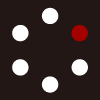
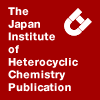
HETEROCYCLES
An International Journal for Reviews and Communications in Heterocyclic ChemistryWeb Edition ISSN: 1881-0942
Published online by The Japan Institute of Heterocyclic Chemistry
e-Journal
Full Text HTML
Received, 12th December, 2014, Accepted, 26th January, 2015, Published online, 30th January, 2015.
DOI: 10.3987/COM-14-13152
■ Development of a Probe Containing Novel Fluorescent Tricyclic-Nucleoside Analogs for Detecting Single Nucleotide Polymorphisms
Ayako Moriya, Tokimitsu Ohki, Aya Hayai, and Yoshihito Ueno*
Faculty of Applied Biological Sciences, Gifu University , 1-1 Yanagido, Gifu, Gifu 501-1193, Japan
Abstract
Here we report the synthesis of nucleoside analogs containing several aryl groups at the 6-position of the fluorescent tricyclic base, 8-amino-3-(2,3-dihydroxypropyl)imidazo[4′,5′:5,6]pyrido[2,3-d]pyrimidine (1), to expand the fluorescence color range of single nucleotide polymorphism (SNP)-detecting probes. The identity of the RNA target bases could be determined using probes containing an analog with a phenyl group at the 6-position of 1.INTRODUCTION
Single nucleotide polymorphisms (SNPs) serve as markers for the prediction of an individual’s response to certain drugs, and his or her risk of developing particular diseases. Therefore, the development of accurate, rapid, and cost-effective methods for SNP genotyping has become one of the most important subjects in pharmacogenomics, and for the advancement of personalized medicine.1,2
Recently, we reported the synthesis of novel probes for SNP detection using the fluorescent nucleoside analogs 1 and 2 (Figure 1).3,4 The fluorescence intensity of 1 and 2 are dependent on solvent polarity; the nucleoside analogs 1 and 2 exhibit higher fluorescence in more polar solvents, such as methanol and water, than in less polar solvents, such as chloroform. The probes for detection of SNPs are comprised of 1 or 2 and a discriminating D nucleoside (dA, dG, dC, or dT) (Figure 2). When the Ds are complementary to the target sequence, they can form base pairs with the target nucleosides, causing 1 or 2 to flip outside of the DNA helix, detectable by an increase in fluorescence intensity. However, when the target nucleoside is mismatched with the D, 1 or 2 intercalates into the DNA helix, since the tricyclic base-linked nucleoside analogs 1 and 2 are more intercalative than natural nucleosides. This weakens their fluorescence intensity. Thus, the identity of the target nucleoside can be determined by comparing the fluorescence intensity of each duplex.
In this paper, we report on the synthesis of nucleoside analogs 3−6, containing several aryl groups at the 6-position of the tricyclic base of 1, to expand the fluorescence color range of the probes. In addition, we characterized the properties of DNA sequences containing analog 3 as SNP-detecting probes.
RESULTS AND DISCUSSION
The method for synthesis of the tricyclic base-linked nucleoside surrogates 3−6 is shown in Scheme 1. Honjo et al. reported that treatment of 5-amino-4-cyano-1-(β-D-ribofuranosyl)imidazole with alkyl- and aryl-nitriles produced moderate yields of the corresponding 2-substituted adenine-arabinosides.5 Thus, we applied this method for synthesis of our compounds. Compound 7, which was synthesized using a previously reported method,3 was treated with benzonitrile in a methanolic ammonia solution in a sealed steel tube at 110 °C, to give the corresponding 6-phenyl tricyclic derivative 8 in 80% yield. Deprotection of the isopropylidene group with 80% AcOH afforded 3 in 67% yield. Similarly, compounds 4−6 were obtained by treating 7 with 4-dimethylaminobenzonitrile, anisonitrile, or 2-furonitrile, followed with 80% AcOH in 64, 64, and 70% yields, respectively.
