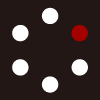
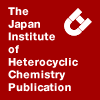
HETEROCYCLES
An International Journal for Reviews and Communications in Heterocyclic ChemistryWeb Edition ISSN: 1881-0942
Published online by The Japan Institute of Heterocyclic Chemistry
e-Journal
Full Text HTML
Received, 10th December, 2014, Accepted, 26th January, 2015, Published online, 5th February, 2015.
DOI: 10.3987/COM-14-13149
■ Structures and Bioactivities of Xenicanes from an Okinawan Soft Coral Xenia sp.
Wilmar Maarisit, Kentaro Yano, Tomofumi Miyazato, Prodip K. Roy, Junsei Taira, and Katsuhiro Ueda*
Department of Chemistry, Biology and Marine Sciences, Faculty of Science, University of Ryukyus, 1 Senbaru, Nishihara-cho, Okinawa 903-0213, Japan
Abstract
Three new xenicane diterpenoids 1–3 were isolated from a soft coral Xenia sp. together with the known xenicanes 4–7. Their structures were determined by spectroscopic methods and the structure of 1 was further confirmed by single X-ray diffraction analysis. Metabolites 1–7 showed weak cytotoxicity against HCT 116 cells and a dose dependent, anti-inflammatory effect in LPS/IFN-γ-stimulated inflammatory RAW 264.7 macrophage cells.INTRODUCTION
Soft corals are rich sources of novel secondary metabolites.1,2 The majority of compounds identified from soft corals fall into the category of diterpenoids, which are of considerable interest due to their cytotoxic and antibacterial activities.1,2 In the course of our study on the secondary metabolites from Okinawan marine organisms, we investigated a soft coral Xenia sp.3,4 This paper describes the isolation, structure elucidation of three new xenicanes 1–3 and bioactivities of isolates 1–7.
RESULTS AND DISCUSSION
Samples (9.5 kg, wet weight) of the Xenia sp. overgrown on a coral reef were collected by hand from the coast of Miyako Island, Okinawa, and extracted with acetone. The acetone extracts were partitioned between water and ethyl acetate. The EtOAc extract was concentrated to yield a heterogeneous brown mixture (60.0 g), which was separated by filtration. The filtrate and the red solids were fractionated by column chromatography on silica gel separately. Each fraction was checked by 1H NMR spectroscopy, and the fractions showing proton resonances for xenicane diterpenes were fractionated over a SiO2 column followed by HPLC purification to give three new compounds 1–3 and four known compounds 4–7. The structures of the new compounds were determined by extensive analysis of spectroscopic data. The known compounds were unambiguously identified by comparison of obtained spectral data with those described in the literature.5-7
Compound 1 was obtained as colorless crystals, [α]D22 −54 (c 0.42 CHCl3). The molecular formula of 1 was determined to be C20H26O5 by HRESIMS [m/z 369.1673 (M+Na)+, calcd for C20H26O5Na, 369.1677], which accounted for eight degrees of unsaturation. IR absorption bands at 3446 and 1702 cm−1 indicated the presence of hydroxy and carbonyl groups.
