. J. Dannenberg, S. Dapprich, A. D. Daniels, O. Farkas, J. B. Foresman, J. V. Ortiz, J. Cioslowski, and D. J. Fox, Gaussian, Inc., Wallingford CT, 2009.