The photophysical properties of compounds 3−6 were examined. Typical emission spectra for compounds 4−6 are shown in Figure 3. The fluorescence intensities of all compounds were dependent on solvent polarity. The fluorescence intensities of 3 and 6 were greater in more polar solvents, such as methanol and water, than in less polar solvents, such as chloroform. In contrast, the fluorescence intensity of the dimethylaminophenyl derivative 4 was greater in less polar solvents than in more polar solvents. The fluorescence intensity of 5 was lower in water than in other solvents. The fluorescence quantum yield (Φ) of 4 in chloroform was 0.51, whereas those of 3, 5, and 6 in methanol were 0.58, 0.61, and 0.31, respectively. Compound 4 showed an emission maximum at ~515 nm under 404-nm excitation in chloroform, whereas 3, 5, and 6 exhibited emission maxima at ~410, ~417, and ~411 nm under 270-, 355-, and 282-nm excitation in methanol, respectively. Thus, the fluorescence emission maxima of 3, 5, and 6 were similar to those of 1 and 2. Although the emission wavelength of 4 was longer than those of 1 and 2, 4 was shown to be unsuitable as a nucleoside surrogate for use in this method because its fluorescence intensity was lower in more polar solvents than in less polar solvents.
Next, to investigate the effect of an aryl group at the 6-position of the tricyclic base on SNP detection, we examined the properties of DNA probes containing 3 (B). The synthetic route for the phosphoramidite unit 14 is shown in Scheme 2. The amino group of 3 was protected with a (di-n-butylamino)methylene group in 82% yield. Then, the primary hydroxy group of 12 was protected with a 4,4′-dimethoxytrityl (DMTr) group in 71% yield. Phosphitylation of the hydroxy group of 13 gave the corresponding phosphoramidite unit 14 in 91% yield. In the present study, we chose a CYP2C9 sequence containing an A1075(C) mutation as a model sequence.6 The sequences of the DNAs containing 3 and the target RNAs are given in Table 1. The 5′-P-probes, termed BA, BG, BC, and BT, contained B at the 5′ end of the Ds, whereas the 3′-P-probes, termed AB, GB, CB, and TB, contained B at the 3′ end of the Ds. All ODNs containing 3 (B) were synthesized using a DNA/RNA synthesizer.
The fluorescence emission spectra of the duplexes containing the 3′-B-probes, AB, GB, CB, and TB, are shown in Figure 4 (left column). In all sequences, the fluorescence intensities were greatest when the discriminating bases were complementary to the target bases: the AB probe for the SrU target, the CB probe for the SrG target, the TB probe for the SrA target, and the GB probe for the SrC target. Thus, the identity of the target base can be determined using the 3′-B-probes. The results for probes containing the surrogate B at the 5′ side of the discriminating bases (5′-B-probes) are also shown in Figure 4 (right column). The BC and BG probes were effective, but the BT and BA probes were ineffective as SNP-detecting probes. Using our method, when mismatched bases are present opposite the discriminating bases, the nucleoside surrogate must be intercalated into the DNA-RNA duplex to quench the fluorescence of the surrogate. However, in the case of the BT and BA probes, the bulky phenyl group attached at the 6-position of the base moiety might prevent intercalation of the nucleoside surrogate B into the DNA-RNA duplex.
In conclusion, we demonstrated the synthesis of nucleoside surrogates 3 (B)−6 with aryl groups at the 6-position of the tricyclic base of 1 and DNA probes containing 3 (B). The fluorescence emission maxima of 3 (B), 5, and 6 were similar to those of 1 and 2, whereas the emission wavelength of 4 was longer than those of 1 and 2. The 3′-B-probes were effective as SNP-detecting probes, but some of the 5′-B-probes were ineffective as SNP-detecting probes. The bulky phenyl group attached at the 6-position of some of the 5′-B-probes might prevent the nucleoside surrogate B from intercalating into the DNA-RNA duplex.
EXPERIMENTAL
General remarks. Thin-layer chromatography was carried out on Merck coated plates 60F254. Silica gel column chromatography was carried out on Silica Gel 60N (spherical, neutral). 1H-, 13C-, and 31P-NMR spectra were obtained with a JEOL ECX-400P or JEOL ECA-600 spectrometer. CDCl3 (CIL) or DMSO-d6 (CIL) was used as a solvent for obtaining NMR spectra. Chemical shifts (δ) are given in parts per million (ppm) downfield from (Me)4Si (δ 0.00 for 1H-NMR in CDCl3), 80% H3PO4 (δ 0.00 for 31P-NMR), or a solvent (for 13C-NMR and 1H-NMR in DMSO-d6) as an internal reference with coupling constants (J) in Hz. The abbreviations s, d, and q signify singlet, doublet, and quartet, respectively.