The 1H and 13C NMR data of 1 are summarized in Tables 1 and 2. These data indicated the presence of one ester (δC 168.7), one trisubstituted double bond (δC 141.1, δH 7.17; δC 129.4), one disubstituted double bond (δC 151.1, δH 6.23; δC 121.7, δH 6.85), one exocyclic double bond (δC 142.1; δC 117.5, δH 5.19, 5.24), two quarternary carbons (δC 71.1; δC 54.8), four methines (δC 58.2, δH 3.07; δC 55.7, δH 3.24; δC 43.6, δH 2.75; δC 33.7, δH 3.64), five methylenes (δC 69.3, δH 4.12, 4.28; δC 49.1, δH 2.88, 2.71; δC 32.7, δH 2.83, 2.73; δC 32.7, δH 2.21, 1.80; δC 28.2, δH 1.81), and two methyls (δC 29.7, δH 1.36; δC 29.3, δH 1.34). The NMR data of 1 showed similarities to those of xeniatine A epoxide (4)5 and xeniolide K (xenicane possessing the 7(18), 8(9) diepoxy moieties).8 Compared to 4, the 13C NMR data showed the absence of a methyl group and as well as the presence of an additional methylene. The methylene at C-18 (δC 49.1, δH 2.88, 2.71) and the quaternary carbon at C-7 (δC 54.8) were assigned as part of an additional
epoxide based on an HMBC correlation (H-18/C-7) and the NMR chemical shifts. 1H-1H COSY correlations revealed three spin systems [a (H-1/H-11a/H-4a/H-5/H-6), b (H-8/H-9/H-10) and c (H-12/H-13/H-14)] (Figure 1). HMBC correlations (H-1/C-3; H-4a/C-3, -4; H2-6/C-7; H-8/C-7; H-10/C-11a; H-12/C-3, -4; H-14/C-15; H3-16/C-14, -15; H3-17/C-14, -15; H2-19/C-10, -11, -11a) finally connected the partial structures (a, b, c) and other fragments (O–C-3–C-4, C-7–C-18, C-11–C-19, C-16–C-15–C-17) to give the planar structure of 1 (Figure 1).
The large coupling constant of J13,14 = 15.2 Hz along with no NOE effect on H-13 and H-14 established E geometry at C-13/C-14. The NOESY correlation of H-13/H-5 showed E geometry at C-4/C-12. The NOESY correlations of H-9/H-8, -10β, -19b, H-11a/H-1β, -19a suggested that H-8, H-9 and H-11a were on the same β face on the ring system, while NOESY cross-peaks between H-4a/H-1α, -10α showed that these protons were on the α face on the ring system. The epoxide stereochemistry at C-7/C-18 was inferred as depicted in Figure 1 by considering the NOESY correlations of H-8/H-6, -18a and by assuming common biogenesis of 1 and isoxeniatine A epoxide (4). The full relative stereochemistry of 1 was secured by X-ray diffraction analysis (Figure 4).
Compound 2 obtained as a colorless oil, [α]D24 −24 (c 0.49 CHCl3), had the same molecular formula as that of 1, as deduced from HRESIMS [m/z 369.1647 (M+Na)+, calcd for C20H26O5Na, 369.1677]. IR absorption bands at 3446 and 1705 cm−1 indicated the presence of hydroxy and carbonyl groups.
The 1H and 13C NMR data of 2 (Tables 1 and 2) are very similar to those of 1, except for 1H NMR resonances of H-12 and H-13, suggesting that 1 and 2 could be geometric isomers. The large coupling constant of J13,14 = 15.5 Hz along with no NOE effect on H-13 and H-14 established E geometry at C-13/C-14. The NOEs between H-12/H-4a, -5 showed Z geometry at C-4/C-12. Extensive analysis of the 1D and 2D NMR data led to the planar structure of 2. Further NOEs between H-1α/H-4a, -10α, H-4a/H-10α, H-6/H-8, H-8/H-9, H-9/H-10β, -19b, H-10β/H-19b, H-11a/H-19a allowed us to assign the orientation of H-11a, H-8 and H-9 as β and H-4a as α. The C-7 oxygen atom was tentatively deduced to be in an α orientation by comparing the NOE data of 2 with those of 1 and by assuming a common biosynthetic route for 2 and 1.