(S)-8-Amino-3-[(2,2-dimethyl-1,3-dioxolan-4-yl)methyl]-6-phenylimidazo[4′,5′:5,6]pyrido[2,3-d]py-rimidine (8). Compound 7 (0.50 g, 1.83 mmol) and benzonitrile (3.8 g, 36.6 mmol) were dissolved in 7 M methanolic NH3 (20 mL) in a stainless steel portable reactor at 0 ºC. The portable reactor was sealed and the resulting solution was stirred at 140 ºC for 2 days. After cooling the mixture, the tube was opened and the excess NH3 was allowed to escape slowly. The mixture was concentrated. The residue was purified by column chromatography (SiO2, 3% MeOH in CHCl3) to give 8 (0.55 g, 1.47 mmol) in 80% yield: 1H NMR (400 MHz, DMSO-d6) δ 1.23 (s, 3H), 1.30 (s, 3H), 3.84 (dd, J = 8.7 and 5.5, 1H), 4.09 (dd, J = 8.3 and 6.8, 1H), 4.39-4.44 (dd, J = 14.2 and 6.4, 1H), 4.50-4.55 (dd, J = 14.2 and 4.1, 1H), 4.57-4.61 (m, 1H), 7.46-7.53 (m, 3H), 8.06 (s, 2H), 8.50 (dd, J = 6.7 and 4.6, 2H), 8.62 (s, 1H), 9.05 (s, 1H); 13C NMR (100 MHz, DMSO-d6) δ 25.6, 27.1, 46.0, 66.7, 73.9, 105.4, 109.5, 123.8, 128.6, 128.8, 130.8, 134.2, 139.0, 150.7, 152.4, 156.7, 162.3, 164.6; HRMS (DART) calcd for C20H20N6O2 (M+H+): 377.1726, found; 377.1701. Anal. Calcd for C20H20N6O2·1/5H2O: C, 63.21; H, 5.41; N, 22.11. Found: C, 63.26; H, 5.23; N, 21.90.
(S)-8-Amino-3-[2,3-dihydroxypropyl]-6-phenylimidazo[4′,5′:5,6]pyrido[2,3-d]pyrimidine (3). A solution of 8 (0.55 g, 1.5 mmol) in 80% AcOH (15 mL) was stirred at 60 °C for 22 h. The mixture was concentrated. The residue was purified by column chromatography (SiO2, 1% MeOH in CHCl3) to give 3 (0.31 g, 0.91 mmol) in 67% yield: 1H NMR (400 MHz, DMSO-d6) δ 3.34-3.47 (m, 2H), 3.95-3.98 (m, 1H), 4.16-4.18 (dd, J = 13.7 and 8.2, 1H), 4.51-4.54 (dd, J = 10.3 and 3.4, 1H), 4.94-4.96 (t, J = 5.9, 1H), 5.18 (d, J = 5.5, 1H), 7.49-7.51 (m, 3H), 8.04 (s, 2H), 8.50-8.52 (dd, J = 7.6 and 2.1, 2H), 8.58 (s, 1H) , 9.04 (s, 1H); 13C NMR (151 MHz, DMSO-d6) δ 47.9, 64.3, 69.9, 105.3, 123.5, 128.6, 128.7, 130.8, 134.4, 139.0, 151.0, 152.5, 156.5, 162.2, 164.6. Anal. Calcd for C17H16N6O2·1/5H2O: C, 59.99; H, 4.86; N, 24.12. Found: C, 60.25; H, 4.97; N, 24.21.
(S)-8-Amino-3-(2,3-dihydroxypropyl)-6-(4-dimethylaminophenyl)imidazo[4′,5′:5,6]pyrido[2,3-d]py-rimidine (4). Compound 7 (0.25 g, 0.92 mmol) and 4-dimethylaminobenzonitrile (2.68 g, 18.3 mmol) were dissolved in 7 M methanolic NH3 (10 mL) in a stainless steel portable reactor at 0 ºC. The portable reactor was sealed and the resulting solution was stirred at 140 ºC for 2 days. After cooling the mixture, the tube was opened and the excess NH3 was allowed to escape slowly. The mixture was concentrated. The residue was purified by column chromatography (SiO2, 5% MeOH in CHCl3) to give 9 with a small amount of impurity. Subsequently, compound 9 (0.20 g, 0.48 mmol) was dissolved in 80% AcOH (10 mL) and the resulting mixture was stirred at 60 °C for 22 h. The mixture was concentrated. The residue was purified by column chromatography (SiO2, 17% MeOH in CHCl3) to give 4 (0.11 g, 0.29 mmol) in 64% yield: 1H NMR (400 MHz, DMSO-d6) δ 3.00 (s, 6H), 3.37-3.49 (m, 2H), 3.91-3.98 (m, 1H), 4.13 (dd, J = 13.8 and 8.7, 1H), 4.49 (dd, J = 13.7 and 3.2, 1H), 4.92 (t, J = 5.7, 1H), 5.15 (d, J = 5.5, 1H), 6.78 (d, J = 9.2, 2H), 7.83 (s, 2H), 8.34 (d, J = 9.2, 2H), 8.51 (s, 1H), 8.95 (s, 1H); 13C NMR (151 MHz, DMSO-d6) δ 46.3, 63.6, 69.3, 104.2, 111.1, 122.8, 125.6, 129.3, 133.1, 149.6, 151.7, 156.1, 162.0, 163.5. Anal. Calcd for C19H21N7O2·2/5H2O·1/2CH3CO2H: C, 57.65; H, 5.76; N, 23.53. Found: C, 57.32; H, 5.67; N, 23.85.
(S)-8-Amino-3-(2,3-dihydroxypropyl)-6-(4-methoxyphenyl)imidazo[4′,5′:5,6]pyrido[2,3-d]pyrimidi-ne (5). Compound 7 (1.00 g, 3.66 mmol) and anisonitrile (9.74 g, 73.2 mmol) were dissolved in 7 M methanolic NH3 (40 mL) in a stainless steel portable reactor at 0 ºC. The portable reactor was sealed and the resulting solution was stirred at 140 ºC for 2 days. After cooling the mixture, the tube was opened and the excess NH3 was allowed to escape slowly. The mixture was concentrated. The residue was purified by column chromatography (SiO2, 5% MeOH in CHCl3) to give 10 with a small amount of impurity. Subsequently, compound 10 was dissolved in 80% AcOH (40 mL) and the resulting mixture was stirred at 60 °C for 22 h. The mixture was concentrated. The residue was purified by column chromatography (SiO2, 10% MeOH in CHCl3) to give 5 (0.86 g, 2.35 mmol) in 64% yield: 1H NMR (400 MHz, DMSO-d6) δ 3.38-3.52 (m, 2H), 3.83 (s, 3H), 3.92-4.01 (m, 1H), 4.12-4.18 (dd, J = 13.7 and 8.7, 1H), 4.49-4.56 (dd, J = 14.2 and 3.6, 1H), 4.90-4.92 (t, J = 5.5, 1H), 5.14-5.19 (d, J = 5.5, 1H), 7.03-7.06 (d, J = 8.7, 2H), 7.95 (s, 2H), 8.44-8.46 (dd, J = 4.0 and 3.3, 2H), 8.55 (s, 1H), 9.00 (s, 1H); 13C-NMR (100 MHz, DMSO-d6) δ 47.1, 55.8, 64.3, 69.9, 105.0, 114.1, 123.5, 130.3, 131.5, 134.1, 150.8, 152.4, 156.6, 161.7, 162.0, 164.5; HRMS (DART) calcd for C18H18N6O3 (M+H+): 367.1517, found; 367.1485. Anal. Calcd for C18H18N6O3·1/3H2O: C, 58.04; H, 5.05; N, 22.56. Found: C, 58.01; H, 5.08; N, 22.26.