Compound 3 was obtained as a colorless oil, [α]D24 −81 (c 0.46 CHCl3). The 13C NMR (Table 2) and HRESIMS [m/z 355.1863 (M+Na)+, calcd for C20H28O4Na, 355.1885] data revealed that 3 had a formula of C20H28O4. 1H and 13C NMR data indicated the presence of two trisubstituted double bond (δC 142.8; δC 120.5, δH 5.40 and δC 133.8; δC 125.2, δH 5.12), one exocyclic double bond (δC 141.9; δC 119.7, δH 5.18, 5.14), one quaternary carbon (δC 72.2), four methines (δC 107.4, δH 5.37; δC 83.7, δH 5.53; δC 61.7, δH 2.77; δC 61.0, δH 3.06; δC 53.5, δH 2.36; δC 35.1, δH 3.35), four methylenes (δC 69.3, δH 3.70, 3.43; δC 34.1, δH 1.90, 1.62; δC 29.1, δH 2.72, 2.64; δC 25.5, δH 1.77, 1.65), and three methyls (δC 32.2, δH 1.36; δC 25.6, δH 1.71; δC 18.1, δH 1.72). The NMR data of 3 showed similarities to those of xeniatine A epoxide (4)5 and novaxenicin A (containing a dihydrofuran-tetrahydropyran-cyclononane skeleton).9 Compared to xeniatine A epoxide, the 13C NMR data indicated the presence of an acetal instead of an ester. The major spin systems [d (H-1/H-11a/H-4a/H-5/H-6), e (H-8/H-9/H-10) and f (H-12/H-13/H-14)] were revealed based on 1H-1H COSY data (Figure 5). The partial structures (d and f) and the acetal moiety (C-3–C-4) were connected using HMBC correlations of H-1/C-3 and H-3/C-4, -12, -13, to give the planar structure of the A- and C-ring moieties. The correlation peaks of H-6/C-7, H-8/C-7, H2-10/C-11, H-12/C-4, H3-18/C-6, -7 and H2-19/C-10, -11a allowed us to assemble the uncoupled moieties (C-7, C-11–C-19, C-18) and the partial structures d and e, to draw the planar structure of the cyclononane moiety. Finally, attachment of the partial structure f to the dimethyl-bearing C-15 was deduced by the cross-peaks exhibited by the methyl protons H3-16 and H3-17 (Figure 5), thus establishing the planar structure of 3. The relative stereochemistry of 3 was established based on the NOE data and by the analysis of coupling constants. Comparable coupling constants between H-4a/H-11a in 3 (J = 11.7 Hz) and 4 (J = 11.0 Hz)5 along with no NOE on H-4a and H-11 suggested that the protons at C-4a and C-11a were trans-oriented. A cis-configuration for the 8(9) epoxy moiety was determined from the measured 4.4 Hz coupling constant. 9 This was further supported by an NOE between H-8 and H-9. Further NOEs (H-3/H-1α, -4a, -13; H-4a/H-1α, -10α; H-11a/H-1β, -19b; H-19a/H-9, -10β) allowed us to assign the orientation of H-3, H-4a and H-13 as α and H-8, H-9 and H-11a as β. The hydroxy group at C-7 was in an α orientation from the presence of the NOESY correlations between H-8/H-6β, -18 and H-18/H2-6. The suggested stereochemistry of the A-, B- and C-ring moieties is the same as in a known Xenia diterpene, novaxenicin A.9
Assuming a common biosynthetic route for all isolates, the absolute stereochemistry of 1, 2 and 3 could be depicted on the basis of the stereostructures of the known isolates, as shown in Figure 1.
Three new xenicane diterpenoids 1–3 were isolated from the Okinawan soft coral Xenia sp. together with the known xeniatine A epoxide (4), xeniaether C (5), isoxeniatine C (6) and isoxeniatine A (7).5-7 Metabolites 1, 2, 4, 6 and 7 belong to the class of xeniolide A (possessing a δ-lactone-cyclononane skeleton)8,9 and metabolite 3 belongs to the class of novaxenicin A (containing a dihydrofuran-tetrahydropyran-cyclononane skeleton).9 Metabolite 5 was a xeniaether A congener (containing a tetrahydrofuran-cyclononane skeleton).5
Compounds 1–7 were evaluated for anti-tumor activity in HCT116 colon cancer cells (human colorectal cancer cells) (Figure 7). All isolates showed weak cytotoxicity (IC50s, >83.6 μM). These compounds were also assessed for anti-inflammatory activity in LPS/IFN-γ-stimulated inflammatory RAW 264.7 macrophage cells and showed a dose dependent, anti-inflammatory effect (Figure 8). The lack of cytotoxicity against RAW 264.7 macrophage cells in the test concentration range indicated that inhibition of nitric oxide production was due to the effects of 1–7 (Figure 9). Further chemical and biological studies on the extract of the soft coral Xenia sp. are in progress in our laboratory.
EXPERIMENTAL
General Aspects
Optical rotations were measured on a JASCO P-1010 polarimeter. UV spectra of the methanol solutions were measured on a JASCO V-660 spectrophotometer. IR spectra were recorded on a JASCO FT/IR-600 spectrometer. The 1H, 13C, and 2D NMR spectra were recorded on a Bruker Avance III 500 spectrometer, and 1H and 13C chemical shifts were referenced to the solvent peaks (δH 7.26 and δC 77.24 in CDCl3). Mass spectra were measured on a JEOL JMS-T100LP mass spectrometer. X-Ray crystallographic data were collected on a RIGAKU Saturn724+instrument. Open column chromatography was performed on Kieselgel 60 (70-230 mesh, Merck). HPLC was performed using a COSMOSIL-packed ODS HPLC column (C18, 10 x 250 mm) or COSMOSIL Si60 HPLC column (5SL, 10 x 250 mm). Analytical and preparative TLC were performed using Kieselgel 60 F254 DC-fertigplatten (Merck). All solvents used were reagent grade.
Animal Material. The soft coral was collected at low tide from the coast of Miyako Island, Okinawa, Japan in April, 2013, and identified as Xenia sp. A voucher specimen was deposited at the University of the Ryukyus (Specimen no. 1304011).
Isolation.
The soft coral Xenia sp. (wet weight 9.5 kg) was soaked in acetone (6.5 L) two times at room temperature and filtered. After filtration, the extract was concentrated under reduced pressure and partitioned between EtOAc (400 mL x 2) and distilled water (400 mL). The EtOAc extract was concentrated to yield a heterogeneous brown mixture (60.0 g). The mixture was separated by filtration and the red solids were chromatographed on a silica gel column using a linear gradient system from n-hexane–EtOAc (1:1) to EtOAc to give twelve fractions. Fraction 10 (94.5 mg) was purified by PTLC (silica gel) using CHCl3–MeOH (20:1, twice developments) to afford compound 1 (7.3 mg, 7.7×10-6 %, wet weight). The filtrate was concentrated and the resulting brown gum (57.4 g) was fractionated by column chromatography (CC) on silica gel using hexanes with increasing proportions of EtOAc to yield twelve fractions. The sixth fraction was determined to be the known xeniaether C (423.0 mg, 4.5×10-4 %). The tenth fraction (1.432 g) was subjected to further separation by CC on silica gel using the gradient solvent mixture of n-hexane–EtOAc–MeOH to afford ten fractions. The fifth fraction (818.8 mg) was separated by CC on silica gel using CH2Cl2–2-propanol (25:1) to give ten fractions. Fractions 5 and 7 afforded xeniatin A epoxide (106.5 mg, 1.1×10-4%) and isoxeniatin C (235.6 mg, 2.5×10-4%), respectively. Fraction 3 (35.3 mg) was purified by PTLC (silica gel) using n-hexane–EtOAc–2-propanol (20:30:1) to afford compound 2 (13.9 mg, 1.5×10-5 %). Fraction 10 was further chromatographed on a silica gel column using a linear gradient system from n-hexane–EtOAc (1:1) to EtOAc. The first fraction (67.1 mg) was purified by HPLC (silica gel) using n-hexane-EtOAc (1:2) to yield isoxeniatin A (21.6 mg, 2.5×10-5 %).