(S)-8-Amino-3-(2,3-dihydroxypropyl)-6-furanylimidazo[4′,5′:5,6]pyrido[2,3-d]pyrimidine (6). Compound 7 (0.25 g, 0.92 mmol) and 2-furonitrile (1.70 g, 18.3 mmol) were dissolved in 7 M methanolic NH3 (40 mL) in a stainless steel portable reactor at 0 ºC. The portable reactor was sealed and the resulting solution was stirred at 140 ºC for 24 h. After cooling the mixture, the tube was opened and the excess NH3 was allowed to escape slowly. The mixture was concentrated. The residue was purified by column chromatography (SiO2, 5% MeOH in CHCl3) to give 11 with a small amount of impurity. Subsequently, compound 11 was dissolved in 80% AcOH (40 mL) and the resulting mixture was stirred at 60 °C for 21 h. The mixture was concentrated. The residue was purified by column chromatography (SiO2, 10% MeOH in CHCl3) to give 6 (0.20 g, 0.63 mmol) in 70% yield: 1H NMR (600 MHz, DMSO-d6) δ 3.31-3.49 (m, 2H), 3.91-3.95 (m, 1H), 4.14 (dd, J = 14.4 and 8.2, 1H), 4.49 (dd, J = 11.0 and 3.4, 1H), 4.94 (t, J = 5.0, 1H), 5.18 (d, J = 4.8, 1H), 6.67 (dd, J = 3.5 and 1.4, 1H), 7.26 (d, J = 2.3), 7.87 (s, 1H), 8.08 (s, 2H), 8.56 (s, 1H), 9.00 (s, 1H); 13C NMR (151 MHz, DMSO-d6) δ 47.0, 64.2, 69.8, 105.2, 112.7, 113.2, 123.4, 134.2, 145.4, 150.8, 152.3, 153.4, 155.8, 156.1, 164.5. Anal. Calcd for C15H14N6O3·1/3 H2O: C, 54.22; H, 4.45; N, 25.29. Found: C, 54.44; H, 4.53; N, 25.00.
(S)-8-[(Di-n-butylamino)methyleneamino]-3-(2,3-dihydroxypropyl)-6-phenylimidazo[4′,5′:5,6]pyri-do[2,3-d]pyrimidine (12). A solution of 3 (0.10 g, 0.30 mmol) and N,N-di-n-butylformamide dimethyl acetal (0.30 g, 1.50 mmol) in DMF (2 mL) was stirred at 60 °C. After 24 h, the mixture was concentrated. The residue was purified by column chromatography (SiO2, 5% MeOH in CHCl3) to give 12 (0.12 g, 0.24 mmol) in 81% yield: 1H NMR (400 MHz, DMSO-d6) δ 0.96-1.02 (m, 6H), 1.32-1.49 (m, 4H), 1.64-1.77 (m, 4H), 3.44-3.52 (m, 2H), 3.58-3.62 (t, J = 7.3, 2H), 3.74-3.78 (t, J = 7.8, 2H), 3.97-4.02 (m, 1H), 4.17-4.30, 4.54-4.58 (m, 2H), 4.90-4.93 (t, J = 6.0, 1H), 5.15-5.16 (d, J = 5.5, 1H), 7.53-7.55 (dd, J = 5.5 and 1.8, 3H), 8.62 (s, 1H), 8.63-8.64 (d, J = 4.2, 2H), 9.01 (s, 1H) , 9.21 (s, 1H); 13C NMR (100 MHz, DMSO-d6) δ 14.1, 14.3, 19.7, 20.3, 29.4, 31.0, 45.7, 47.0, 51.9, 64.3, 69.9, 111.8, 124.7, 128.9, 131.0, 135.0, 139.1, 151.3, 152.9, 157.0, 157.8, 161.4, 168.2; HRMS (DART) calcd for C26H33N7O2 (M+H+): 476.27740, found; 476.27936.