Compound 1: Colorless crystals (crystallized from n-hexane/CH2Cl2); mp 153–154 ˚C; [α]D22 −54 (c 0.42 CHCl3); FT IR νmax (film) 3446, 2974, 1702, 1635, 1457 and 1119 cm-1; UV λmax (MeOH) 274 nm (log ε 4.25); 1H and 13C NMR (CDCl3) data are listed in Tables 1 and 2; HRESIMS m/z 369.1673 (M+Na)+ (calcd for C20H26O5Na, 369.1677).
Compound 2: Colorless oil; [α]D24 −24 (c 0.49 CHCl3); FT IR νmax (film) 3446, 2974, 1705, 1685, 1507 and 1191 cm-1; UV λmax (MeOH) 272 nm (log ε 3.83); 1H and 13C NMR (CDCl3) data are listed in Tables 1 and 2; HRESIMS m/z 355.1863 (M+Na)+ (calcd for C20H28O4Na, 355.1885).
Compound 3: Colorless oil; [α]D24 −81 (c 0.46 CHCl3); FT IR νmax (film) 3464, 2971, 1718, 1634, 1458 and 1126 cm-1; 1H and 13C NMR (CDCl3) data are listed in Tables 1 and 2; HRESIMS m/z 355.1863 (M+Na)+ (calcd for C20H28O4Na, 355.1885).
X-Ray Structure Determination of 1. A colorless prism crystal of C20H26O5 having approximate dimensions of 0.682 x 0.047 x 0.040 mm was mounted on a glass fiber. All measurements were made on a Rigaku Saturn724 diffractometer using graphite monochromated Mo-Kα radiation. Cell constants and an orientation matrix for data collection corresponded to a primitive orthorhombic cell with dimensions: a = 5.893(2) Å, b = 13.166(5) Å, c = 23.616(9) Å, V = 1832.4(12) Å3. The crystal belongs to the space group P212121. Of the 15073 reflections that were collected, 4139 were unique (Rint = 0.0810); equivalent reflections were merged. Data were collected and processed using CrystalClear (Rigaku). The structure was solved by direct methods and expanded using Fourier techniques. Additional details can be found in the supporting information.
ACKNOWLEDGEMENTS
The authors thank Prof. Yehuda Benayahu (Tel-Aviv University, Israel) for identifying the soft coral.
References
1. D. J. Faulkner, Nat. Prod. Rep., 2002, 19, 1.
2. J. W. Blunt, B. R. Copp, R. A. Keyzers, M. H. G. Munro, P. T. Northcote, and M. R. Prinsep, Nat.
Prod. Rep., 2013, 30, 237
. CrossRef
3. D. J. Vanderah, P. A. Steudler, L. S. Ciereszko, F. J. Schmitz, J. D. Ekstrand, and D. Van der Helm, J. Am. Chem. Soc., 1977, 99, 5780. CrossRef
4. E. Fattorusso, A. Romano, O. Taglialatela-Scafati, M. J. Achmad, G. Bavestrello, and C. Cerrano,
Tetrahedron, 2008, 64, 3141
. CrossRef
5. T. Iwagawa, Y. Amano, T. Hase, and M. Shiro, Tetrahedron, 1995, 51, 11111. CrossRef
6. T. Iwagawa, Y. Amano, Y. H. Okamura, M. Nakatani, and T. Hase, Heterocycles, 1996, 43, 1271. CrossRef
7. T. Iwagawa, T. Matsuda, H. Okumura, and M. Nakatani, Tetrahedron, 1996, 43,13121.
8. Y. Kashman and A. Groweiss, Tetrahedron Lett., 1978, 4833. CrossRef
9. A. Bishara, A. Rudi, I. Goldberg, Y. Benayahu, and Y. Kashman, Tetrahedron, 2006, 62, 12092. CrossRef
10. J. Taira, H. Nanbu, and K. Ueda, Food Chemistry, 2009, 115, 1221 CrossRef