(S)-8-[(Di-n-butylamino)methyleneamino]-3-[3-(4,4′-dimethoxytrityloxy)-2-hydroxypropyl]-6-phen-ylimidazo[4′,5′:5,6]pyrido[2,3-d]pyrimidine (13). A solution of 12 (0.40 g, 0.84 mmol) and DMTrCl (0.43 g, 1.30 mmol) in pyridine (6 mL) was stirred at room temperature. After 2 h, the mixture was partitioned between EtOAc and H2O. The organic layer was washed with aqueous NaHCO3 (saturated) and brine, dried (Na2SO4), and concentrated. The residue was purified by column chromatography (SiO2, 33% EtOAc in hexane) to give 13 (0.47 g, 0.60 mmol) in 71% yield: 1H NMR (400 MHz, DMSO-d6) δ 0.97-1.00 (m, 6H), 1.33-1.50 (m, 4H), 1.64-1.80 (m, 4H), 2.95-3.08 (m, 2H), 3.60-3.64, 3.76-3.79 (t, J = 7.3, 4H), 3.68 (s, 6H), 4.20-4.24 (dd, J = 14.2 and 7.3, 1H), 4.34-4.39, 4.51-4.55 (dd, J = 14.2 and 4.1, 2H), 5.42-5.44 (d, J = 6.0, 1H), 6.81-7.42 (m, 13H), 7.52-7.53 (m, 3H), 8.58 (s, 1H), 8.62-8.65 (q, J = 6.6 Hz, 2H), 8.98 (s, 1H) , 9.21 (s, 1H); 13C NMR (151 MHz, DMSO-d6) δ 14.1, 14.3, 19.7, 20.3, 29.3, 31.0, 45.7, 47.3, 51.9, 55.5, 66.2, 68.3, 79.7, 86.0, 112.0, 113.6, 124.8, 127.1, 128.3, 128.9, 128.9, 130.3, 130.3, 131.0, 135.0, 136.1, 136.1, 139.1, 145.4, 151.2, 153.0, 157.2, 157.8, 158.5, 161.4, 168.4. Anal. Calcd for C47H51N7O4·3/2H2O: C, 70.13; H, 6.76; N, 12.18. Found: C, 69.95; H, 6.36; N, 11.85.
(S)-8-[(Di-n-butylamino)methyleneamino]-3-[3-(4,4′-dimethoxytrityloxy)-2-[[(2-cyanoethoxy)(N,N–diisopropylamino)phosphanyl]oxy]propyl]-6-phenylimidazo[4′,5′:5,6]pyrido[2,3-d]pyrimidine (14). A solution of 13 (0.37 g, 0.48 mmol), N,N-diisopropylethylamine (0.41 mL, 0.95 mmol), and chloro(2-cyanoethoxy)(N,N-diisopropylamino)phosphine (0.21 mL, 1.48 mmol) in THF (3 mL) was stirred at room temperature. After 30 min, the mixture was partitioned between CHCl3 and H2O. The organic layer was washed with aqueous NaHCO3 (saturated) and brine, dried (Na2SO4), and concentrated. The residue was purified by column chromatography (SiO2, 50% EtOAc in hexane) to give 14 (0.43 g, 0.43 mmol) in 91% yield: 31P-NMR (162 MHz, DMSO-d6) δ 149.0, 149.9.
Oligonucleotide Synthesis. The synthesis was carried out with a DNA/RNA synthesizer by the phosphoramidite method. In the case of the coupling of the amidite 14, a 0.15 M solution of the amidite 14 in MeCN was used. Deprotection of the bases and phosphates was performed in concentrated NH4OH at 55 °C for 16 h. The oligonucleotides were purified by 20% PAGE containing 7 M urea to give the highly purified oligonucleotides, BA (26), BG (38), BC (43), BT (41), AB (36), GB (30), CB (34), and TB (33). The yields are indicated in parentheses as OD units at 260 nm starting from 1.0 µmol scale.
MALDI-TOF/MS Analyses of Oligonucleotides. Spectra were obtained with a SHIMADZU AXIMA-CFR plus time-of-flight mass spectrometer equipped with a nitrogen laser (337 nm, 3-ns pulse). BA m/z = 5919.2 ([M – H]–, calcd 5919.9; C194H237N75O109P18); BG m/z = 5931.0 ([M – H]–, calcd 5935.9; C194H237N75O110P18); BC m/z = 5896.3 ([M – H]–, calcd 5895.9; C193H237N73O110P18); BT m/z = 5906.6 ([M – H]–, calcd 5910.9; C194H238N72O111P18); AB m/z = 5914.9 ([M – H]–, calcd 5919.9; C194H237N75O109P18); GB m/z = 5932.4 ([M – H]–, calcd 5935.9; C194H237N75O110P18); CB m/z = 5892.1 ([M – H]–, calcd 5895.9; C193H237N73O110P18); TB m/z = 5907.6 ([M – H]–, calcd 5910.9; C194H238N72O111P18).
Fluorescence Experiments. Steady-state fluorescence emission spectra (370–670 nm) were obtained on a SHIMADZU RF-5300PC spectrofluorophotometer in quartz cuvettes with a path length of 1.0 cm and a 30 μM B concentration in an appropriate solvent or a 3.0 μM duplex concentration in a buffer comprising 10 mM sodium phosphate (pH 7.0) and 0.1 M NaCl at 20 °C. Spectra were recorded with use of excitation slit of 1.5 nm and emission slit of 1.5 nm for B or excitation slit of 3.0 nm and emission slit of 3.0 nm for the duplexes. The fluorescence quantum yield (Φem) was determined by use of quinine as a reference with the known Φem value of 0.58 (22 °C) in 0.1 M H2SO4. The quantum yield was calculated according to the following equation: Φem(S)/Φem(R) = (I(S)/I(R))×(A(S)/A(R))×(n(S)2/n(R)2). Here, Φem(S) and Φem(R) are the fluorescence quantum yields of the sample and the reference, respectively, I(S) and I(R) are the integrated fluorescence intensities of the sample and the reference, respectively, A(S) and A(R) are the respective optical density of the sample and the reference solutions at the wavelength of excitation, and n(S)2 and n(R)2 are the values of the refractive index for the respective solvents.
ACKNOWLEDGEMENTS
This research was partially supported by the Ministry of Education, Culture, Sports, Science, and Technology of Japan (Grant-in-Aid for Exploratory Research, 24659045) and by a C19 Kiyomi Yoshizaki research grant.
References
1. S. Kim and A. Misra, Annu. Rev. Biomed. Eng., 2007, 9, 289; CrossRef R. Nielsen, J. S. Paul, A. Albrechtsen, and Y. S. Song, Nature Rev. Genet., 2011, 12, 443; CrossRef N. R. Wray, J. Yang, B. J. Hayes, A. L. Price, M. E. Goddard, and P. M. Visscher, Nature Rev. Genet., 2013, 14, 507. CrossRef
2. A. Okamoto, K. Tanaka, and I. Saito, J. Am. Chem. Soc., 2003, 125, 4972; CrossRef O. Köhler and O. Seitz, Chem. Commun., 2003, 2938. CrossRef
3. K. Furukawa, M. Hattori, T. Ohki, Y. Kitamura, Y. Kitade, and Y. Ueno, Bioorg. Med. Chem., 2012, 20, 16; CrossRef M. Harrori, T. Ohki, E. Yanase, and Y. Ueno, Bioorg. Med. Chem. Lett., 2012, 22, 253. CrossRef
4. P. A. Harris and W. Pendergast, J. Heterocycl. Chem., 1996, 33, 319; CrossRef A. T. Krueger and E. T. Kool, J. Am. Chem. Soc., 2008, 130, 3989. CrossRef
5. R. Marumoto, Y. Yoshioka, O. Miyashita, S. Shima, K. Imai, K. Kawazoe, and M. Honjo, Chem. Pharm. Bull., 1975, 23, 759; CrossRef Y. Sato, T. Maruyama, and M. Honjo, Chem. Pharm. Bull., 1989, 37, 1604. CrossRef
6. A. E. Rettie, L. C. Wienkers, F. J. Gonzalez, W. F. Trager, and K. R. Korzekwa, Pharmacogenetics, 1994, 4, 39; CrossRef T. H. Sullivan-Klose, B. I. Ghanayem, D. A. Bell, Z.-Y. Zhang, L. S. Kaminsky, G. M. Shenfield, J. O. Miners, D. J. Birkett, and J. A. Goldstein, Pharmacogenetics, 1996, 6, 341. CrossRef